Chloroform-Isopropyl Alcohol RNA Extraction Method
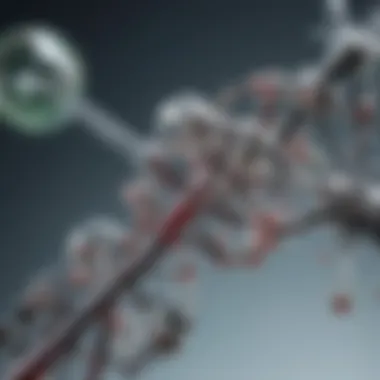
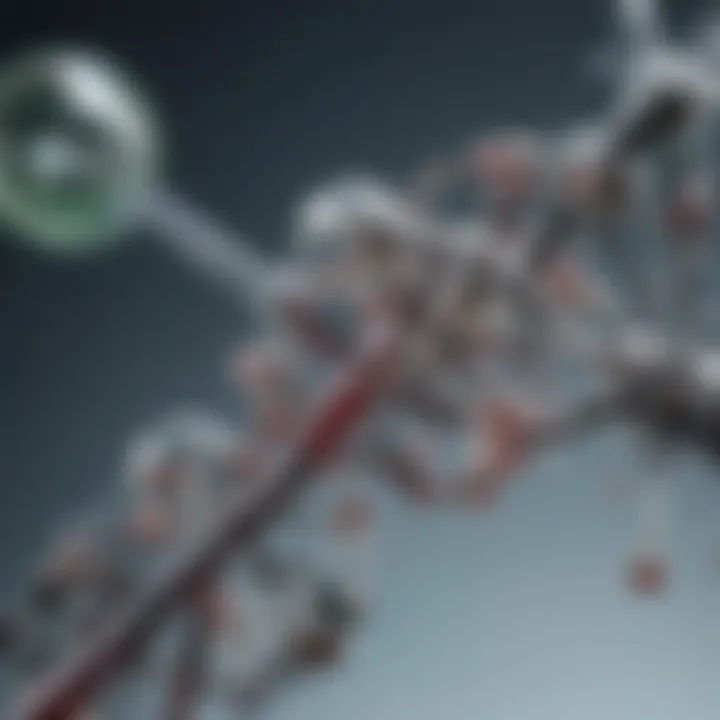
Intro
RNA extraction is an essential procedure in molecular biology, serving as a foundation for various applications such as gene expression analysis, transcription studies, and sequencing. Among the diverse methods available, the chloroform-isopropyl alcohol technique stands out for its effectiveness and efficiency. It utilizes the distinct properties of chloroform and isopropyl alcohol to separate RNA from other cellular components, allowing researchers to obtain high-quality nucleic acids for further experimentation. The following sections will provide a comprehensive overview of this method, detailing its principles, protocols, as well as troubleshooting tips.
Research Overview
Methodological Approaches
The chloroform-isopropyl alcohol method is based on the principle of phase separation, which occurs when specific solvents are added to an aqueous sample containing RNA. Chloroform, a non-polar solvent, lowers the polarity of the overall mixture, promoting the partitioning of molecules based on their solubility. Once chloroform is added, the solution separates into three layers: the upper aqueous phase containing RNA, the interphase containing DNA, and the lower organic phase containing proteins and lipids. Isopropyl alcohol is subsequently introduced to precipitate the RNA from the aqueous phase, making it easier to collect through centrifugation.
This method has proven to be valuable for isolating RNA from various biological samples, including plant tissues, animal cells, and microbial cultures, thanks to its straightforward protocol and the effectiveness in yielding high-quality RNA.
Significance and Implications
The significance of RNA extraction using this method cannot be overstated. High-quality RNA is crucial for reliable results in downstream applications, including PCR, qPCR, and RNA sequencing. Moreover, the chloroform-isopropyl alcohol method offers several benefits, such as cost-effectiveness, availability of reagents, and compatibility with different types of samples.
"The chloroform-isopropyl alcohol method remains a cornerstone in RNA extraction protocols, demonstrating its continued relevance in modern genetic research."
Current Trends in Science
Innovative Techniques and Tools
Recent advancements in molecular biology have seen a shift toward automation and improved efficiency in RNA extraction. While the traditional chloroform-isopropyl alcohol method is still highly regarded, newer techniques such as spin-column and magnetic bead-based methods are gaining popularity. These methods promise to reduce extraction time and minimize contamination risks.
