CDNA RT: A Comprehensive Overview of Techniques
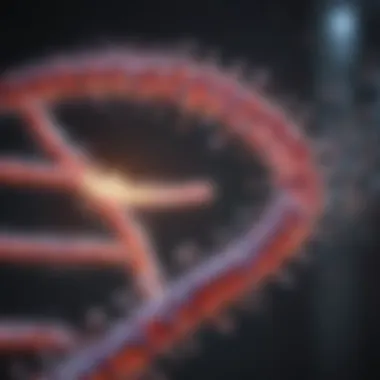
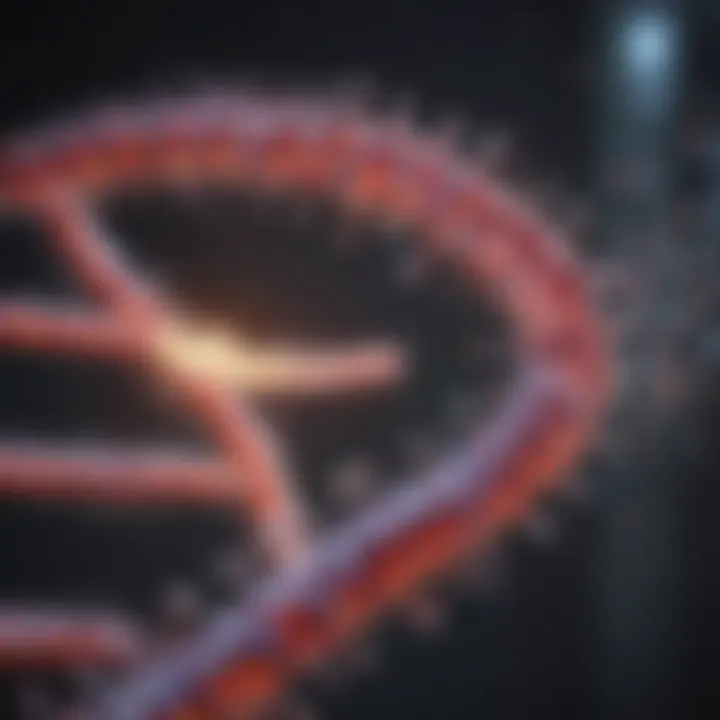
Intro
Complementary DNA (cDNA) synthesis via reverse transcription is a crucial technique in molecular biology. This method allows researchers to analyze gene expression by converting RNA into DNA. Understanding cDNA RT is essential for advancements in diagnostics, biotechnology, and genomics. This overview aims to explain the foundational aspects and current trends in cDNA synthesis.
Research Overview
Methodological Approaches
The process of cDNA synthesis involves several key steps. First, messenger RNA (mRNA) is isolated from the sample of interest. Reverse transcriptase enzymes then transcribe this mRNA into cDNA. There are different types of reverse transcriptases, each with specific characteristics that optimal for various applications. Some of the commonly used enzymes are M-MuLV and AMV reverse transcriptases.
Key Steps in cDNA Synthesis:
- RNA Extraction: Use kits or organic solvents for efficient RNA isolation from cells.
- Reverse Transcription Reaction: Mix mRNA with reverse transcriptase, nucleotides, and primers, then incubate to facilitate cDNA synthesis.
- Quality Control: Validate cDNA synthesis through techniques like quantitative PCR or gel electrophoresis.
The quality of the cDNA synthesized can significantly impact downstream applications, including RT-qPCR, cloning, and sequencing. Different methodologies can be applied depending on the specific needs of a research project.
Significance and Implications
The implications of cDNA RT are vast. It serves as a foundation for numerous molecular biology applications. Understanding gene expression is essential for studying diseases, understanding development, and improving genetic engineering techniques. cDNA synthesis has also proven significant in the field of diagnostics, where it helps in detecting viral RNA, like in the case of HIV or SARS-CoV-2.
