Understanding the Role of 3D Structures in Science
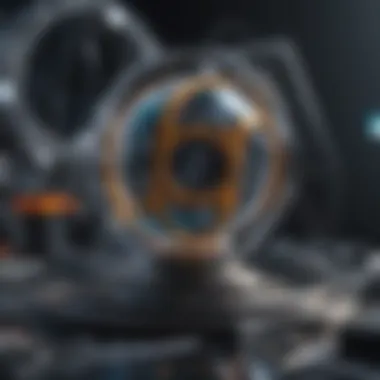
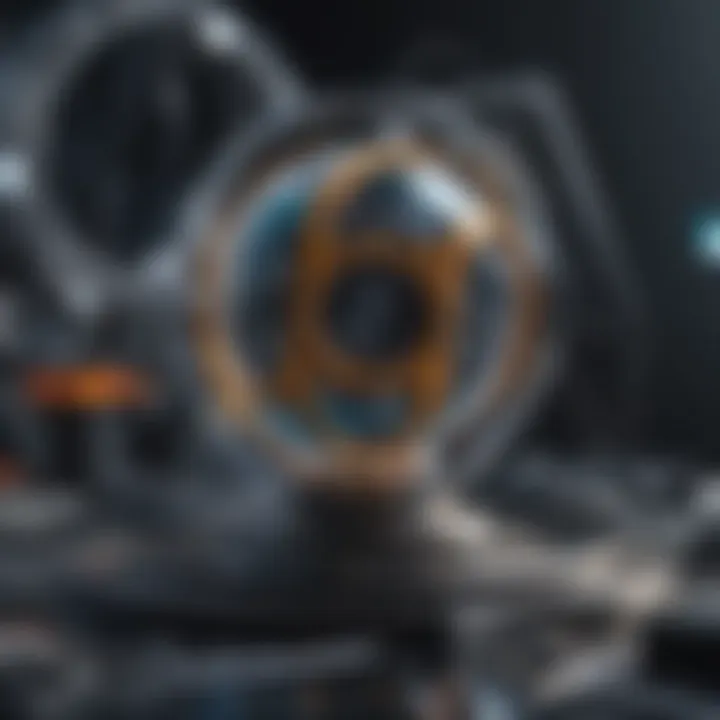
Intro
Understanding three-dimensional structures is crucial in various scientific fields. These structures are not just physical forms; they represent complex interactions, relationships, and phenomena in biology, chemistry, physics, and earth sciences. The following sections will explore the methodologies that scientists use to analyze 3D structures, their significance in advancing research, and how they connect various disciplines to drive innovation.
Research Overview
Methodological Approaches
In scientific research, several methodologies exist to model and analyze three-dimensional structures. Advanced imaging techniques, such as X-ray crystallography and nuclear magnetic resonance (NMR) spectroscopy, allow scientists to visualize molecular structures at atomic resolution. Moreover, computer-aided design (CAD) software and molecular dynamics simulations provide valuable insights into the behavior of molecules in a three-dimensional space.
These methodologies help clarify the spatial arrangement of structures, allowing researchers to derive functional information. For instance, understanding the 3D structure of proteins assists in drug design, as it reveals potential binding sites for therapeutic compounds. Methods like cryo-electron microscopy and tomography offer further opportunities for researchers to examine large biological complexes in their native states.
Significance and Implications
The implications of studying 3D structures are profound. These structures serve as a foundation for innovations in multiple areas, including materials science, drug development, and environmental monitoring. For example, knowing the 3D structure of a protein can direct the design of new pharmaceuticals, aiding in treatment for diseases like cancer or Alzheimer's.
Furthermore, when scientists grasp how elements interact within their 3D environments, they can create better predictive models for global phenomena, like climate change and ecological dynamics. Thus, 3D analysis not only enhances theoretical understanding but also enables tangible advancements in technology and healthcare.
"Three-dimensional structures are more than shapes; they are essential to understanding the dynamics of the natural world."
Current Trends in Science
Innovative Techniques and Tools
Having advanced tools is pivotal in modern research. Recently, artificial intelligence has emerged as a game changer in 3D structural analysis. AI algorithms can process vast datasets, identifying patterns and predictings interactions that traditional methods might miss. This supports researchers in making informed decisions quickly, crucial for time-sensitive research.