Moreover, integrating RNA extraction with next-generation sequencing technologies has expanded the potential applications, allowing researchers to explore complex transcriptomes and gene regulation networks with greater precision.
Interdisciplinary Connections
RNA extraction techniques, including the chloroform-isopropyl alcohol method, have found relevance across various scientific disciplines. For instance, in environmental sciences, RNA extraction is utilized to study microbial communities and their functional capabilities within ecosystems. In clinical research, the method aids in identifying biomarkers for diseases by analyzing RNA profiles from patient samples.
A thorough understanding of RNA extraction methods enhances collaborative efforts among researchers from diverse fields, driving innovation and discovery in the life sciences.
Preface to RNA Extraction
RNA extraction is a pivotal process in molecular biology and genetic research. This method is essential for analyzing gene expression, studying RNA viruses, and elucidating various cellular mechanisms. RNA, or ribonucleic acid, serves as a crucial intermediary in the expression of genetic information. Understanding the intricacies of RNA extraction enables researchers to obtain high-quality RNA samples, which are vital for downstream applications such as polymerase chain reaction (PCR) and RNA sequencing.
The ability to extract RNA efficiently and accurately affects the clarity and reliability of experimental results. High-quality RNA extraction ensures that researchers can conduct reproducible studies and obtain valid data, impacting the conclusions drawn from research findings significantly. As the demand for RNA-based diagnostics and therapeutics rises, it becomes increasingly relevant to master RNA extraction techniques.
Importance of RNA Extraction in Research
The significance of RNA extraction in the field of research cannot be overstated. First, it allows scientists to study gene expression patterns, which lends insights into cellular behavior. Investigating different RNA molecules, such as mRNA, rRNA, and tRNA, enables researchers to ascertain how genes are turned on or off in various conditions.
Moreover, RNA extraction plays a crucial role in medical research, especially in understanding diseases at a molecular level. For instance, viral RNA extraction is vital for studying RNA viruses such as the influenza virus or SARS-CoV-2, leading to vaccine development and therapeutic strategies. Additionally, the analysis of non-coding RNAs has opened new avenues in cancer research, aiding in the identification of biomarkers for diagnosis and treatment.
Overview of RNA Types
RNA molecules are diverse and have specific roles within biological systems. The key types of RNA include:
- mRNA (Messenger RNA): This type conveys genetic information from DNA to ribosomes for protein synthesis. Analysis of mRNA allows researchers to understand which genes are expressed under specific conditions.
- rRNA (Ribosomal RNA): It is a fundamental component of ribosomes, facilitating protein synthesis. Studies on rRNA can provide insights into ribosome biogenesis and function.
- tRNA (Transfer RNA): These molecules transport amino acids to the ribosome during protein synthesis. Understanding tRNA levels can reflect translational efficiency.
- siRNA (Small Interfering RNA): These are involved in gene silencing via RNA interference, offering tools for genetic manipulation.
- miRNA (MicroRNA): These short, non-coding RNAs regulate gene expression and have implications in various diseases, including cancers.
By comprehending these diverse classes, researchers can choose the appropriate extraction methods tailored to their specific needs, ultimately enhancing the quality and applicability of their findings.
Chloroform and Isoamyl Alcohol: Properties and Functions
The combination of chloroform and isoamyl alcohol plays a pivotal role in the RNA extraction process. Understanding their properties is essential for researchers looking to achieve high-quality results in molecular analysis. Chloroform serves as a powerful solvent that assists in the liberation of RNA while isoamyl alcohol enhances phase separation. Together, they optimize the efficiency of RNA extraction, making this combination a common choice in laboratories.
Chemical Properties of Chloroform
Chloroform, with the chemical formula CHCl₃, is a colorless, dense liquid with a discernible sweet odor. Its properties as a solvent are crucial for RNA extraction. Chloroform is hydrophobic and has a low polarity, making it effective in dissolving organic compounds without interfering with the aqueous phase that contains the nucleic acids.
Chloroform's density is greater than that of water, allowing it to separate distinctly during centrifugation. This property is vital as it aids in displacing other DNA and protein contaminants, resulting in purer RNA. Moreover, since chloroform is a volatile compound, it evaporates easily, which is advantageous during certain procedural steps.