"cDNA synthesis is essential in modern biology to unveil the complexities of gene expression and regulation."
Current Trends in Science
Innovative Techniques and Tools
Recent advancements in technology have enhanced the efficiency and accuracy of cDNA synthesis. Newer tools like high-throughput sequencing and digital PCR are being integrated with traditional methods. These innovations allow for a more comprehensive analysis of the transcriptome, providing deeper insights into gene expression profiles.
Examples of Innovative Tools:
- Nanopore Sequencing: Enables real-time sequencing of RNA molecules.
- CRISPR-based Methods: Permits targeted modifications and evaluations of gene expression.
Interdisciplinary Connections
The integration of cDNA synthesis into various scientific fields underscores its importance. It connects molecular biology with areas like bioinformatics and genetics. As researchers increasingly collaborate across disciplines, the understanding and application of cDNA techniques will continue to advance.
This convergence not only enhances research but also leads to new methodologies that push the boundaries of existing knowledge. The continuous development in cDNA RT methodologies indicates a promising future, where precision in molecular analysis will offer deeper insights into biology.
Prolusion to CDNA and RT
Understanding complementary DNA (cDNA) synthesis via reverse transcription (RT) is essential in molecular biology. This process allows researchers to convert RNA sequences into a stable DNA form, facilitating various experimental applications. The significance of cDNA lies in its versatility for gene expression studies, diagnostics, and even therapeutic developments. Researchers can measure levels of specific transcripts in biological samples, making cDNA synthesis a keystone in genetic and genomic analysis.
Defining cDNA
Complementary DNA, or cDNA, is synthesized from a messenger RNA (mRNA) template through the action of the enzyme reverse transcriptase. This enzymatic process reverses the usual flow of genetic information in cells, where DNA is typically transcribed into RNA. By converting RNA back into DNA, cDNA provides a means to study gene expression in a stable format. cDNA replicates the mRNA's coding sequence, making it invaluable for cloning, sequencing, and analysis of the transcriptome. Essentially, cDNA acts as a complementary copy of the original RNA, allowing researchers to explore gene function and regulation.
Understanding Reverse Transcription
Reverse transcription is the process utilized to generate cDNA from RNA. The process begins with the addition of reverse transcriptase to an RNA sample. This enzyme catalyzes the synthesis of a complementary DNA strand, utilizing the RNA as a template. The efficiency of this process can vary greatly and is influenced by several factors. Key amongst them is the quality and quantity of the RNA input.
Reverse transcriptase not only lacks the proofreading capability present in many DNA polymerases but also demonstrates a preference for specific RNA sequences. Therefore, optimizing conditions during reverse transcription is crucial to achieving accurate and reliable cDNA synthesis.
Understanding these principles allows scientists to design experiments thoughtfully and choose appropriate methods for their specific research needs. As reverse transcription is the foundation for cDNA synthesis, it is paramount to grasp its mechanics and implications in molecular biology.
The Science Behind CDNA Synthesis
The significance of understanding the science behind cDNA synthesis cannot be overstated. cDNA synthesis via reverse transcription plays a critical role in molecular biology, allowing researchers to investigate gene expression and function. This section delves into the fundamental elements that contribute to effective cDNA synthesis. Specifically, we examine the principles of synthesis, the function of reverse transcriptase, and the selection of appropriate templates. These components combine to facilitate accurate synthesis.The understanding of these scientific principles empowers researchers to optimize protocols and enhance their experimental outcomes.