In addition, techniques like single-particle tracking provide deeper insights into molecular movements within live cells, allowing scientists to observe the dynamics of structures in real time.
Interdisciplinary Connections
The investigation of 3D structures fosters collaborations across different scientific disciplines. For instance, biochemists work closely with physicists and computer scientists to improve modeling techniques. Such collaborations enhance the understanding of complex systems, bridging gaps between theoretical knowledge and practical application.
As researchers continue to explore these intersections, they uncover new insights that further drive innovation and discovery across various fields.
Intro to 3D Structures
The realm of three-dimensional structures plays a pivotal role in advancing scientific understanding across various disciplines. The significance of 3D structures lies not only in their representation of physical forms but also in their ability to encapsulate complex interactions and behaviors. In scientific research, the representation of data and concepts in three dimensions can illuminate aspects that two-dimensional views cannot convey.
Definition and Importance
3D structures can be defined as representations of objects or systems that possess depth, width, and height. This three-dimensionality allows researchers to interpret spatial relationships more accurately. Whether in biology, where the complexity of protein folding is illustrated, or in geology, where rock formations depict historical processes, these structures provide critical insights.
The importance of 3D structures is magnified when considering their applications. They aid in the visualization of structures, enhancing communication among scientists. This clarity is equally crucial in educational contexts, where students can better grasp intricate concepts. Furthermore, the study of 3D structures facilitates innovations in various fields, particularly in drug development and materials science.
Historical Context
The journey of understanding 3D structures has its roots deeply embedded in scientific history. Early scientists primarily relied on two-dimensional representations such as drawings and graphs. With the advent of technologies such as X-ray crystallography in the mid-20th century, researchers began to uncover the hidden three-dimensional forms of biological macromolecules. The elucidation of the double helix structure of DNA by James Watson and Francis Crick in 1953 marked a significant milestone.
Over time, advancements in computational methods have propelled the exploration of 3D structures. Software such as PyMOL and Chimera have allowed scientists to visualize and manipulate molecular structures with unprecedented accuracy. The introduction of 3D printing technology further underscores the evolution of 3D structures, enabling the physical realization of complex models. Today, interdisciplinary approaches examining these structures are critical for ongoing scientific discourse and discovery.
3D Structures in Biology
3D structures play a crucial role in biology, serving as the foundation for understanding various biological processes. These structures are not just simple arrangements; they hold the keys to comprehending how life functions at multiple levels. The significance of 3D structures in biological research cannot be overstated, as they enhance the understanding of molecular interactions, cellular dynamics, and tissue organization.
Molecular Biology: Protein Folding
Protein folding is one of the most significant aspects of molecular biology influenced by 3D structures. Proper protein folding is essential for function. Misfolding can lead to diseases such as Alzheimer's or cystic fibrosis. In recent years, advancements in techniques like X-ray crystallography and nuclear magnetic resonance (NMR) spectroscopy have allowed scientists to visualize protein structures in three dimensions. This visualization helps in understanding how proteins interact with other molecules, further aiding drug design and therapeutic interventions.
The relationship between a protein's structure and its function is well documented, highlighting the necessity to study these conformations in detail.
Cellular Architecture
Cellular architecture refers to the arrangement of various cellular components. It is characterized by complex 3D configurations that define cellular functions. The understanding of cellular architecture allows researchers to uncover how cells communicate and coordinate activities. Techniques such as advanced imaging methods, including confocal microscopy, have revolutionized our understanding of cellular structures by providing detailed 3D reconstructions of cells. These images not only clarify the organization of cellular parts but also hint at their interactions, paving the way for discoveries in cellular biology.
Tissue Structures and Functionality
The 3D organization of tissues is imperative for their functionality. Tissues consist of various cell types that interact within a spatial framework that affects their behavior and function. For example, in connective tissue, the arrangement of collagen fibers provides strength and flexibility. The use of 3D bioprinting is showing promising results in replicating tissue structures for research and medical applications. Understanding how 3D structures relate to tissue functionality leads to insights into how to tackle issues like tissue degeneration and injuries, guiding regenerative medicine efforts and the development of biomaterials.