Role of Isoamyl Alcohol in RNA Extraction
Isoamyl alcohol, also known as 3-methyl-1-butanol, is often added along with chloroform to improve the RNA extraction process. This addition enhances the phase separation and reduces the emulsification that can occur, leading to a clearer separation of RNA from proteins and other cellular components.
Mechanisms of Separation
The mechanisms of separation facilitated by isoamyl alcohol involve its unique capacity to create a more distinct phase boundary between the organic and aqueous layers. This characteristic is particularly beneficial as it reduces the risk of contamination from proteins and other nucleic acids. The presence of isoamyl alcohol leads to a more efficient stratification of phases, allowing for a more straightforward collection of RNA.
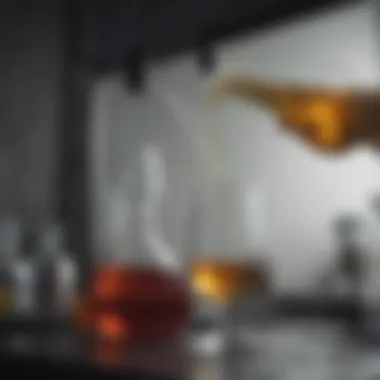
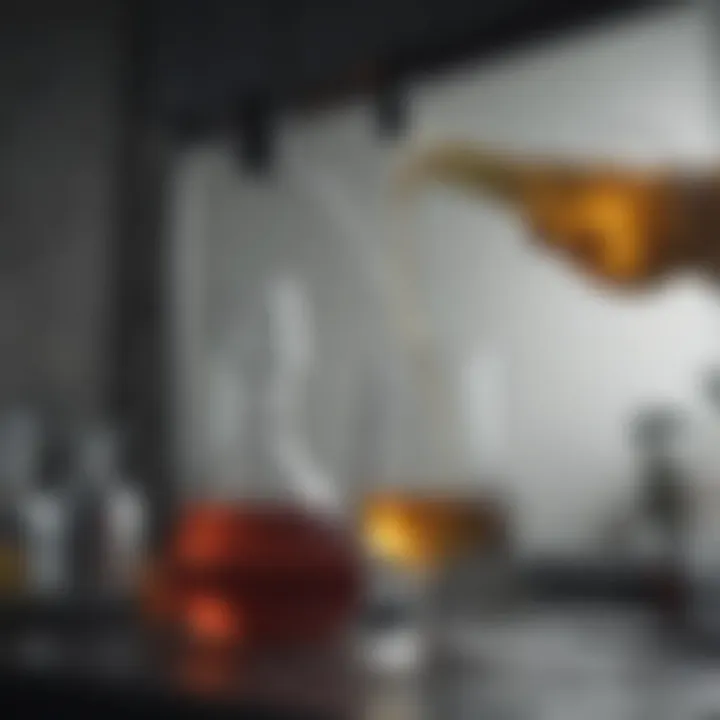
This clear demarcation is advantageous because it reduces the likelihood of carryover contamination from the organic layer into the RNA fraction.
Facilitating Phase Separation
Isoamyl alcohol plays a crucial role in facilitating phase separation during the extraction process. Its hydrophobic nature allows it to interact primarily with the organic layer during centrifugation. This effective separation enhances the integrity of the RNA being extracted.
One unique feature of isoamyl alcohol is its ability to decrease the viscosity of the organic phase. This decrease is significant as it promotes smoother phase transitions, making it easier to separate the aqueous RNA solution from contaminants.
In summary, both chloroform and isoamyl alcohol are vital for RNA extraction. Their chemical properties and the mechanisms they enable allow for more efficient and cleaner RNA extractions, supporting subsequent molecular biology applications effectively.
The Protocol: Steps for RNA Extraction Using Chloroform and Isoamyl Alcohol
The protocol for extracting RNA using chloroform and isopropyl alcohol is pivotal in molecular biology. This method provides a reliable means of isolating RNA from various biological samples, essential for subsequent analyses such as gene expression studies. Properly following this protocol can significantly affect the yield and quality of RNA, thus impacting downstream applications. Therefore, understanding the detailed steps in the protocol is crucial for achieving optimal results.
Sample Preparation
Selection of Biological Samples
The choice of biological samples for RNA extraction is critical. Samples can include tissues, cells, or even blood. The selection significantly influences the extraction process and the integrity of the final RNA. For instance, liver and muscle tissue often provide high yields, while plant samples can present challenges due to cell wall rigidity.