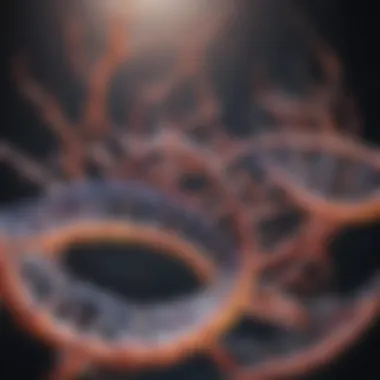
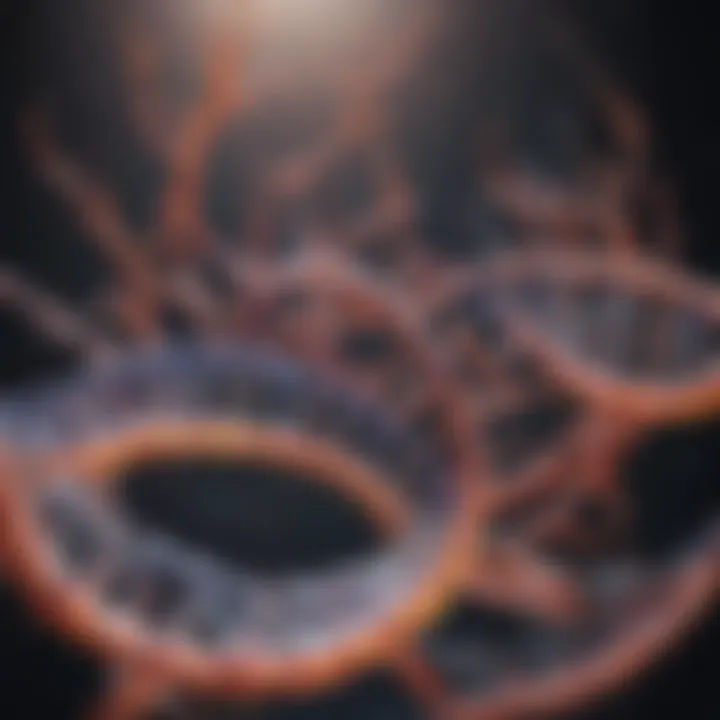
Basic Principles of Synthesis
The basic principles of cDNA synthesis rest on the conversion of RNA into complementary DNA. This process requires the action of an enzyme known as reverse transcriptase. By treating RNA with this enzyme, researchers can create a DNA strand that mirrors the RNA template. This transformation is crucial, as it allows the stable genetic material (cDNA) to be used in various applications such as cloning, PCR, and sequencing.
Key aspects of the synthesis process include:
- Isolating RNA: It is essential to start with high-quality RNA. Contaminated or degraded RNA can lead to poor cDNA synthesis.
- Reverse Transcriptase Reaction: The reverse transcriptase enzyme acts in a mixture that may include buffers, primers, and nucleotides, enabling the synthesis of cDNA.
- Temperature Considerations: Reverse transcription typically requires a specific temperature range to ensure optimal enzyme activity and minimize degradation.
Role of Reverse Transcriptase
Reverse transcriptase is a core component of the cDNA synthesis process. This enzyme has the ability to synthesize DNA from an RNA template, making it indispensable in the study of gene expression. It is derived from retroviruses, signifying its natural role in replicating viral RNA into DNA within host cells.
The types of reverse transcriptase can differ in their characteristics, such as:
- Processivity: Some enzymes remain attached to the RNA longer than others, improving synthesis efficiency.
- Specificity: Variations in enzyme specificity can affect the types of RNA that can be reverse transcribed.
Utilizing a reverse transcriptase with optimal features can substantially enhance the yield and quality of cDNA produced. Furthermore, researchers often source these enzymes from commercial suppliers to ensure reliability.
Template Selection for CDNA
The selection of the RNA template is vital for successful cDNA synthesis. Various types of RNA, including mRNA, rRNA, and non-coding RNA, can be transformed into cDNA. Researchers must discern which RNA type will best suit their experimental needs.
When choosing a template, several factors should be considered:
- RNA Quality: High-purity RNA will yield the best results in cDNA synthesis.
- Template Length: Longer RNA molecules can impact the synthesis process, as they may lead to incomplete transcription.
