Exploring the Impact of Cas9 Nickase D10A in Genome Editing
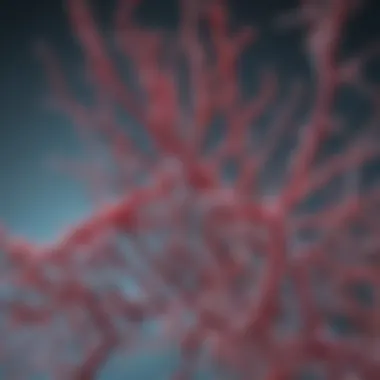
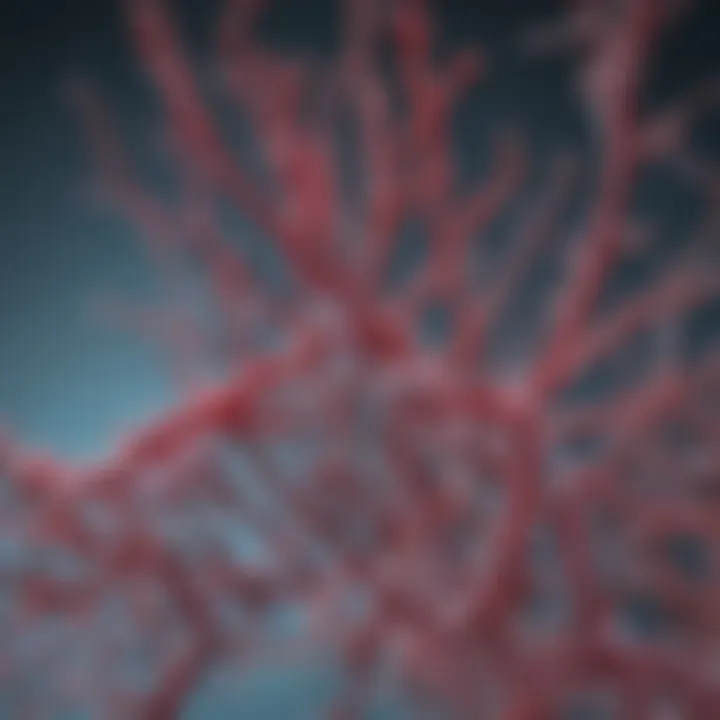
Intro
In recent years, advances in genome editing have reshaped the way scientists approach genetic modifications. Among the myriad of tools available, the Cas9 nickase D10A variant has demonstrated noteworthy promise. This article will explore the unique features and potential applications of Cas9 D10A, shedding light on its role in gene therapy and from a biological perspective, addressing its structure and action mechanism within cellular contexts.
Understanding Cas9 D10A, especially in comparison to traditional Cas9, is pivotal for researchers in the fields of genetics and molecular biology. The analysis not only highlights the enzyme's significance but also its implications for medical applications, particularly in treating genetic disorders.
Research Overview
Methodological Approaches
The exploration of Cas9 nickase D10A begins with methodological advancements that enhance its precision. Traditional CRISPR-Cas9 systems utilize a double-strand break mechanism, which, while effective, can lead to unintended mutations elsewhere in the genome. Alternatively, the D10A variant introduces a single-strand break, offering greater control and reduced off-target effects. Researchers have started employing this modification to improve gene editing accuracy.
Different methodologies are employed to assess the efficacy of Cas9 D10A, including:
- Cell Culture Studies: In vitro approaches to evaluate editing efficiency and specificity.
- Animal Models: Examination of therapeutic effects in genetically modified organisms.
- Bioinformatics Tools: Predictive analytics to anticipate potential off-target sites, enhancing target site selection.
Significance and Implications
The implications of using Cas9 D10A are far-reaching. Its precision not only minimizes genome disruption but also enhances the overall therapeutic potential of gene editing. This is particularly relevant in the context of gene therapy, where targeted interventions can correct genetic defects, paving the way for treatments of diseases like muscular dystrophy or cystic fibrosis.