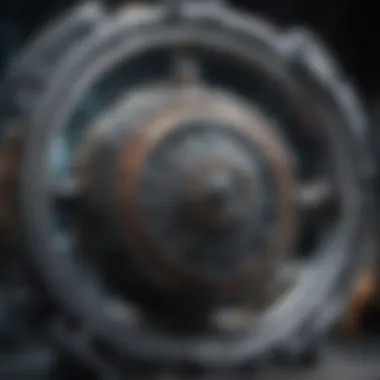
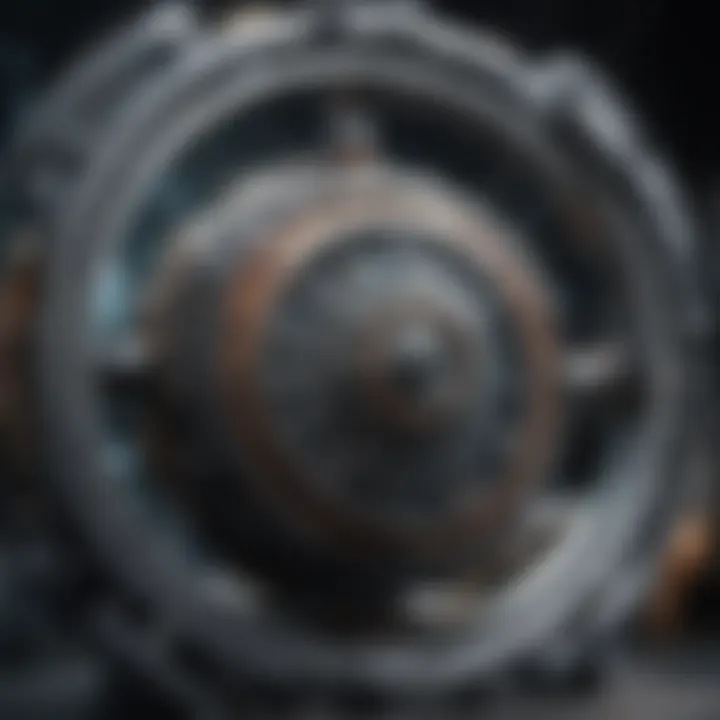
In summary, studying 3D structures in biology reveals crucial information about the functions of molecules, cells, and tissues. As technology advances, the tools available to investigate these structures become more sophisticated, enhancing our knowledge and opening avenues for innovation in healthcare and biotechnology.
3D Structures in Chemistry
The study of three-dimensional (3D) structures in chemistry is essential to understanding the nature of matter. These structures are crucial for explaining how substances behave and interact at the molecular level. The geometric arrangement of atoms within molecules directly influences chemical reactions, stability, and properties of materials. Recognizing the significance of these structures allows researchers to manipulate and predict chemical behavior, which has enormous implications in various applications.
Molecular Geometry
Molecular geometry deals with the shape and spatial arrangement of atoms in a molecule. Understanding this geometry provides insights into the chemical behavior of different substances. For instance, molecules can be linear, bent, trigonal planar, or tetrahedral based on the number of bonding pairs and lone pairs of electrons.
The predictive power of molecular geometry stems from theories such as VSEPR (Valence Shell Electron Pair Repulsion). This theory allows scientists to visualize the 3D arrangement of atoms based on the repulsion between electron pairs. By analyzing molecular geometry, researchers can:
- Identify reactivity patterns. Molecules with similar shapes often show similar reactivity.
- Understand polarity. The shape of a molecule influences its dipole moment, affecting its solubility and interaction with other substances.
- Design new compounds. By knowing how different configurations affect properties, chemists can engineer molecules for specific purposes.
In essence, mastering molecular geometry is fundamental in chemistry. It lays the groundwork for insights into how materials can be synthesized and utilized effectively.
Crystalline Structures
Crystalline structures refer to the ordered arrangement of atoms or molecules in a crystal lattice. This structure is vital for understanding the properties of solid materials. Crystals exhibit unique characteristics, such as distinct melting points, optical properties, and conductivity. The arrangement of particles within a crystal lattice determines its overall properties and behavior.