- Target Genes: Specificity is critical when selecting templates for cDNA synthesis. Researchers should prioritize templates that are relevant to their investigation.
By carefully selecting templates and acknowledging the principles of the synthesis process, researchers can significantly improve experimental outcomes in their work with cDNA.
Materials and Methods in CDNA RT
The study of complementary DNA (cDNA) synthesis through reverse transcription (RT) greatly relies on the appropriate selection of materials and methods. This phase is vital because it completely influences the quality and results of the synthesized cDNA. In the realm of molecular biology, precise protocols empower researchers to yield reliable data and drive innovative findings. Here, we will briefly discuss the essential reagents, synthesis protocols, and optimization techniques essential for successful cDNA RT.
Required Reagents
The selection of reagents for cDNA synthesis is critical. Each component plays a unique role in the process. Some key reagents include:
- RNA Template: The starting material, a high-quality RNA sample, is crucial. This can be total RNA or messenger RNA (mRNA).
- Reverse Transcriptase Enzyme: Enzymes like M-MLV or AMV are typically used to convert RNA into cDNA.
- Primers: These are necessary for starting the synthesis. Oligo(dT) primers or random hexamers are common choices.
- dNTPs: Deoxynucleotide triphosphates serve as the building blocks for cDNA synthesis.
- Buffer Solutions: They ensure optimal conditions for the enzyme reactions, including temperature and pH balance.
Proper assessment of the quality and purity of these reagents impacts the overall success of the experiment. Contaminants or degraded RNA can lead to unreliable cDNA synthesis.
Protocols for Synthesis
Once the appropriate reagents are ready, following a systematic protocol is essential. Here is a general outline of the synthesis process:
- Preparation of the RNA Template: Isolate total RNA or mRNA from cells or tissues. Use methods like phenol-chloroform extraction or column-based purification.
- Mixing with Primers and dNTPs: Combine the RNA template with the chosen primers and dNTPs in an RNase-free microcentrifuge tube.
- Reverse Transcription Reaction: Add reverse transcriptase enzyme and buffer. Incubate at a specific temperature for a defined duration, typically between 37-42Β°C for 60 minutes.
- Inactivation of the Enzyme: Heat the reaction mixture to inactivate the enzyme, commonly at 70Β°C for 5-15 minutes.
- Storage: cDNA can be stored at -20Β°C for later use.
This basic protocol can need modifications depending on specific experimental requirements. Details such as incubation times and temperatures might vary.
Optimization Techniques
To achieve optimal synthesis outcomes, various techniques can be used:
- Temperature Adjustments: Finding the right temperature for the reverse transcription step fosters efficient synthesis.
- Primer Selection: Different primers can enhance yield and specificity. Testing various primers might improve results.
- Increased Enzyme Concentration: Sometimes, adding more reverse transcriptase can enhance efficiency, especially for low-concentration RNA samples.
- Time Extension: Lengthening the incubation time can improve cDNA yield, particularly when working with less abundant RNA templates.
In molecular biology experiments, optimization is an ongoing process. Different experiments may require different approaches. Achieving the best results can necessitate several iterations and adjustments.
"Optimization in reverse transcription is not just a technical endeavor; it is a critical pathway to generating reproducible and credible molecular data."