A key characteristic of selecting biological samples is the RNA quality, which can vary depending on the source. Samples like cultured cells are popular because they offer robustness. However, these choices may limit the diversity of downstream applications.
Another unique aspect to consider is how fresh versus frozen samples can affect RNA yield. Fresh samples generally provide superior quality RNA, but this necessitates rapid processing.
Cell Lysis Techniques
Cell lysis is a crucial step in the RNA extraction process. It allows access to RNA by breaking down cellular structures. Common techniques include using detergents or mechanical methods.
The effectiveness of these techniques can greatly influence the efficiency of RNA recovery.
One key characteristic is that chemical lysis methods, while effective, can sometimes introduce contaminants that complicate downstream applications. Conversely, mechanical methods may not be as effective in homogenizing tough samples but can be cleaner.
The unique feature here is the choice between these methods. Chemical methods require careful handling and timing to prevent RNA degradation, while mechanical methods allow for immediate processing and reduced contamination risk.
Adding Chloroform and Isoamyl Alcohol
Proportions of Reagents
The correct proportions of chloroform and isoamyl alcohol are vital for optimal phase separation. Typically, a ratio of 1:1 for chloroform to the aqueous solution is recommended, while isoamyl alcohol is often added at about 24 µL per 1 mL of aqueous solution.
This ratio is significant because it influences RNA solubility. An unbalanced ratio can result in poor recovery rates or contamination. Keeping reagent volumes accurate is a beneficial practice that ensures reliable and reproducible results.
Moreover, the unique feature of using these reagents in specified amounts is their ability to enhance phase separation during centrifugation, ensuring that RNA remains in the upper aqueous phase.
Mixing Protocols
Well-defined mixing protocols are essential following the addition of chloroform and isoamyl alcohol. Gentle mixing is required to avoid shearing the RNA, which can lead to fragmentation.
A typical approach is gentle inversion rather than vigorous shaking. This attention to how mixing is done can significantly affect the integrity of the RNA.
The unique aspect here is the balance between adequate mixing and the prevention of RNA damage. If mixed too vigorously, the recovered RNA may be of lower quality, impacting subsequent analysis in significant ways.
Centrifugation Process
Centrifuge Settings
Centrifuge settings play a substantial role in the RNA extraction process. The speed and duration of centrifugation need to be optimized for effective separation of phases. Generally, a speed of around 12,000 to 15,000 x g for 15 to 20 minutes is recommended.
Key characteristics include the rotor type and temperature settings, both of which can influence the performance of the process. By adhering to these settings, you ensure efficient separation of the RNA-containing upper phase from the lower organic phase.
The unique feature is that improper settings can lead to incomplete phase separation, thus reducing RNA yield and quality.
Separation of Phases
The separation of phases is a critical consequence of effective centrifugation. After centrifugation, three distinct layers emerge: the lower organic phase, the interphase, and the upper aqueous phase containing RNA.
Recognizing this separation is fundamental, as it allows for the careful retrieval of RNA from the upper phase without contamination from other components. A beneficial practice is to carefully pipette off the upper phase to maintain RNA integrity.
The unique aspect of this step is how gently handling the separated phases can significantly influence the successful extraction and purification of RNA for future analysis.