Some key points about crystalline structures include:
- Types of Crystals: Crystalline materials can be ionic, covalent, metallic, or molecular, each exhibiting different properties. For example, ionic crystals like sodium chloride (table salt) are hard and brittle, while metallic crystals like gold are ductile and conductive.
- Applications in Industry: Crystalline structures play a crucial role in fields like materials science and nanotechnology. Innovations in these areas are often dependent on manipulating crystal properties to create new materials with enhanced features.
- Characterization Techniques: Understanding and analyzing crystalline structures often involve methods such as X-ray diffraction, which reveals the arrangement of atoms within the crystal. This information assists in research and development within various branches of chemistry.
"The arrangement of particles in crystalline structures not only helps to determine their physical properties but is also pivotal in advancing material sciences."
In summary, the study of 3D structures in chemistry extends beyond academic curiosity. It forms the backbone of our understanding of chemical interactions and material development, further aiding innovation across disciplines.
3D Structures in Physics
The realm of physics encompasses numerous complex phenomena that rely heavily on the understanding of three-dimensional (3D) structures. These structures are essential for grasping concepts ranging from fundamental forces to intricate behaviors in materials. Utilizing 3D models enables physicists to visualize problems in ways that 2D representations cannot adequately convey. The benefits of integrating 3D structures into physics include enhancing predictive accuracy and enabling simulations of physical systems under various conditions. Furthermore, this approach aids in the comprehension of dimensional interdependencies that govern physical laws.
Geometric Constructs
Geometric constructs play a vital role in physical theories. They form the backbone for mathematical descriptions of physical phenomena. For instance, the concept of spacetime in relativity is an extrapolation of geometric ideas into higher dimensions. Understanding how objects interact in three-dimensional space allows for more in-depth analyses of motion and forces.
- 3D models help in visualizing particles and their trajectories.
- They clarify the relationships between points, lines, and solids in the context of force fields.
- Geometric constructs provide a framework for calculating dynamics in a more intuitive manner.
Moreover, geometric constructs are not limited to theoretical frameworks. Practical applications abound. For example, engineers use three-dimensional shapes to create blueprints for structures that must withstand various forces and conditions. This intersection of geometry and physics exemplifies how 3D structures enrich both theoretical and applied knowledge.
Vortex Models in Fluid Dynamics
In fluid dynamics, vortex models serve as a critical area of study. Vortices represent regions within fluid flows where rotational motion occurs, and understanding these can help predict behavior in numerous scenarios, from weather systems to aircraft design.
Vortex models illustrate how fluid motion can be simplified through 3D representations, providing insights into complex patterns that involve rotations and pressure changes.
- These models help analyze phenomena like turbulence, which can be computationally demanding when represented in 2D.
- They work in various fields, including aerodynamics and oceanography.
- Interactions between vortices in three-dimensional turbulence offer insights necessary for predicting energy transfer and flow patterns.
3D modeling of vortex behavior also allows for more realistic simulations, enabling researchers to predict and test the impacts of varied environmental factors on fluid behavior. This is particularly relevant in the context of climate modeling and engineering designs, where understanding complex fluid interactions is paramount.
In summary, 3D structures in physics reveal deeper insights into geometrical relationships and fluid motions. This understanding facilitates advancements in both theoretical explorations and practical applications, ultimately enhancing overall comprehension in the physical sciences.
3D Structures in Earth Sciences
3D structures play a crucial role in earth sciences, enabling researchers to visualize and analyze complex geological and environmental phenomena. Through the use of advanced modeling techniques, these structures offer insights into the processes that shape our planet. The importance of 3D representations lies in their ability to integrate multiple data sources, provide a comprehensive view of geological features, and facilitate informed decision-making regarding resource management and environmental protection.
Geological Formations
Geological formations are often complex and multifaceted. 3D models can represent these formations in a way that traditional methods cannot. This representation allows scientists to examine the spatial relationships between different layers of rock, sediment, and other materials. By understanding these relationships, geologists can better predict natural events such as landslides, earthquakes, and volcanic eruptions.
