Apex Agarose: Properties and Applications in Science

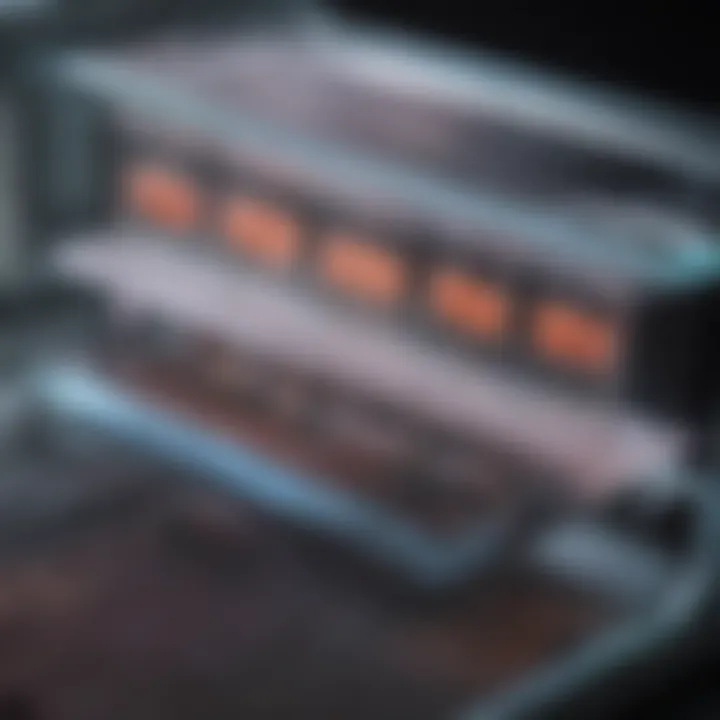
Intro
Agarose stands tall as a cornerstone in the world of molecular biology and biochemistry. It often finds its place in laboratories, bridging gaps between theory and practical applications. The substance, derived from agar, demonstrates remarkable physical and chemical properties, making it the go-to material for gel electrophoresis. When one speaks of apex agarose, it takes this already vital component a notch higher. This particular variant boasts enhanced purity and consistency, which translates into better performance during molecular separation tasks.
Understanding the properties of apex agarose goes beyond mere interest; it’s pivotal for anyone stepping into the life sciences arena. For students, educators, researchers, and professionals alike, grasping its intricacies may pave the way for innovative discoveries in DNA manipulation and protein analysis. As we delve deeper, we’ll explore how this agarose variant serves as a catalyst for advancements in various scientific endeavors.
Preface to Apex Agarose
Apex agarose is of high significance in various scientific disciplines, particularly in molecular biology and biochemistry. This section sets the stage for understanding its myriad properties and practical uses. With the rise of bioanalytical techniques, agarose gels have emerged as essential tools for the separation and analysis of biomolecules. Understanding the fundamentals of apex agarose is crucial, as it aids researchers and lab professionals in selecting appropriate methods for experimental procedures involving nucleic acids and proteins.
Definition and Background
Agarose itself is a polysaccharide that is derived from the cell walls of red algae, mainly from the genus Gelidium and Gracilaria. Apex agarose represents a specific refined variant of agarose, optimized for its properties, aiming to enhance its performance in laboratory applications. Its average molecular weight and gel-matrix porosity make apex agarose distinctly useful in gel electrophoresis. This product boasts lower levels of impurities compared to traditional agarose, resulting in clearer bands and greater resolution during analysis.
The application of apex agarose was born out of necessity for more specialized forms of gel that accommodate biological macromolecules like DNA and proteins. By utilizing this variant, one ensures that the gels provide optimal conditions for both practical use and reproducibility in experiments. The variations in gel concentration allow for adaptable separation, making it an indispensable material for researchers.
Historical Development
Agarose has a storied history, dating back to the mid-20th century. Initially, agarose was primarily used as a bacteriological medium. Researchers began noticing its potential in molecular biology during the 1970s when the methods for electrophoresis were refined. As scientists sought improved ways to analyze complex mixtures of biomolecules, they experimented with various concentrations of agarose.
With the advent of recombinant DNA technology and the rising demand for a highly authentic and reliable method of analyzing nucleic acids, the demand for specialized agarose expanded.