Moreover, the innovations surrounding this work foster interdisciplinary collaboration between geneticists, bioengineers, and healthcare professionals. The result is a more holistic approach to genome editing that incorporates the latest in technology, ethics, and clinical practice.
"With the Cas9 nickase D10A, we are stepping towards a future where genetic disorders can be tackled in a more nuanced manner, reducing risk while maximizing impact in patient care."
Current Trends in Science
Innovative Techniques and Tools
Recent developments in the field have introduced various tools to optimize the use of Cas9 D10A in genome editing. For instance, researchers are leveraging advanced delivery systems, such as lipid nanoparticles, to efficiently introduce the editing components into target cells. These tools are designed with features that enhance uptake and reduce inflammatory responses.
The importance of continuous evolution in techniques cannot be overstated. As scientists uncover better methodologies for targeting, verifying, and delivering genetic edits, the impact of tools like Cas9 nickase D10A will only continue to grow.
Interdisciplinary Connections
The application of Cas9 D10A strains the boundaries between disciplines. Genetic engineering now engages fields such as computational biology for predictive analyses, ethical studies regarding genetic modifications, and even community engagement to ensure public understanding and acceptance. With every engagement from diverse fields, the conversation surrounding genome editing becomes richer and more informed.
The collaboration across disciplines not only enhances the understanding of Cas9 D10A's applications but also drives innovation in therapeutic strategies. As we look ahead, the synergy between these varying domains holds great potential for groundbreaking advancements in genetic research and therapy.
Foreword to Cas9 Nickase D10A
In genome editing, precision is the name of the game. The advent of CRISPR/Cas9 technology has transformed the field, and at the center of this revolution is the Cas9 enzyme. One variant, in particular, stands out due to its unique capabilities: the Cas9 Nickase D10A. This article aims to shine a light on this specific nickase, its significance, and how it offers a different approach compared to traditional Cas9.
The Cas9 Nickase D10A is not just another tool in the toolbox; it carries with it the potential to enhance gene editing techniques while minimizing collateral damage. Its ability to generate single-strand nicks rather than double-strand breaks reduces the chances of off-target effects, making it an attractive option for researchers working on gene therapy and other applications.
Being aware of the nooks and crannies of Cas9 Nickase D10A serves as a stepping stone for understanding its broader implications in genetic engineering. The following sections will delve into the functionalities and mechanisms of this variant, presenting a detailed exploration that is beneficial for students, educators, and professionals alike.
Understanding Cas9 and Its Importance
Cas9 is pivotal in genome editing primarily because it acts as a pair of molecular scissors. When it aligns with a specific target via guide RNA, it introduces cuts in the DNA at designated locations, thereby facilitating the modification of genes. Traditional Cas9 can induce double-strand breaks, which can lead to significant genomic alterations. This can sometimes lead to unwanted mutations, making it essential to approach gene editing with care.
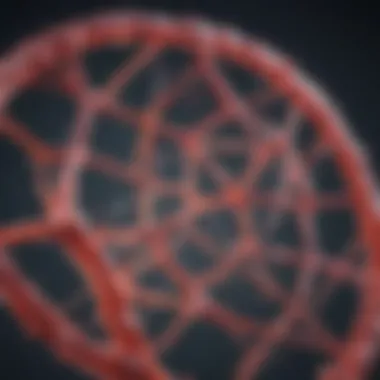
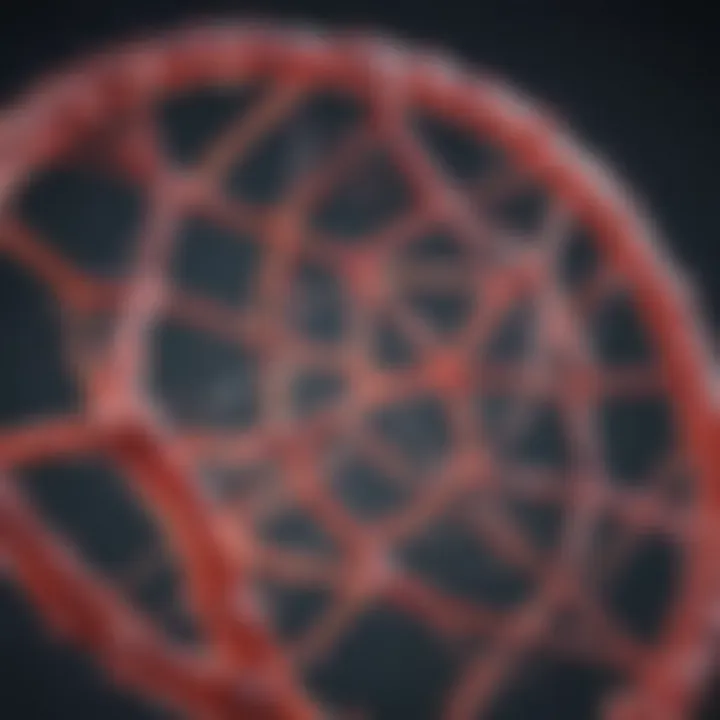