A few key benefits of using 3D models in geological studies include:
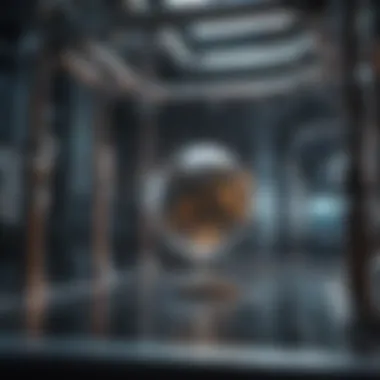
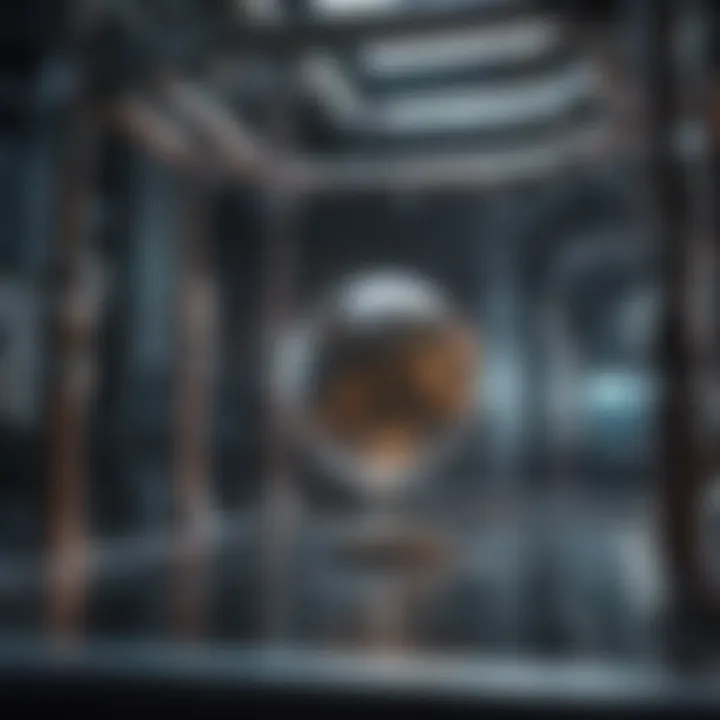
- Enhanced Visualization: 3D models provide a clear picture of geological structures, which may be hidden in 2D maps.
- Dynamic Simulations: Researchers can simulate various geological processes, like erosion or sedimentation, leading to a deeper understanding of their impacts.
- Resource Exploration: In fields such as mining and oil extraction, 3D modeling helps identify potential resources, minimizing exploratory drilling and maximizing efficiency.
“3D modeling allows earth scientists to transcend traditional boundaries, giving them the power to predict and explain natural phenomena effectively.”
3D Models for Climate Studies
In the realm of climate science, 3D structures are pivotal in understanding and predicting climate change impacts. With climate models becoming increasingly complex, 3D representations help scientists visualize interactions within the atmosphere, oceans, and land surfaces.
The application of 3D models in climate studies includes:
- Atmospheric Dynamics: Modeling the atmosphere in three dimensions aids in the study of weather patterns and climate phenomena, such as hurricanes and monsoons.
- Ocean Currents: Understanding the 3D flow of ocean currents is vital for climate predictions, particularly regarding heat distribution across the globe.
- Land Surface Interaction: Representing landforms in 3D assists in modeling how land use changes can influence local and global climates.
As a result, the integration of 3D structures into climate studies enhances predictive capabilities. This leads to better preparedness and adaptive strategies in response to climate change. 3D modeling brings important clarity to the complexities of our environment, making it an invaluable tool in earth sciences.
Technological Advances in Modeling 3D Structures
Technological advances in modeling 3D structures have dramatically transformed multiple fields of scientific research. These innovations not only enhance the understanding of complex phenomena, but they also bridge the gap between theory and practical application. Utilizing advanced technologies allows researchers to visualize intricate data and understand the underlying mechanics of various systems, facilitating insights that were previously unobtainable.
The integration of these technologies fosters better data representation, enhances accuracy, and provides a platform for predictive modeling. By refining the ability to create complex 3D models, researchers can explore the interactions within systems, simulate environmental changes, and study biological processes with greater fidelity than traditional methods.
Computational Techniques
Computational techniques are at the forefront of the advancements in 3D structure modeling. Through the use of algorithms and software, scientists can now generate intricate models that accurately represent various phenomena in three dimensions.