The introduction of apex agarose into the market marked a significant turn—the material offered better resolution, stability, and ease of handling. As the biological science landscape evolved, apex agarose adapted to meet the rigorous needs of protein analysis and nucleic acid separation, paving the way for vibrant discussions around innovations in gel preparation and use.
The exploration of its applications continues, allowing scientists to engage with apex agarose on different levels. By understanding its background, users not only grasp the logistical aspects but also appreciate the relevance of apex agarose within the broader study of life sciences.
Chemical Composition of Apex Agarose
Understanding the chemical composition of Apex Agarose is crucial for elucidating its role in scientific applications, particularly within molecular biology and biochemistry. The properties of agarose, stemming from its unique composition, greatly influence its functionality and suitability for diverse experimental techniques. Thus, this section will delve into two fundamental aspects: the source and extraction of agarose, followed by an examination of its molecular structure.
Source and Extraction
Agarose is primarily derived from red algae, specifically from species like Gelidium and Gracilaria. These algae are not only a renewable resource but also rich in polysaccharides, which are essential for producing high-purity agarose. The extraction process typically involves boiling the algae in water to dissolve the agar, then cooling this solution to allow the agarose to gel. This gel is further purified to enhance its qualities.
The extraction techniques wield a significant impact on the properties of agarose. It can yield a spectrum of agarose types, each varying in gel strength, clarity, and gelling temperature. Researchers often emphasize the importance of sourcing high-quality agarose to ensure reproducibility and reliability in their experiments.
Some notable methods for extraction may include:
- Hot water extraction: This method is effective for obtaining a high yield of agarose.
- Chemical extraction: Although less common due to potential impurities, it can enhance certain properties.
- Enzymatic extraction: Emerging as a more sustainable option, this technique proposes a green chemistry approach to extraction.
Molecular Structure
The molecular structure of agarose is largely responsible for its physical properties, making it particularly valuable for gel electrophoresis and other applications. Agarose is a linear polysaccharide made up of repeating units of agarobiose, which contains D-galactose and 3,6-anhydro-L-galactopyranose. This unique arrangement leads to the formation of a gel matrix with a porous structure when dissolved in water and subsequently cooled.
One can visualize agarose as a tangled network of long chains. When it gels, these chains create microscopic pores, permitting the separation of various biomolecules based on size.
Key points of its molecular structure include:
- Porosity: The size of the pores is inversely proportional to the concentration of the agarose gel. Higher concentrations yield smaller pores, allowing for finer separation of smaller DNA fragments.
- Hydrophilicity: Agarose has an affinity for water, which aids in forming gels that retain moisture, essential for maintaining sample integrity during electrophoresis.
- Thermal reversibility: Agarose gels can be melted and reformed without significant degradation, a trait that enhances its utility in numerous applications.
The molecular design of agarose not only underpins its fundamental properties but enhances its adaptability in research, providing a reliable medium for separating nucleic acids and proteins.
In essence, the chemical composition of Apex Agarose significantly shapes its applications in modern science. The sourcing, extraction, and structural characteristics are interwoven, contributing to a versatile compound tailored for the intricacies of laboratory work.
Physical Properties
Understanding the physical properties of apex agarose is essential for its application in modern science. These properties dictate how agarose behaves under various conditions, impacting its effectiveness in different experimental setups. From its melting and gelation points to porosity and thermal stability, each characteristic plays a critical role in determining how well agarose can perform in intricate techniques such as gel electrophoresis and molecular separations.
Melting and Gelation Points
The melting and gelation points of agarose are vital factors. Agarose is unique in that it becomes liquid when heated and solidifies upon cooling. The gelation point typically hovers around 35 to 40 degrees Celsius, making it a suitable medium for biological applications at room temperature. Important benefits include:
- Controlled environment: Precision in temperature management allows for reproducible gel formation, which is crucial in molecular analysis.
- Wide application range: The ability to solidify at slightly warmer temperatures extends its usability in various experiments, from DNA separation to protein investigations.