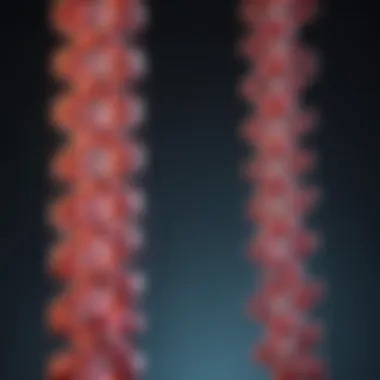
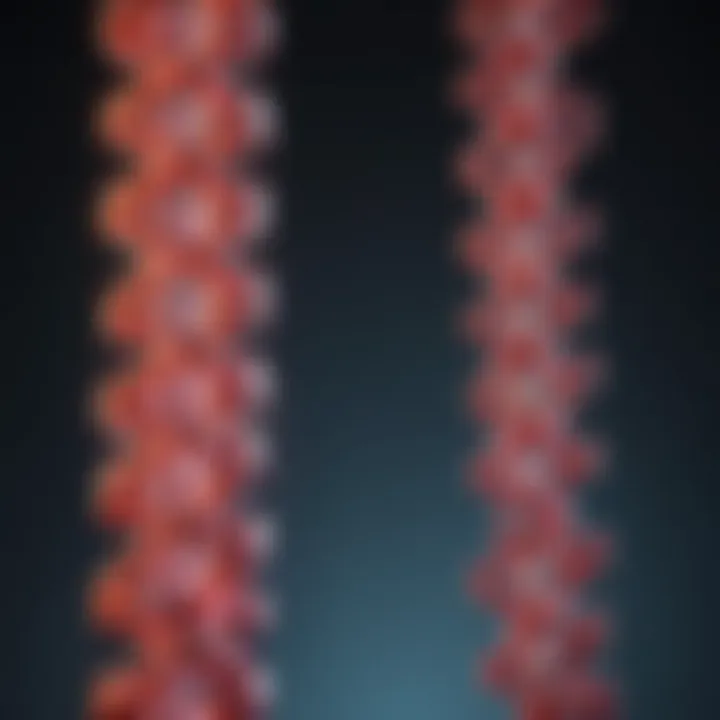
Applications of CDNA RT
The Applications of cDNA RT are critical for understanding its impact in molecular biology. These applications span several domains, including gene expression analysis, the creation of cDNA libraries, and diagnostic applications. Each application serves unique purposes and expands our knowledge of biological systems. Understanding these applications can offer insights and drive further research in genetics, biotechnology, and clinical settings.
Gene Expression Analysis
Gene expression analysis is one of the primary uses of cDNA synthesis. By converting mRNA into cDNA, researchers can measure the expression levels of specific genes under various conditions. This analysis is pivotal in studying cellular responses to stimuli, disease progression, and developmental stages.
The process often involves quantitative techniques such as real-time polymerase chain reaction (PCR) to quantify the generated cDNA. The results help elucidate functional roles of genes, providing a clearer understanding of their activity within cells. This approach is fundamental in research areas such as cancer genomics and personalized medicine.
Moreover, greater accuracy in quantifying gene expression can inform drug development and treatment strategies. As gene expression can vary based on environmental changes, cDNA RT provides a reliable method to track these variations in a controlled manner.
Creation of cDNA Libraries
Creating cDNA libraries involves synthesizing cDNA from mRNA collections, which can then be cloned into vectors for further study. This method enables the preservation of expressed genes from a specific tissue or condition, allowing researchers to analyze gene function comprehensively.
Such libraries facilitate the discovery of new genes, alternative splicing events, or novel transcripts. Applications of cDNA libraries extend to functional genomics, where scientists can explore gene interactions and regulatory networks. For geneticists, having access to a rich cDNA library is invaluable for experimental work.
The construction and utilization of these libraries also empower comparative genomics. Researchers can compare expression profiles across different species or conditions, providing insights into evolutionary processes and functional adaptations.
Diagnostic Applications
In diagnostic contexts, cDNA RT plays a monumental role in developing tests for infectious diseases and genetic disorders. The ability to detect and quantify specific RNA molecules through cDNA synthesis allows for rapid and reliable diagnostics.
For instance, in virology, the detection of viral RNA can indicate active infections. This application is crucial for diseases such as HIV or SARS-CoV-2, where early detection is key to effective management. cDNA RT enables the development of sensitive assays that not only identify pathogens but also quantify viral loads.
Furthermore, cDNA synthesis aids in genetic testing for inherited disorders by analyzing mRNA levels of genes associated with specific diseases. This capacity for precise quantification directly impacts patient diagnosis and management, supporting personalized treatment plans tailored to genetic profiles.