These techniques offer numerous benefits:
- Precision: Computational modeling can capture fine details that manual methods may overlook, ensuring an accurate representation.
- Speed: Advanced computing resources allow for rapid processing of large datasets, which is essential for real-time analysis.
- Versatility: Many software platforms can simulate a variety of systems, applicable in fields such as biology, chemistry, and geology.
Notable software tools include MATLAB, AutoCAD, and Blender, which enable users to create and manipulate 3D structures effectively. With increasing computational power, simulations also incorporate more variables, offering insights into how systems behave under different conditions.
3D Printing Applications
3D printing has emerged as a significant application of modeling 3D structures. It enables the tangible realization of digital models, making the conceptual come to life. This technology has various implications across several domains.
Some of the applications include:
- Prototyping: Researchers can rapidly produce prototypes of complex structures, facilitating quicker testing and modification.
- Customization: 3D printing allows for tailored solutions in fields like medicine, where custom implants or prosthetics can be designed according to patient-specific requirements.
- Materials Science: Innovative materials can be developed and tested readily, expanding the frontiers of engineering and technology.
As a result, 3D printing not only supports the visualization of models but also integrates the modeling process with physical experimentation, promoting a cycle of continuous improvement in research and innovation.
"The intersection of computational techniques and 3D printing signifies a new era in scientific modeling, where ideas can quickly transition from digital concepts to physical realities."
These technological advancements contribute significantly to the effectiveness of research and the potential to unravel the complexities of various systems. They pave the way for interdisciplinary collaboration and a deeper understanding of 3D structures, making it an essential focus in contemporary scientific endeavors.
Challenges in Understanding 3D Structures
Understanding 3D structures in various scientific fields poses significant challenges that can impact research and practical applications. This section delves into two main areas of concern: data complexity and interpretation challenges. Addressing these issues can enhance comprehension and drive advancements in scientific inquiry.
Data Complexity
The intricate nature of 3D structures results in data that is both vast and intricate. In biology, for example, a single protein can exhibit countless configurations and interactions. This complexity arises from the diverse atomic arrangements and the vast number of variables that can affect the shape and function. Additionally, high-resolution imaging techniques like X-ray crystallography and cryo-electron microscopy produce large datasets that require sophisticated computational methods for analysis.
The management of such complex data is crucial. Should researchers neglect data integrity, it can lead to misinterpretations of structural insights. Data complexity also introduces challenges in visualization. Standard representation methods may not effectively capture the details necessitated by thorough analysis. Thus, developing specialized software tools becomes essential. Modern computational techniques can assist in both managing and visualizing these complex datasets, making it necessary for researchers to adopt these tools for better understanding.
Interpretation Challenges
Once data has been analyzed, interpreting the 3D structures remains a formidable task. Misinterpretations can arise from various factors, including inadequate computational models and biases in the analysis process. Different scientific disciplines may apply varied approaches to structure interpretation, which can result in inconsistencies in findings. In multidisciplinary research, this could hinder effective communication among scientists.
Moreover, the ever-evolving nature of scientific knowledge implies that interpretations must be re-evaluated constantly. As new discoveries are made, previously accepted models may become obsolete or require modification. This fluidity adds another layer of challenge, as researchers strive to stay updated with current methodologies and insights.