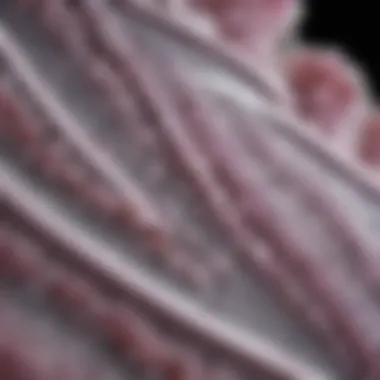
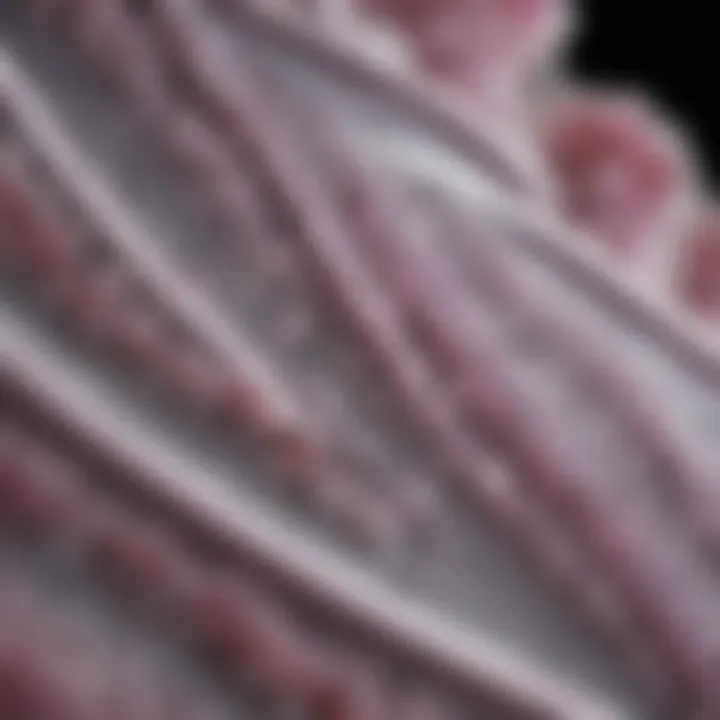
Researchers often compare these points with other gel systems. For instance, polyacrylamide has a higher melting point, influencing its application for different biological materials. Understanding these nuances sets the foundation for efficient experimentation and innovative technique development.
Porosity and Pore Size Distribution
Agarose gels exhibit varying porosity and pore size distributions, both of which are fundamentally important in molecular biology. The porosity dictates how easily molecules can navigate through the gel matrix during electrophoresis, affecting separation efficacy. Some critical considerations include:
- Separation of biomolecules: Larger pores allow for the migration of bigger DNA fragments, while smaller pores are better suited for analyzing smaller fragments.
- Customization: By adjusting agarose concentration, differing pore sizes can be achieved, allowing the researcher to tailor gels for specific tasks, enhancing both resolution and clarity of results.
This flexibility is a substantial advantage in comparative studies, where different molecular weights are analyzed. Agarose has been widely recognized for its ability to modulate pore size through concentration adjustments, an attribute that directly correlates to successful separation outcomes.
Thermal Stability
When it comes to thermal stability, apex agarose shines with notable resilience. The thermal behavior of agarose under various conditions is critical for its performance in the lab. Considerations of thermal stability include:
- Reliability during experiments: Agarose maintains its structure over a range of temperatures, which is vital during extended electrophoresis runs. This stability minimizes risks associated with gel degradation and loss of sample integrity.
- Impact on reproducibility: A stable gel ensures consistent results across experiments, allowing researchers to trust their findings and replicate them in future studies.
In summary, the physical properties of apex agarose, including melting and gelation points, porosity and pore size distribution, and thermal stability, form the backbone for its applications in molecular biology. Each attribute plays a noteworthy role, making agarose a cornerstone in biochemical studies today. Understanding these properties enriches the scientist's toolkit for tackling complex experimental challenges.
Applications in Molecular Biology
Apex agarose holds a significant place in molecular biology due to its versatile and practical applications. Its role extends beyond being just a gel; it serves as a foundational tool for various experimental procedures that drive research and diagnostics. When it comes to methodologies like gel electrophoresis, DNA separation, and protein analysis, apex agarose is often the go-to medium. This section elaborates on the specific applications and the unique attributes that make agarose a vital component in modern biological research.