D10A differs fundamentally in its action. By only nipping one strand of the DNA helix, it offers a level of control that can mitigate the risks associated with double-strand breaks and enhance the efficiency of homology-directed repair. Moreover, as precision becomes increasingly prioritized in genomic modifications, understanding these nuances becomes crucial.
Defining Nickase Variants
Nickase variants like Cas9 D10A introduce a more delicate approach to genome editing. While the classical Cas9 is brilliant in its capacity to create double-strand breaks, the nickases refine this capability. They selectively create single-stranded breaks, or nicks, in the DNA. This targeted action opens avenues for various editing strategies and reduces the likelihood of unintended genetic consequences.
Several nickase variants have emerged, each with distinct characteristics. The appeal of D10A lies not only in its precision but also in its versatility. Different applications may require varying levels of alterations, and by employing a nickase, researchers can fine-tune their approach to gene disruption or correction. What sets D10A apart is its specific mutation at the D10 position, a change that delineates it from its conventional counterparts, thus offering a refined mechanism for DNA manipulation.
In summary, Cas9 Nickase D10A is a significant advancement in the realm of genome editing. By carrying out a delicate dance of precision, it effectively bridges the gap between innovation and safety.
"As genome editing continues to evolve, understanding the tools we have at our disposal is more important than ever. Cas9 Nickase D10A is a prime example of how we can proceed with caution while still pushing the boundaries of genetic engineering."
The upcoming sections will elaborate further on the structural biology of Cas9 Nickase D10A, exploring its protein structure and the key mutations that define its functionality.
Structural Biology of Cas9 Nickase D10A
Understanding the structural biology of Cas9 nickase D10A is paramount in illuminating its role in genome editing. The architecture of this enzyme is a crucial component that underpins its functionality and applications. By dissecting its structural elements, researchers gain insight into how alterations in its configuration can affect its gene-editing capabilities. This section aims to explore the protein structure and the significance of key mutations, shedding light on why these aspects are vital not just for academic inquiry but for practical applications in biotechnology.
Protein Structure and Domains
The Cas9 nickase D10A is an evolution of the traditional Cas9, primarily distinguished by a single amino acid mutation. Unlike its regular counterpart, which generates double-strand breaks, the D10A variant introduces a nick, or a single-strand break, in the DNA. This subtle shift in structure facilitates a more controlled method of gene editing.
At its core, the Cas9 enzyme comprises several conserved domains: the recognition lobe and the nuclease lobe.
- Recognition Lobe: This domain is essential for understanding how the enzyme interacts with the guide RNA and the target DNA sequence. It is responsible for binding to the guide RNA and helps position the enzyme appropriately for cleavage.
- Nuclease Lobe: Here lies the heart of Cas9's functionality. The nuclease lobe contains two critical catalytic domains—RuvC and HNH—that govern its cutting action. The D10A mutation affects the RuvC domain specifically, reducing the enzyme's capacity to create double-strand breaks, hence redefining its action to making nicks.
In essence, the structural intricacies of these domains inform how Cas9 D10A differentiates itself from traditional Cas9, leading to distinct applications in gene editing.