Ultimately, the diverse applications of cDNA RT underscore its central role in contemporary molecular biology. From unraveling gene functions to advancing diagnostics, cDNA RT remains an indispensable tool.
Quantitative CDNA Techniques
Quantitative cDNA techniques are crucial in molecular biology research. These methods allow researchers to measure the amount of specific RNA in samples. This quantification is essential for understanding gene expression levels, which can vary in different conditions or treatments. By employing quantitative techniques, scientists can gain insights into how genes function and interact within biological systems.
One of the key elements of quantitative cDNA methods is their ability to provide precise data. This precision can aid in identifying small changes in gene expression that may be significant in a biological context. Moreover, the use of quantitative techniques helps to standardize results across experiments, making comparisons easier and more reliable.
Real-Time PCR Integration
Real-Time PCR, also known as quantitative PCR (qPCR), is a fundamental technique in quantitative cDNA methodologies. This method allows for the amplification of DNA while simultaneously measuring the amount of cDNA in real time. The integration of real-time PCR into cDNA techniques provides several advantages:
- Sensitivity: Real-time PCR can detect minute quantities of cDNA, making it suitable for experiments where samples are limited.
- Speed: Results can be obtained quickly, reducing the time required to analyze samples compared to traditional PCR methods.
- Specificity: The technique employs specific primers, ensuring that only the target cDNA is amplified, which minimizes contamination and improves accuracy.
Data Analysis Methods
Once the quantitative data is generated from real-time PCR, robust data analysis methods are essential. Analyzing expression data can require careful consideration of several factors:
- Normalization: Before interpreting results, it is essential to normalize the data against reference genes. This ensures accuracy in measuring relative expression levels.
- Threshold Cycle (Ct) Analysis: The threshold cycle is the point at which the fluorescence signal exceeds a background level. Analyzing Ct values allows researchers to infer the initial amount of target cDNA in the sample.
- Software Tools: Various software tools are available for data analysis, such as the comparative Ct method or absolute quantification methods. These tools support researchers in generating meaningful insights from their data.
In summary, quantitative cDNA techniques play a pivotal role in contemporary molecular biology. Integrating real-time PCR with precise data analysis methods facilitates a better understanding of gene expression and its implications in biology and medicine.
Challenges in CDNA RT
In the realm of molecular biology, understanding cDNA synthesis via reverse transcription is crucial, yet it is fraught with challenges that can affect experimental outcomes. Addressing these challenges is imperative for achieving reliable and reproducible results. The difficulties in this area can obstruct the interpretation of gene expression data, thus influencing subsequent research and diagnostic applications. This section will dissect common pitfalls and technical limitations encountered during cDNA synthesis.