Gel Electrophoresis Techniques
Principles of Gel Electrophoresis
Gel electrophoresis plays a crucial role in the separation of biomolecules based on size and charge. The basic principle involves applying an electric field to a gel matrix, allowing charged particles to migrate through the gel. Apex agarose gel is favored for this process due to its appropriate viscosity and the ease of preparing a gel with varying concentrations suitable for different applications. One key characteristic of gel electrophoresis is its high resolution, allowing the precise separation of nucleic acids and proteins.
A primary advantage of this technique is its ability to simultaneously analyze multiple samples, making it efficient in a researcher’s workflow. However, one downside is that large DNA fragments may not separate well if the agarose concentration is too high.
"The simplicity and versatility of gel electrophoresis techniques have transformed how scientists visualize and analyze complex mixtures of biomolecules."
Comparative Analysis of Agarose and Polyacrylamide Gels
In this analysis, agarose gel is often pitted against polyacrylamide gel, each having its advantages and drawbacks. While agarose is generally better for separating larger DNA fragments, polyacrylamide excels in resolving smaller proteins and nucleic acids. The unique feature of polyacrylamide gel is its ability to be tailored precisely for various specific applications, making it a well suited choice for high-resolution work, such as protein sequencing.
However, agarose excels in its ease of preparation and less toxic handling requirements compared to its polyacrylamide counterpart, which often involves more hazardous reagents. Here, the choice often depends on the specific application at hand, highlighting the need for a nuanced understanding of each gel’s capabilities.
Applications in DNA Separation
Optimization of Electrophoresis Conditions
Optimizing the electrophoresis conditions is crucial for the separation of DNA. This involves adjusting parameters such as voltage, buffer composition, and gel concentration based on the size and nature of the DNA fragments being analyzed. Apex agarose’s compatibility with various buffers and its tunable concentration enable researchers to achieve optimal separation in DNA analysis workflows.
One significant benefit of optimization is improving resolution, allowing researchers to distinguish between fragments that are only a few base pairs apart. On the flip side, less-than-ideal conditions can lead to smearing and poor separation, confounding results and leading to misinterpretations.
Visualization Techniques
Once DNA has been separated, visualization techniques come into play. Ethidium bromide, a commonly used intercalating agent, can stain the gel post-electrophoresis to enable visualization under UV light. 'Safer' alternatives, like SYBR Green, are being embraced for their lower mutagenic potential, thus representing a significant advancement in how researchers visualize results.
The unique aspect of these visualization techniques is that they allow for real-time monitoring during electrophoresis. The downside might be the need for specialized imaging equipment, which is not always accessible in all laboratories.
Protein Analysis Techniques
Western Blotting Protocols
Western blotting is instrumental in detecting specific proteins in a sample after they have been separated by gel electrophoresis. This method utilizes antibodies specific to the target protein, allowing researchers to quantify and visualize proteins of interest. One critical strength of using agarose gels in Western blotting is related to the minimal background noise due to the low binding properties of agarose, enhancing signal clarity.
However, the limitation lies in its ability to separate smaller proteins effectively, where polyacrylamide gels might perform better. Nevertheless, agarose retains its prominence in the field due to its practicality and ease of use.
Challenges in Protein Gel Electrophoresis
Despite its advantages, protein gel electrophoresis with agarose presents several challenges, including potential issues with protein denaturation. The integrity of proteins can be compromised if not run under optimal conditions. This phenomenon may lead to difficulties in analyzing post-translational modifications and complex protein structures, which are vital for in-depth studies.
Another constraint is the reproducibility of results; factors such as gel preparation errors or inconsistent sample loading may hinder clarity. Nevertheless, continued advancements in techniques, alongside a better understanding of gel properties, show promise for mitigating these challenges.