Key Mutations and Their Effects
The D10A mutation significantly alters the enzyme's functionality, shifting its specificity from double-strand breaks to single-strand nicks. This change is not merely academic; it brings tangible benefits for applications in gene therapy and precision editing among various fields.
A few noteworthy effects of this mutation include:
- Reduced Off-Target Activity: With less aggressive cutting action, Cas9 D10A provides a safer option that minimizes unintended modifications to the genome. This is particularly crucial in therapeutic applications where precision is paramount.
- Enhanced Homology-Directed Repair (HDR): The introduction of a nick can enhance the efficiency of HDR. Instead of triggering a non-homologous end joining (NHEJ) repair pathway, which can be error-prone, the single-strand break encourages the cells to utilize more accurate repair mechanisms, leading to precise gene editing.
- Functional Versatility: This variant can be paired with other editing techniques, like base editing, amplifying its utility in genetic modification strategies.
These distinctions highlight why the structural biology of Cas9 nickase D10A is crucial for its role in modern genome editing techniques. As research continues to advance, understanding these structural elements may lead to further refinements and applications that leverage the unique capabilities of D10A.
Mechanisms of Action
Understanding the mechanisms of action behind Cas9 nickase D10A is fundamental to grasping its role within genome editing. Cas9 D10A operates as a molecular scalpel, providing researchers with a powerful tool for modifying genetic material with precision. By focusing on the distinct nuances of how this variant alters DNA cleavage, we can appreciate its advantages in terms of specificity and efficiency compared to traditional Cas9.
How D10A Modifies DNA Cleavage
The D10A mutation in Cas9 converts the enzyme from a double-strand cutter to a nickase, which means it creates a single-strand nick in the DNA rather than a complete cut. This is pivotal for several reasons:
- Targeted Modifications: By only nicking one strand, D10A allows for more controlled edits. This is particularly useful when making subtle changes in the genome without introducing unintended alterations.
- Reduced Off-Target Effects: Nicking rather than cleaving both strands significantly lowers the likelihood of off-target effects. Research shows that when only one strand is modified, the risk of disrupting surrounding genes or regulatory regions is lesser. This precision is crucial when designing therapeutic applications to treat genetic disorders.
- Homology-Directed Repair: The resulting single-strand break activates the cell's DNA repair mechanisms, favoring homology-directed repair (HDR) when a donor template is supplied. This means that researchers can introduce new genetic material seamlessly and more accurately than with standard Cas9.
In essence, the modification from a double-strand cut to a single-strand nick reshapes how gene editing can be performed. As the science of genetics progresses, such modifications offer new avenues for exploration.
Comparison with Regular Cas9
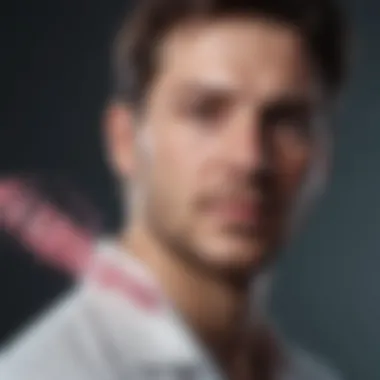
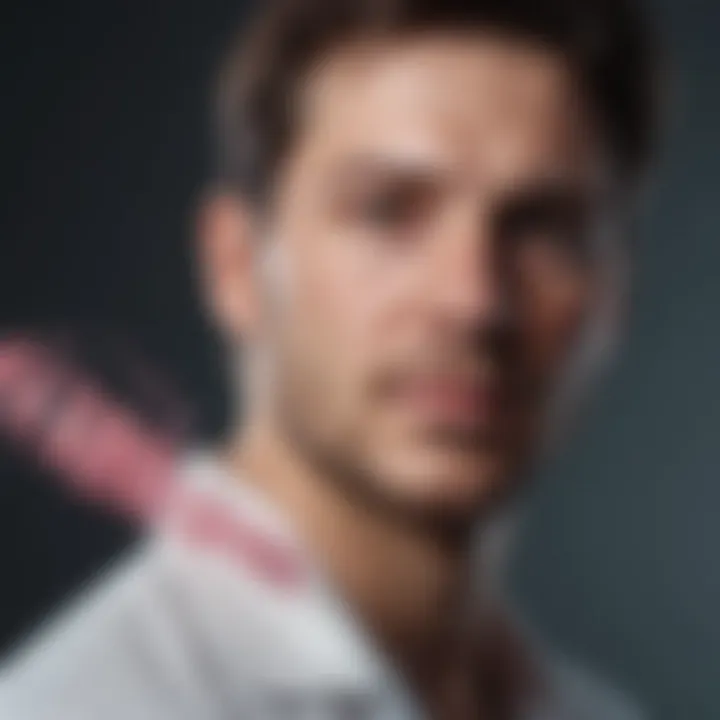
When comparing Cas9 D10A with traditional Cas9, it's clear that this specific variant holds its own advantages. Regular Cas9 is often celebrated for its effectiveness but comes with certain trade-offs that D10A helps to mitigate. Here are key considerations in direct comparison:
- Editing Precision: Regular Cas9 can result in double-stranded breaks, which, while effective, may lead to extensive unintended genomic alterations. In contrast, the higher precision offered by D10A allows for targeted interventions while minimizing collateral damage.
- Cellular Response: Following a double-strand break, cells often activate a pathway known as the non-homologous end joining (NHEJ), which can lead to insertions or deletions. This process can be problematic if an accurate edit is the goal. The D10A variant’s nicking method encourages accurate repair processes that are more favorable for specific outcomes.
- Applications in Therapeutics: For researchers focused on gene therapy, D10A's ability to perform edits without large-scale disruptions makes it a prime candidate for developing therapeutic strategies for genetic disorders. Its less invasive nature suits sensitive applications better than the traditional approach.
"The choice of Cas9 variant can profoundly impact the outcomes of genome editing, underscoring the importance of selecting the appropriate tool for each biological challenge."
Applications in Genome Editing
The diverse applications of Cas9 nickase D10A in genome editing mark it as a groundbreaking tool in modern genetics. Understanding these applications is crucial, as they underscore the potential of this nickase variant in enhancing precision and efficacy in genetic modifications. The real magic lies in how D10A modifies traditional genome editing methods, making it not just a viable alternative but in many cases, a preferred option.
Targeted Gene Editing Techniques
The primary allure of Cas9 nickase D10A is its ability to create single-strand breaks in DNA rather than double-strand breaks, as is the case with the wild-type Cas9 enzyme. By doing so, it enables a range of targeted gene editing techniques. Some of these include:
- CRISPR/Cas9 Sterility: For organisms where complete knockout is undesirable, D10A allows the introduction of precise modifications without additional genomic disruption. This is highly beneficial in agriculture for crop improvement, where maintaining plant viability is paramount.
- Gene Insertion and Replacement: The D10A variant can be harnessed in strategies that require inserting new gene sequences or replacing defective ones. A single nick provides room for the cell's natural repair mechanisms to fix the break, potentially incorporating provided donor DNA.
- Base Editing: When used in conjunction with other techniques, such as prime editing, the nickase allows for more controlled edits at specific loci, eradicating the need for double-strand breaks that could lead to unwanted rearrangements in the genome.
These methods emphasize not just the versatility of D10A but also its suitability for addressing diverse biological challenges.
Advantages of Using Nickase
Utilizing Cas9 nickase D10A rather than regular Cas9 presents several advantages that can significantly advance research and therapeutic applications:
- Reduced Off-Target Effects: One of the foremost advantages is the decreased likelihood of off-target effects. The less aggressive nicks made by D10A lead to a more controlled editing process, which, ultimately, minimizes harm to non-target sequences.
- Higher Precision: Precision is key in therapeutic applications, particularly in cases like gene therapy. D10A's targeted approach enhances the efficiency of editing specific genes without affecting others, crucial for applications in medicine.