Common Pitfalls
Numerous common pitfalls may arise when performing cDNA reverse transcription. These pitfalls can lead to reduced efficiency and reliability of synthesized cDNA. Below are some specific concerns:
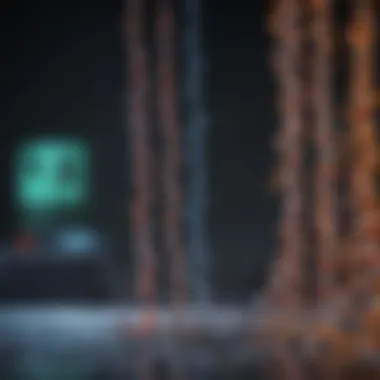
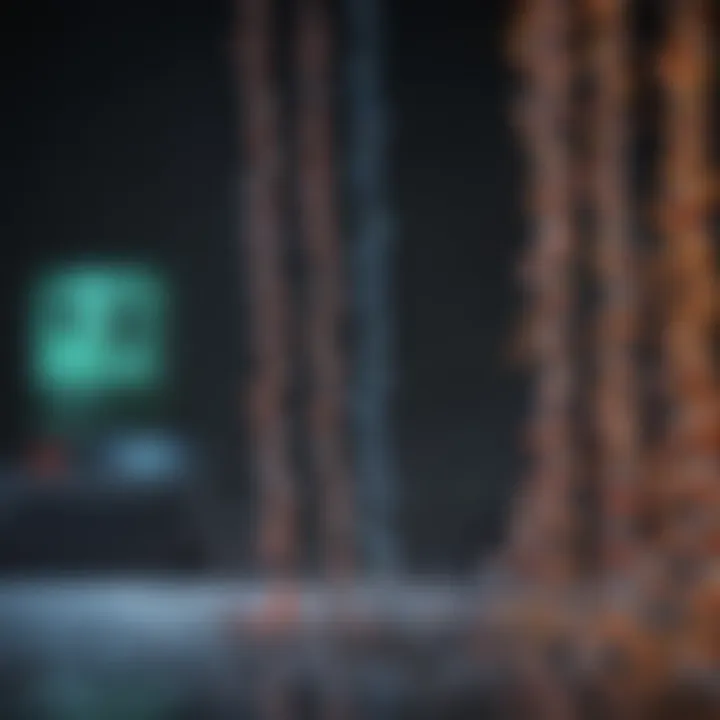
- Quality of RNA: The integrity of the RNA used is critical. Degraded RNA can yield poor-quality cDNA. It is important to use high-quality, intact RNA to ensure accurate representation of the original mRNA.
- Enzyme Performance: The reverse transcriptase enzyme is sensitive to various conditions. Keeping the enzyme at optimal temperatures and avoiding freeze-thaw cycles is essential.
- Reaction Conditions: Inappropriate reaction conditions, such as incorrect pH or ionic strength, can hinder the efficiency of reverse transcription. Carefully following established protocols can mitigate these issues.
- Primer Design: Poorly designed primers can lead to non-specific amplification. Using optimized primers that suit the target sequence is critical.
"Ensuring RNA quality and precise reaction conditions directly influences cDNA synthesis success."
The above factors emphasize the need for careful preparation and meticulous attention to detail throughout the reverse transcription process. Ignoring these considerations may lead to erroneous conclusions in downstream applications, such as gene expression analysis or diagnostic evaluations.
Technical Limitations
The technical limitations encountered in cDNA synthesis can also impede research progress. Such limitations include:
- Quantitative Variability: Variability in cDNA yield can affect quantification of gene expression. Some factors contributing to this variability include enzyme batch-to-batch differences and variations in the template RNA quantity.
- Sensitivity to Contaminants: Contaminants such as genomic DNA can interfere with the reaction and create misleading results. It is essential to employ purification steps to reduce the presence of DNA.
- Optimization is Required: The need for optimization can be seen as a barrier, especially for lower expertise laboratories. Different RNA templates may require distinct conditions, thus complicating standardization among experiments.
These limitations highlight the necessity for researchers to carefully evaluate their methodologies and routinely refine their techniques to achieve optimal results in cDNA RT studies. By understanding these pitfalls and limitations, one can better navigate the complexities of cDNA synthesis, leading to more informative research findings.
Future Directions in CDNA RT Research
The exploration of cDNA synthesis via reverse transcription holds great promise for the future. As science progresses, new techniques and applications emerge, making this area of study increasingly relevant. Understanding these future directions can illuminate the potential benefits for various fields, especially molecular biology.
Innovations in Synthesis Techniques
Recent studies indicate that optimizing synthesis techniques can significantly improve the efficiency of cDNA generation. New methods such as hot-start reverse transcription and the utilization of advanced polymerases can enhance yield and specificity, which are crucial for downstream applications.
Some key innovations to note include:
- Single-Cell cDNA Synthesis: This method allows for the analysis of gene expression profiles at the single-cell level. It can reveal heterogeneity in cell populations, which is necessary for understanding complex biological systems.