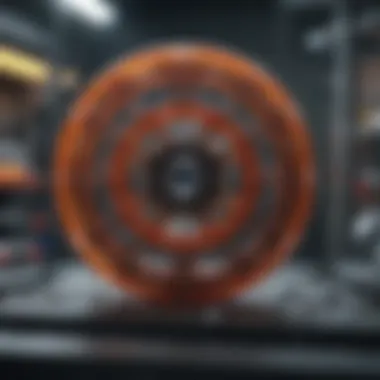
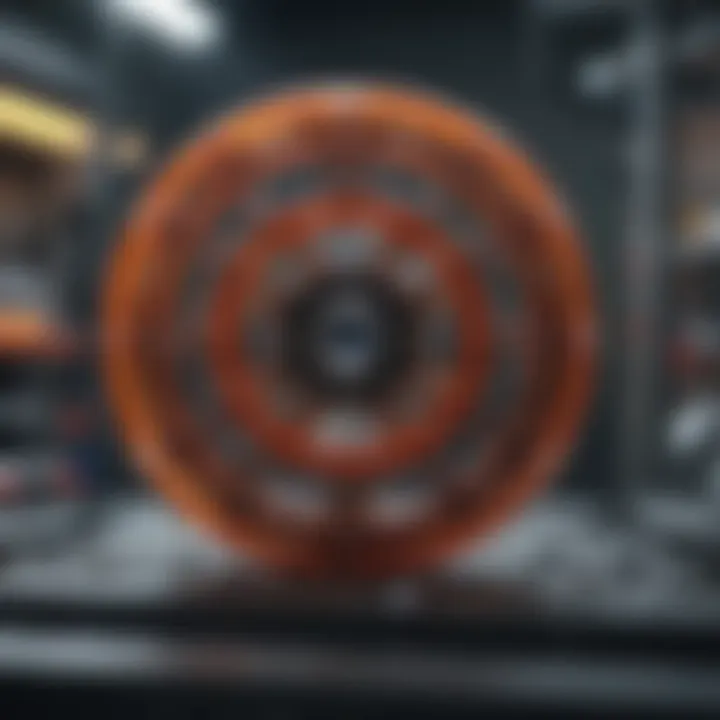
The nature of interpretive analysis in 3D structures signifies the need for continual learning and adaptation in scientific research.
Applications of 3D Structures in Research and Industry
The application of three-dimensional structures has enormous implications in both research and industry. These structures provide critical insights across multiple scientific disciplines. As scientists and engineers explore complex problems, the role of 3D modeling becomes more pronounced. Understanding three-dimensional configurations is vital for developing innovative solutions. By offering a visual context, these models enhance comprehension and communication among various stakeholders.
Drug Development
In the field of drug development, 3D structures of biological molecules significantly impact the design of new medications. For instance, when researchers analyze the structure of a target protein, they can determine how a potential drug might interact with it. This structural understanding aids in optimizing drug efficacy and minimizing side effects. By employing techniques like X-ray crystallography and nuclear magnetic resonance, scientists can visualize molecule interactions in detail.
- Molecular Docking: This process uses computational methods to predict the preferred orientation of a drug molecule when bound to its target. This is paramount in identifying new leads for drug candidates efficiently.
- Structure-Based Drug Design: This design approach relies on the knowledge of the three-dimensional structure of biological targets. It enables the rational design of compounds that can effectively bind and modulate the target's activity.
Through advancements in computational power and software, the integration of 3D structure analysis has accelerated the pace of drug discovery. Many pharmaceutical companies now employ these methodologies as standard practice in research pipelines.
Materials Science Innovations
In materials science, the design and analysis of 3D structures play a key role in developing new materials with desired properties. The understanding of how materials behave at the atomic or molecular level allows for the creation of innovative solutions that cater to modern challenges.
- Nanostructures: Researchers are exploring the use of nanomaterials to revolutionize industries like electronics and healthcare. The unique properties of materials at this scale often lead to unexpected applications and improvements.
- Additive Manufacturing: The rise of 3D printing technologies has transformed how materials and products are designed and produced. This method allows for the creation of complex geometries that were impossible with traditional manufacturing techniques. As a result, it has enabled customized and lightweight designs in various sectors, including aerospace and automotive.
Innovative collaborations between researchers in different disciplines fuel advancements in materials science. By combining expertise in chemistry, physics, and engineering, the study of 3D structures contributes valuable knowledge and breakthroughs.
"3D modeling is not just a tool, but a language that brings clarity to complex scientific ideas."
In summary, the applications of 3D structures in research and industry span numerous fields and offer profound insights. As the methodologies continue to evolve, especially with technology, the potential for groundbreaking discoveries remains vast. The roles of 3D structures in drug development and materials science exemplify the critical influence this area has in scientific progress.
Future Directions in 3D Structure Research
The exploration of future directions in 3D structure research presents crucial insights into how scientific inquiry can evolve. As researchers delve deeper into the complexities of three-dimensional frameworks, the significance of understanding these structures becomes ever more pronounced. Advancements in technology and interdisciplinary collaboration will likely yield innovative approaches. This section emphasizes emerging fields and interdisciplinary strategies, illustrating their potential impact on research and industry.