- Enhanced Repair Mechanisms: The cellular machinery that repairs single-strand breaks behaves differently than that which repairs double-strand breaks. This enables more complex editing strategies whereby the research at a molecular level can achieve inherently more significant outcomes.
- Promotes Homology-Directed Repair: With Cas9 D10A, there is a clearer path for homology-directed repair (HDR). This is particularly advantageous in scenarios requiring precise integrations, as it allows for controlled modifications with high fidelity.
"As the field of genome editing continues to evolve, the choice of tool can mean the difference between success and unwanted mutations. The innovative use of D10A is paving the way for a more refined approach to gene editing."
In summary, the applications of Cas9 nickase D10A in genome editing are both broad and deeply impactful. They herald a shift towards more precise, effective, and safer genetic modifications that are not only applicable in research settings but also stand to revolutionize therapeutic strategies.
Role in Gene Therapy
The incorporation of Cas9 nickase D10A into the narrative of gene therapy is not just a fling; it stands at the forefront of transformative biotechnological advancements. In a world where genetic diseases cast shadows over millions, the potential of this innovative tool becomes ever more crucial. Unlike traditional Cas9, which can lead to unwanted double-strand breaks, the nickase variant offers a more refined approach. It selectively induces single-strand breaks, minimizing the collateral damage often associated with genome editing. This precision can be the difference between a successful therapeutic outcome and unforeseen complications that arise from off-target effects.
Potential in Therapeutic Applications
The therapeutic possibilities unlocked by Cas9 D10A are as diverse as they are promising. It shows potential for tackling genetic disorders like cystic fibrosis, hemophilia, and sickle cell anemia, enhancing the standard repertoire of gene editing. Here’s how it operates:
- Selective Editing: By targeting specific genes, researchers can address the root cause of a disease rather than just managing symptoms.
- Reduced Off-Target Effects: The nickase variant’s single-strand break approach minimizes unintended changes elsewhere in the genome. This means that therapies could be safer and more reliable.
- Compatibility with Homology-Directed Repair (HDR): When paired with HDR, Cas9 D10A can facilitate accurate gene correction. This opens doors to precise edits that were difficult to achieve with traditional methods.
These elements demonstrate how the use of Cas9 D10A is a forward-thinking approach, prioritizing safety and efficacy in genetic endeavors.
Case Studies and Success Stories
Real-world applications echo the theoretical benefits of Cas9 D10A, showcasing its efficacy across various studies. Here are some noteworthy examples:
- Duchenne Muscular Dystrophy (DMD): Researchers have used Cas9 D10A in animal models to correct mutations related to DMD. The outcomes have shown increased muscle function and longevity compared to previous methods.
- Sickle Cell Disease: In preclinical studies, scientists have deployed this nickase in hematopoietic stem cells, resulting in the successful editing of the beta-globin gene. The edited cells demonstrated the potential for long-term correction of the disease phenotype.
"The impact of Cas9 D10A in gene therapy is not an empirical wish; it’s a blossoming reality."
These breakthroughs signify just the tip of the iceberg. As researchers continue to refine techniques and explore new avenues for application, the scope for Cas9 D10A remains vast. The journey of this nickase variant in gene therapy underlines a significant shift towards precision medicine, making it a vital player in our quest to eradicate genetic disorders.