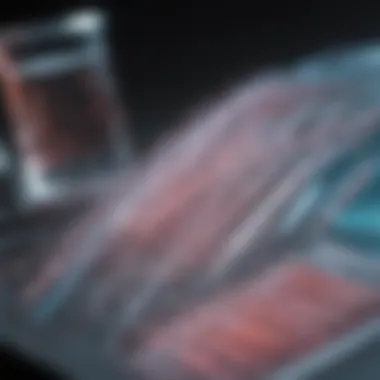
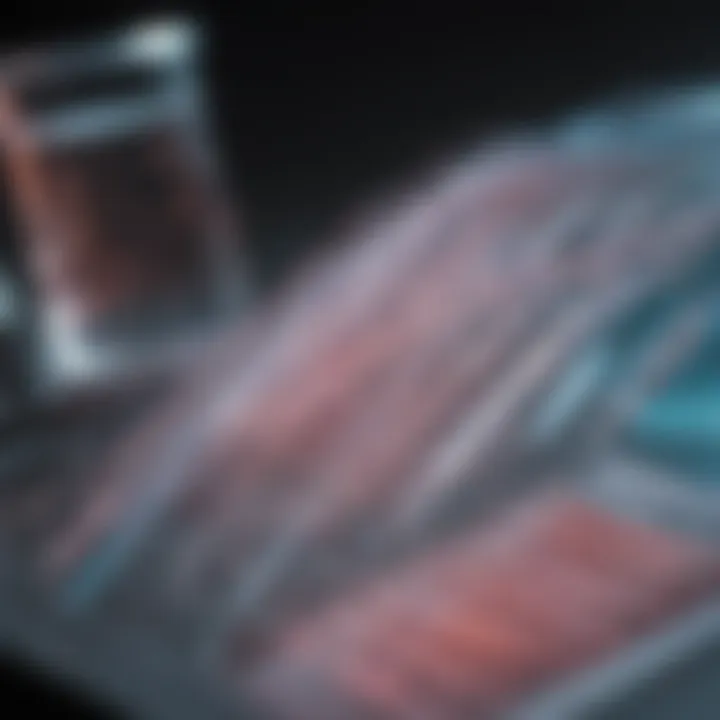
Emerging Trends in Agarose Research
The sphere of agarose research is evolving rapidly, opening doors to innovations that promise to reshape the landscape of molecular biology. Far from being a static field, advancements in agarose technology are enhancing its effectiveness, making it a cornerstone in various applications. Key trends to note include modified agarose variants and the integration of nanotechnology, both of which are driving the development of more efficient and adaptable gel systems. These changes bring with them significant implications for researchers, educators, and students alike.
Innovations in Agarose-Based Technologies
Modified Agarose Variants
Modified agarose variants have emerged as game changers in the gel electrophoresis arena. One primary aspect of these variants is their tailored chemical properties, designed to tackle specific experimental requirements. These modifications can enhance their electrophoretic resolution and allow for better separation of complex biomolecules. This flexibility makes modified agarose a noteworthy choice for applications that require higher precision, such as genomics and proteomics.
A significant characteristic of modified agarose is its ability to maintain structural integrity across diverse laboratory conditions. This quality means that researchers are less likely to encounter issues such as gel shrinkage or distortion during experiments. However, there are caveats; while these variants hold great potential, their cost can sometimes be a barrier for budget-constrained laboratories. Yet, considering their enhanced performance, many argue that the benefits far outweigh these downsides.
Integration with Nanotechnology
The integration of nanotechnology with agarose gels is ushering in a new frontier in research. This combination allows scientists to create smarter, more responsive gel systems that can react dynamically to varying conditions. For instance, by incorporating nanoparticles into agarose, researchers can manipulate gel properties, modifying its porosity and stability. This capability opens avenues for more versatile and efficient applications, especially in targeted drug delivery and biosensing.
Another interesting facet is the potential for nanotechnology to enhance image and signal sensitivity in diagnostic applications. With nanoparticles, the detection limits in various assays can improve significantly, resulting in quicker and more reliable diagnostic outcomes. However, such innovations must be approached carefully, as implications for biocompatibility are paramount.
High-Throughput Screening Applications
The rise of high-throughput screening has revolutionized the pace of research, and agarose gels play a crucial role in this movement. Researchers can employ agarose to quickly evaluate large libraries of samples, streamlining the process of compound screening for drug discovery. This efficiency not only saves time but also labor costs, allowing laboratories to allocate resources more effectively.