- Nanopore Sequencing: Integrating nanotechnology with cDNA synthesis can improve the speed and accuracy of sequencing results, facilitating real-time analysis of genetic material.
- Automated Platforms: Automation in cDNA synthesis protocols reduces the chances of human error and increases reproducibility. It allows labs to handle more samples efficiently, which is essential for large-scale studies.
These advancements not only streamline processes but also expand the boundaries of what cDNA RT can achieve in research applications.
Expansion to Clinical Applications
The applicability of cDNA synthesis is extending into clinical settings, offering substantial benefits in diagnostics and therapeutics. The ability to analyze gene expression profiles can lead to breakthroughs in understanding diseases at a molecular level.
One noteworthy aspect is:
- Cancer Research: cDNA synthesis plays a vital role in identifying biomarkers for cancer detection and treatment. With precise expression data, personalized medicine can improve patient outcomes through tailored therapies.
- Infectious Disease Diagnostics: Real-time detection of viral or bacterial RNA can be achieved through cDNA synthesis. This technique is crucial during outbreaks and can guide appropriate medical responses.
- Genetic Disorders: Understanding the underlying genetic causes of disorders can help in developing targeted gene therapies that address specific mutations.
The transition from basic research to clinical applications necessitates collaboration between researchers and healthcare professionals. By leveraging advanced techniques in cDNA synthesis, there is potential for significant improvements in diagnosis and treatment, ultimately benefiting patient welfare and public health.
"The future of cDNA research lies in its application beyond the bench, translating discoveries into effective clinical practices that can genuinely transform patient care."
The continuous advancements in both synthesis methods and clinical applications promise a rich future for cDNA RT research, opening the door to new frontiers in molecular biology and its practical applications.
Culmination
The conclusion of this article serves as the pivotal wrap-up that encapsulates the essence and significance of complementary DNA synthesis via reverse transcription. It brings together the various components discussed earlier, highlighting how cDNA RT is not merely a technique but a foundational methodology within molecular biology that influences numerous research avenues.
One of the primary benefits of understanding cDNA RT lies in its direct relevance to gene expression analysis. With this approach, researchers can assess which genes are active in different conditions or developmental stages. This understanding is crucial for advancements in genetic research, disease diagnosis, and therapeutic interventions.
The implications of cDNA RT extend beyond basic research into practical applications in biotechnology. The creation of cDNA libraries and gene expression profiles enables scientists to tackle complex biological questions. Additionally, integrating cDNA RT with real-time PCR enhances quantitative analysis, further expanding its utility in labs worldwide.
To summarize, the conclusion finds that cDNA RT is not isolated to a single domain; its reach influences diagnostics, therapeutics, and our comprehensive understanding of genetics. It posits that ongoing research in this area will yield exciting advancements, shaping the future of molecular biology.
Summary of Key Points
- cDNA synthesis via RT is crucial for studying gene expression.
- Reverse transcriptase plays a vital role in the transcription process, converting RNA into cDNA.
- Applications include gene expression analysis, creating cDNA libraries, and diagnostics.
- Quantitative techniques such as real-time PCR integrate with this methodology for enhanced data validity.
- Challenges include common pitfalls and technical limitations, which need addressing for optimal results.
Implications for Future Research
Research in cDNA RT opens numerous avenues for exploration. One significant implication is the innovation of synthesis techniques. Developing novel methods could enhance efficiency, specificity, and yield in cDNA synthesis. The ongoing improvements in enzyme technology and reaction conditions hold potential for groundbreaking advancements.
Moreover, expanding applications to clinical spheres is another promising direction. As understanding deepens, integrating cDNA RT in diagnostics could lead to better disease management strategies, including personalized medicine.
In summary, the future of cDNA RT research is bright. It stands to further our knowledge of genetics, enhance research methodologies, and potentially revolutionize clinical diagnostics and therapeutic strategies.