Challenges and Limitations
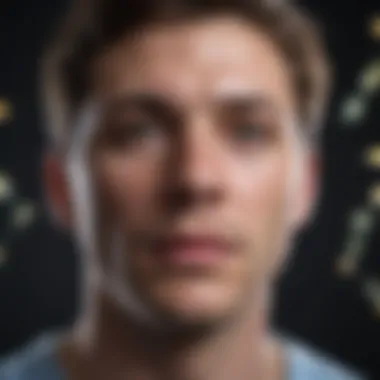
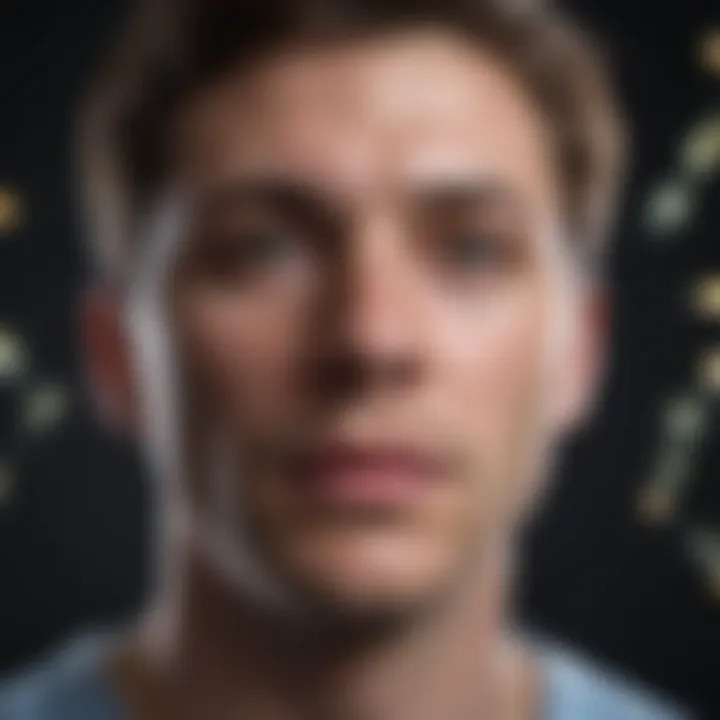
In the realm of genome editing, every tool comes with its share of hurdles, and Cas9 nickase D10A is no exception. Grasping the complexities surrounding its challenges and limitations is vital for scientists, especially those who aim to maximize its potential while mitigating risks. Acknowledging these aspects forms the bedrock of responsible research and application in gene therapy and other biotechnological fields.
Off-Target Effects
One of the foremost concerns when employing Cas9 nickase D10A is the risk of off-target effects. Unlike a precision instrument that cuts exactly where intended, the process may err, creating unintended modifications elsewhere in the genome. This phenomenon can stem from various factors, including the degree of complementarity between the guide RNA and the target DNA sequence.
The probability of these off-target alterations can lead to unforeseen consequences. For instance, a gene that is meant to be edited may end up not being the only one affected, resulting in adverse cellular outcomes. This can be particularly troublesome in therapeutic applications where safety is paramount.
"Understanding off-target effects isn’t just about science; it's about the ethics of interweaving human health with genetic modifications."
Strategies to minimize these risks include optimizing guide RNA design to enhance specificity and using screening methods like whole-genome sequencing to identify and address off-target mutations. Researchers are continually devising innovative techniques to improve accuracy, effectively trying to hit the bullseye every single time with minimal collateral damage.
Technical and Ethical Considerations
On a broader scale, technical limitations can hinder the efficiency and efficacy of Cas9 nickase D10A. Factors such as delivery methods, the cellular environment, and variations in target cell types all play significant roles in the success of genome editing endeavors. For instance, viral vectors, commonly used for delivering CRISPR components, can trigger immune responses or integrate into unintended genomic sites, raising safety concerns.
Moreover, ethical considerations are paramount when utilizing such powerful tools. The possibility of germline genome editing—alterations that are heritable—sparks heated debates. Questions arise about the implications of 'playing God' and whether we have the right to alter human genetics in ways that could pass down through generations. Regulatory frameworks are still catching up to these advancements, necessitating a careful approach as we navigate the blurred lines between innovation and morality.
The balance of advancing scientific knowledge while ensuring ethical integrity is crucial. As genome editing technologies further their reach, the conversation surrounding limitations must continue, driving the development of more rigorous standards in research and application. Ultimately, a thoughtful integration of scientific rigor and ethical governance will be essential to harnessing the full capabilities of Cas9 nickase D10A.
Future Perspectives
The landscape of genome editing is constantly shifting, and the role of Cas9 Nickase D10A is becoming increasingly pivotal. As researchers push the envelope, innovations in this area could supercharge therapies, boost agricultural advances, and sharpen tools for biological research. Understanding these future perspectives is key for anyone interested in genetic manipulation.