High-throughput techniques often rely on agarose gels as a platform for assays that can test multiple conditions simultaneously. This multiplexing capability is particularly useful in applications such as enzyme activity studies or cellular responses, where traditional methods may fall short. It enables scientists to rapidly pinpoint effective compounds from vast arrays, expediting the journey from the lab to real-world applications.
Impact on Diagnostic Techniques
Finally, the impact of agarose developments on diagnostic techniques cannot be understated. As the demand for rapid and accurate diagnostics grows, agarose gels are adapting to fulfill this need. Innovations in agarose technology often lead to enhanced sensitivity and specificity, which are critical in fields such as infectious disease testing and cancer detection. These improvements in diagnostic capabilities could mean the difference between effective treatment and missed opportunities for patient care.
"Harnessing the power of agarose in innovative ways paves the path forward for breakthroughs in scientific research."
As researchers dive deeper into the potential of modified agarose variants and incorporate advanced technologies like nanomaterials, the future of agarose applications appears promising. The continued exploration will further solidify agarose's role as an essential tool in modern scientific inquiry.
Comparative Analysis with Other Gel Systems
The comparative analysis of agarose with other gel systems is a crucial part of understanding its role in modern science, particularly in molecular biology and biochemistry. Gel systems, such as polyacrylamide and starch gels, have distinct properties that influence their applications. By diving into these comparisons, we can grasp the reasons why researchers select one over the other in different experimental contexts. This discussion not only sheds light on the individual characteristics of each type but also enables scientists to make informed choices that can affect the accuracy and efficiency of their work.
Agarose vs. Polyacrylamide Gel
Advantages and Disadvantages
When examining the advantages and disadvantages of agarose versus polyacrylamide gels, one can't overlook the pivotal role these two mediums play in electrophoresis. Agarose gels are often favored for their simplicity and ease of preparation. Their porous structure allows for the efficient separation of DNA fragments based on size, making them a go-to choice for genetic analysis.
Conversely, polyacrylamide gels offer much greater resolution for smaller molecules, such as proteins. This is particularly useful when fine-tuning the analysis in more complex samples. However, the preparation of polyacrylamide can be technically demanding and requires more safety precautions due to the handling of acrylamide, a neurotoxin.
The choice between the two largely depends on the specific requirements of the experiments being performed:
- Agarose:
- Polyacrylamide:
- Easy to handle
- Ideal for larger DNA fragments
- Higher gel strength makes it suitable for a variety of applications
- Superior resolution for small DNA or protein samples
- More sensitive to variations in preparation
- Requires careful handling due to toxicity
Use Cases
The use cases of agarose and polyacrylamide gels highlight their specialized applications. For instance, agarose is widely adopted when preparing for gel electrophoresis aimed at the separation of large DNA fragments. Thanks to its user-friendly nature, it is commonly used in undergraduate laboratories and even in some professional settings.
Polyacrylamide gels, on the other hand, dominate in the realm of protein analysis, particularly in techniques such as SDS-PAGE. Here, the gel composition can be fine-tuned to achieve the desired resolution for different proteins, lending to its utility in proteomics research.