Emerging Fields of Study
As the realm of scientific exploration progresses, several emerging fields focus on the study of 3D structures. These fields blend diverse disciplines, creating a rich tapestry of knowledge and insights.
- Bioinformatics and Structural Biology: The intersection of biology and computer science has paved the way for new analytical techniques. These methods are crucial in predicting protein structures, enhancing our understanding of molecular interactions.
- Materials Science: This field investigates novel substances by utilizing 3D structural modeling. Here, researchers explore the properties of materials at a nanoscale level, leading to breakthroughs in nanotechnology and sustainable materials.
- Quantum Computing: Although still in its infancy, quantum computing holds the promise of vastly improving simulations of complex 3D structures. Its application could revolutionize fields such as drug design and materials engineering.
- Artificial Intelligence and Machine Learning: These technologies are being used to analyze vast datasets efficiently, allowing for patterns and structures to be identified that may have gone unnoticed previously. This can significantly advance the understanding of biological systems and materials.
The integration of these fields signifies a shift towards more cohesive and comprehensive explorations of 3D structures, ultimately benefiting scientific research.
Interdisciplinary Approaches
Interdisciplinary approaches represent another vital aspect of advancing 3D structure research. Collaboration among different scientific domains can unravel the complexities present in traditional studies.
- Integrative Biology and Chemistry: Understanding biochemical processes requires insights from both biology and chemistry. For instance, knowledge of enzymes and their 3D configurations is pivotal in developing effective drugs.
- Physics in Biological Contexts: Physics principles can elucidate phenomena such as molecular transport. Applying physical models to biological questions allows for more precise interpretations of cellular functions.
- Engineering and Computational Science: These two disciplines work closely to develop sophisticated modeling tools. These tools help visualize intricate 3D structures in real-time, enhancing our ability to manipulate and analyze data.
“Interdisciplinary collaboration is not merely beneficial; it is essential for innovative scientific discoveries.”
The future of 3D structure research is contingent on the flexibility and willingness of scientists to cross traditional boundaries. By embracing these emerging fields and interdisciplinary approaches, we can foster a richer understanding of complex three-dimensional frameworks, yielding significant advancements across various scientific areas.
Closure
The exploration of 3D structures is not merely an academic exercise. It represents a fundamental aspect of scientific inquiry across disciplines. By dissecting complex geometries, researchers can gain a nuanced understanding of various biological, chemical, physical, and earth science phenomena. This article has detailed the myriad influences that 3D structures exert on scientific research, from their role in protein folding to the interpretation of geological formations.
Summary of Insights
Throughout this article, we highlighted several key points that underlie the significance of 3D structures:
- Multidisciplinary Importance: Different fields benefit uniquely from 3D modeling. In molecular biology, understanding the spatial configuration of proteins is crucial for drug design. In earth sciences, 3D models can depict geological processes that are not easily conveyed in two dimensions.
- Technological Advances: As computational power increases, so does the sophistication of modeling techniques. 3D printing, for example, enables the practical application of theoretical structures, which can lead to tangible advancements in industries such as medicine and manufacturing.
- Challenges & Limitations: The complexity inherent in 3D data can be overwhelming. Among the discussed challenges are data interpretation difficulties and the need for interdisciplinary collaboration to overcome these barriers.
These insights indicate not only the relevance of 3D structures in current research but also their potential future applications.
Final Thoughts on the Future of 3D Structures
Looking ahead, the evolution of 3D modeling and its applications in scientific research appears promising. The integration of artificial intelligence into modeling software may soon enhance the accuracy and efficiency of creating complex 3D structures. Additionally, the trend towards interdisciplinary cooperation is likely to foster innovative solutions to existing problems.
Moreover, as society grapples with global challenges such as climate change and health crises, the importance of 3D structures will only increase. For example, more accurate 3D climatic models can lead to a better understanding of environmental changes, while advances in biomolecular architecture may yield breakthroughs in drug development.
Ultimately, understanding and utilizing 3D structures presents an opportunity to bridge gaps across scientific domains. Continued focus on this area of research will not only enhance our comprehension of the universe but may also lead to transformative applications that address critical societal needs.