Using Cas9 Nickase D10A could lead to more precise editing techniques. Researchers aim to go beyond the typical double-stranded breaks, which could inadvertently cause unwanted mutations. The targeted single-strand cuts made by this protein variant promise not just a higher specificity in gene editing but also an improved safety profile, minimizing the chances of off-target effects.
Emerging Trends in Genome Editing
Recent advancements bring a plethora of new methods to the forefront of genome editing. Some notable directions include:
- Base Editing: A game changer derived from CRISPR technology that enables conversion of one DNA base into another without cutting both strands, significantly reducing the risks of undesired mutations.
- Prime Editing: Often referred to as the “search and replace” technique, this method provides unparalleled accuracy and versatility in modifying genes.
- Epigenome Editing: Rather than altering the actual DNA sequence, this trend focuses on modulating gene expression through epigenetic modifications. This could be particularly useful for conditions where gene function is more critical than sequence itself.
The emergence of these techniques highlights a trend towards precision and reducing potential risks associated with gene editing, establishing a basis for the integration of Cas9 Nickase D10A in future applications. The combination of these cutting-edge technologies suggests a promising horizon for therapeutic development.
The Role of Cas9 Nickase D10A in Research Advancements
As the scientific community plunges into the nitty-gritty of genomic alterations, Cas9 Nickase D10A stands out as a versatile tool that could catalyze several research advancements. It not only shows potential in targeted gene therapy but also opens doors for other applications such as:
- Functional Genomics Studies: By enabling more precise gene editing, research can delve deeper into gene functions and interactions, revealing insights into complex biological systems.
- Disease Modeling: The specificity of Cas9 D10A facilitates the creation of models that mimic human diseases more closely, aiding in the development of effective treatments.
- Synthetic Biology: Leveraging the unique capabilities of this tool, scientists can design and construct new biological parts, devices, and systems, propelling forward industrial and environmental applications.
The importance of Cas9 Nickase D10A in advancing our understanding of genome dynamics cannot be overstated. It serves not just as a tool but as a beacon guiding future researchers in the intricate world of genetic modification.
Epilogue
In the ever-evolving field of genome editing, the significance of Cas9 nickase D10A cannot be overstated. This article elucidates various aspects of this unique variant, illuminating its distinct functionalities and potential within genetic modification. Through thorough examination of its structure, mechanisms, and practical applications, several key insights have surfaced that reveal why understanding Cas9 D10A is crucial for both educational pursuits and advanced research.
Summarizing Key Insights
- Structural Uniqueness: The D10A variant modifies the cut mechanism of standard Cas9, leading to a more precise editing tool in genetic engineering.
- Versatile Applications: As highlighted, D10A features prominently not only in targeted gene editing but also shows promise in therapeutic contexts, providing pathways for treating genetic disorders.
- Off-Target Reduction: With a focus on single-strand breaks rather than double-strand breaks, Cas9 D10A minimizes unintended mutations, thus increasing accuracy in genetic modifications.
- Ethical Considerations: Challenges related to ethical implications and the necessity for stringent regulations surrounding gene editing technologies have been acknowledged, stressing the need for responsible applications.
Call for Continued Research
The intricate nature of genetic manipulation means that exploration surrounding Cas9 nickase D10A is far from over. Ongoing study and experimentation are vital. Here are a few areas worth delving into:
- Broader Applications: Investigating the evaluation of D10A in diverse organisms, which could unlock its potential across various fields such as agriculture or biotechnology.
- Long-term Effects: Understanding the long-term implications of editing via D10A will provide insight into stability and efficacy over generations, paving the way for safe therapeutic applications.
- Technical Advancements: As tools and methodologies in genetics progress, refining the delivery mechanisms for CRISPR components like D10A will likely enhance its usability in clinical settings.
In summary, though we stand at the brink of a new era in genome editing, thorough examination and an unquenchable thirst for knowledge about tools like Cas9 D10A will ensure a safe and groundbreaking future in genetic research. We should all advocate for continued studies and remain vigilant in addressing any challenges that may arise.