Some unique factors to consider include:
- Agarose:
- Polyacrylamide:
- Primarily for genetic sequencing and analysis
- Often employed in pulsed-field gel electrophoresis for massive DNA molecules
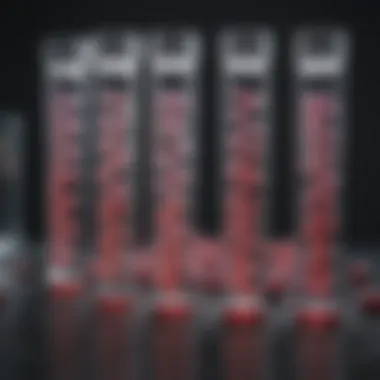
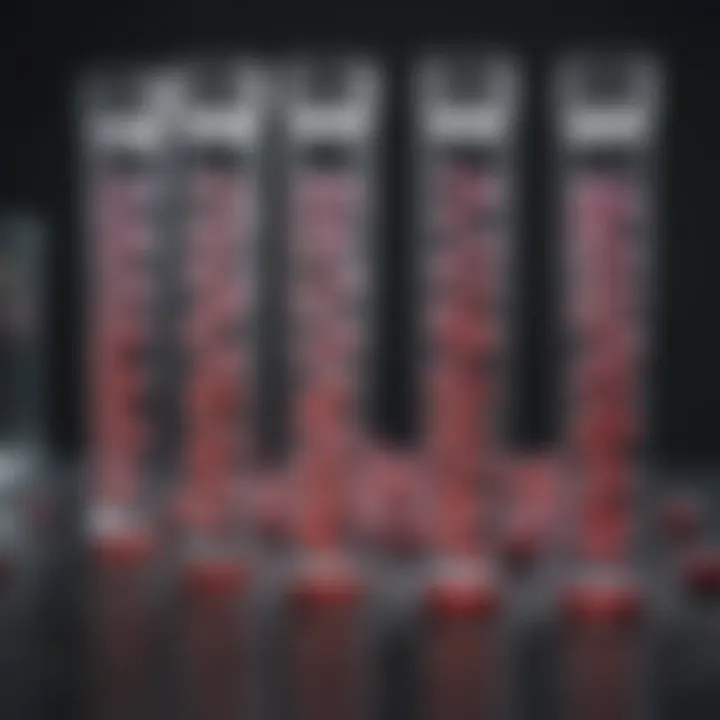
- Essential for Western blotting protocols
- Used in 2D gel electrophoresis for detailed protein profiling
Choosing the right gel for a specific task influences the outcome of experiments and could lead to substantial differences in data quality.
Agarose vs. Starch Gel
Agarose and starch gels also represent an interesting comparison within gel electrophoresis. While both serve similar roles, their properties differ markedly. Starch gels, traditionally used in biochemical assays, can demonstrate distinct migration patterns when subjected to electrophoresis, which sometimes yields lower resolution in comparison to agarose.
However, their simplicity in preparation makes starch gels attractive for quick analyses. They are particularly beneficial in diagnostic applications where immediate results are paramount. In contrast, agarose gels provide a more uniform surface, leading to consistent results across multiple tests.
In summary, the comparative analysis of agarose with other gel systems is crucial for students, educators, and researchers alike. Understanding the unique strengths and limitations of each gel type allows for better experimental design and outcomes in a wide variety of applications, from basic research to advanced diagnostics.
Limitations and Challenges
Exploring apex agarose in a laboratory setting reveals its significance as a versatile tool, yet it's vital to acknowledge its limitations and the challenges that come along with it. Understanding these weaknesses allows researchers to make informed decisions about selecting the right materials for their experiments. Various elements, benefits, and considerations around the limitations and challenges of apex agarose will be covered to deepen the understanding of its role in modern science.
Resolution Limitations
One of the primary issues associated with apex agarose is its resolution limitations. While agarose is celebrated for its ability to separate large biomolecules, it might not excel in distinguishing smaller fragments. For instance, when separating DNA fragments, agarose gels typically provide less resolution than polyacrylamide gels, especially for sequences below 500 base pairs. This can lead to ambiguity in results, particularly in crucial experiments where accuracy is paramount. Researchers often find themselves grappling with a conundrum — whether to choose the ease of agarose or the higher resolution that can be achieved with polyacrylamide.
Despite these challenges, agarose maintains its popularity due to the larger size of DNA fragments associated with many molecular biology applications. However, its limitations become apparent when precision is required.
Reproducibility Concerns
Another hurdle faced in the use of apex agarose pertains to reproducibility. In scientific practices, the ability to recreate results consistently is crucial. Unfortunately, variations in gel preparation, agarose concentration, and even environmental factors can lead to significant discrepancies in outcomes. Researchers might experience different migration patterns for the same sample under seemingly identical conditions. This inconsistency mars the reliability of experiments, as it becomes challenging to draw definitive conclusions.
Several factors can contribute to these reproducibility issues:
- Gel Concentration: A slight change in agarose concentration can drastically shift the pore size of the gel, thereby affecting how proteins and nucleic acids migrate.
- Buffer Conditions: The choice of buffer and its concentration also play an important role. Small fluctuations can yield differing results.
- Temperature and Time: Variations in gel casting or running times due to temperature or skill level can influence the final outcome.
In the realm of molecular biology, where precision is king, these challenges cannot be overlooked. The need for standardized protocols becomes evident, as only through rigorous adherence to methods can reproducibility be achieved.
"Understanding the limitations of apex agarose is not merely academic; it's a practical necessity to ensure the integrity of scientific findings."
Navigating these limitations does not erase the value of apex agarose but rather emphasizes the need to approach its use with careful consideration and an understanding of its capabilities and constraints.
The Future of Apex Agarose in Research
As science continues to advance, the significance of apex agarose in various fields becomes ever more profound. This section explores the potential pathways for agarose development and its role in innovative research practices. With its existing applications persisting in molecular biology and biochemistry, a closer look into future enhancements provides insight into how apex agarose can further transform laboratory practices.
Potential Developments
Future advancements in apex agarose are likely to focus on enhancing its properties to meet the increasingly demanding needs of scientific research. These developments could include:
- Modified agarose formulations: Researchers are experimenting with modifying the molecular structure of agarose to create variants that possess tailored properties, such as improved gel strength or altered pore size distributions.
- Smart gels: The concept of smart gels that respond to environmental changes remains an exciting prospect. These could be designed to react to specific pH levels or temperature variances, enabling precise control over gelation conditions during experiments.
- Biocompatible gel systems: As the field of regenerative medicine expands, future agarose applications may integrate biocompatibility features. This could involve developing agarose formulations that are more suitable for encapsulating live cells and facilitating their growth in vitro.
- Enhanced imaging capabilities: Investigating methods to improve visualization—like coupling agarose with fluorescent compounds—will also be crucial for enriching data analysis in applications.
Such advancements not only hold promise for expanding the functionality of apex agarose but can also pave the way for groundbreaking discoveries in various scientific domains.
Cross-Disciplinary Applications
The beauty of apex agarose lies in its versatility across numerous scientific disciplines. In the near future, a greater focus on interdisciplinary collaborations may emerge. Some cross-disciplinary applications worth noting include:
- Cancer research: The role of agarose in tumor studies can be expansive. Techniques may evolve to include models that allow visualization of cancer cell behaviors in various microenvironments, shedding light on treatment responses.
- Environmental science: Utilizing apex agarose in gel technologies for environmental samples can enhance the detection and analysis of contaminants by providing a better medium for sample processing.
- Food science: Innovations may extend into food safety, where agarose-based gels can be used for testing the presence of harmful bacteria or toxins in food products.
- Pharmaceutical development: Integrating agarose in drug delivery systems could transform how medications are administered, enhancing the targeting of therapeutic agents within the body.
The potential applications demonstrate how apex agarose could serve as a bridge between emerging fields, driving knowledge forward and fostering collaboration among researchers.
The future of apex agarose is not just about its properties; it's about its ability to adapt and contribute to solving real-world challenges across various domains.
The End
In wrapping up this detailed exploration of apex agarose, it's clear this gelling agent is much more than just a laboratory staple. Its significance in molecular biology and various scientific applications cannot be overstated. The discussion highlights not just its fundamental properties but also the innovative applications leveraging its unique characteristics.
Summary of Key Points
- Chemical Properties: Apex agarose stands out due to its exceptional compatibility with various biological samples, making it a prime choice for gel electrophoresis.
- Applications: The article delves into its significance in DNA separation and protein analysis techniques, showcasing how it facilitates crucial experiments in molecular biology.
- Research Trends: Innovations, such as agarose modifications and integration with nanotechnology, point to a promising future that will continue to shape research methodologies.
- Limitations: While advantageous, challenges such as resolution limits and reproducibility concerns remain. Understanding these limitations is vital for researchers as they design experiments.
Final Thoughts on Apex Agarose
Overall, apex agarose symbolizes the intersection of traditional practices with modern scientific demands. As new techniques emerge, the importance of optimizing agarose's use becomes clearer. The insights derived from understanding its properties allow researchers to navigate various challenges effectively. Decisions regarding its application can lead to breakthroughs not just in academic settings but also in clinical diagnostics and biotechnology workflows.
As we anticipate developments in the field, it's evident that apex agarose will continue to play a pivotal role in advancing scientific knowledge, reminding us that the foundations of molecular biology rest on exemplary materials like this one. The interconnectivity of disciplines should inspire further research, as the road ahead promises to be filled with exciting discoveries and transformative applications.