RNA Precipitation
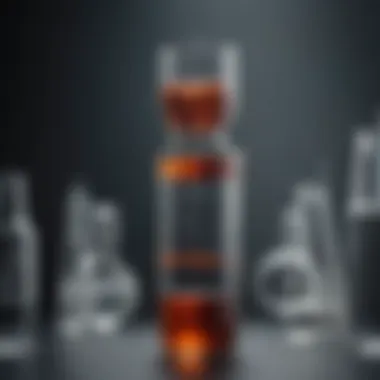
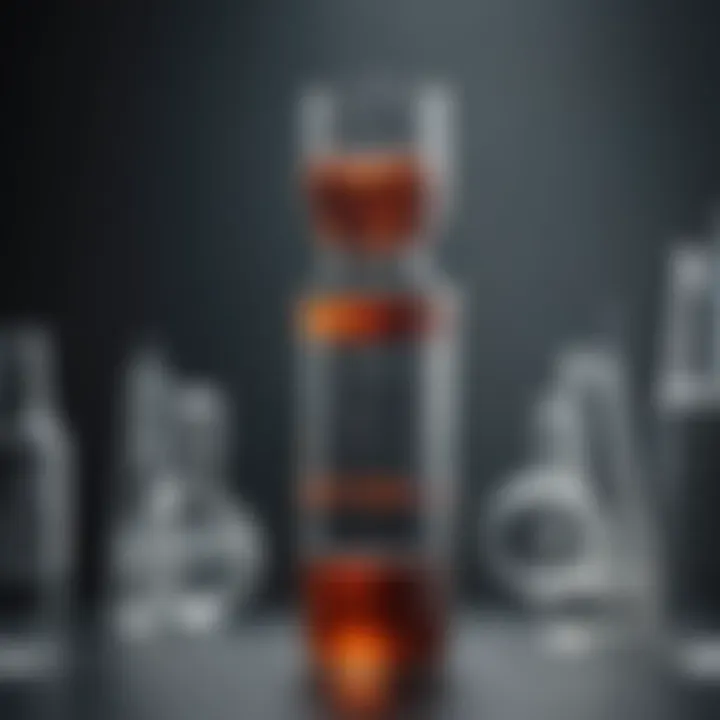
Use of Ethanol or Isopropanol
In RNA precipitation, alcohol plays a pivotal role. Ethanol or isopropanol is added to facilitate the nucleic acid precipitation process. The typical recommendation is to use 2-3 volumes of cold ethanol or isopropanol relative to the aqueous phase.
The effectiveness of this step largely depends on the type of alcohol used. Ethanol is widely preferred due to its efficient co-precipitation of impurities along with RNA, ensuring a cleaner final product.
Another unique feature is that while both alcohols are effective, isopropanol can lead to lower yields in some cases due to stronger binding, which can complicate the washing steps.
Incubation Conditions
Incubation conditions following the addition of alcohol are vital. Typically, samples are incubated at -20 degrees Celsius for at least one hour. This chilling enhances precipitation.
Key characteristics include ensuring that the samples are adequately chilled, which enhances sedimentation of RNA during centrifugation. If incubation times are inconsistent, RNA yield may vary, complicating analysis.
The unique aspect of these incubation conditions is the balance of time and temperature that allows optimal RNA recovery while preventing degradation.
Washing and Resuspension
Washing Steps
Efficient washing steps are crucial for purifying RNA. Typically, a wash solution such as 70% ethanol is used to wash the RNA pellet post-precipitation. This step helps to remove residual salts and contaminants.
The key characteristic is that thorough washing significantly improves the purity of the RNA, ensuring that it is suitable for downstream applications. The washing process also decreases the overall amount of variable contaminants in the final product.
The unique aspect here is how the washing steps must be handled delicately to prevent dislodging the RNA pellet, which can ultimately affect yield and quality.
Optimal Resuspension Solutions
Finally, the choice of resuspension solution is critical for maintaining RNA stability. Common practices involve using RNase-free water or specific buffer solutions. The goal is to preserve RNA integrity for future experiments.
Key characteristics include the pH and ionic strength of the resuspension solution, both of which can impact RNA stability and usability.
A unique observation is that using water may lead to RNA degradation over time compared to buffer solutions specifically designed for RNA storage, further underlining the importance of this decision.
Troubleshooting Common Issues
In any scientific procedure, especially one as delicate as RNA extraction, issues may arise that can hinder progress. Addressing these problems effectively is crucial for ensuring successful outcomes. This section delves into common issues encountered during the chloroform-isopropyl alcohol method for RNA extraction.
Low RNA Yield
One of the significant challenges in RNA extraction is obtaining a low yield of RNA.
Potential Causes
Low RNA yield can be attributed to various factors. One common factor is suboptimal cell lysis. If the biological sample is not thoroughly lysed, this will lead to incomplete extraction of RNA from the cells. Inadequate mixing of the reagents can also result in inefficient phase separation, leading to loss of RNA in the organic phase. Another potential cause could be the quality of the reagents used. If the chloroform or isoamyl alcohol is degraded or contaminated, this may further impact yield. Understanding these causes is critical for labs aiming to achieve reliable and reproducible results. Each source of low yield can significantly affect overall RNA isolation efficiency, underscoring the need for diligence in following protocols.
Remedial Strategies
To mitigate low RNA yield, it is essential to consider several remedial strategies. Firstly, optimization of cell lysis techniques can enhance the extraction efficiency. Using mechanical disruption methods may facilitate a more thorough breakdown of cells. Additionally, careful adherence to mixing protocols ensures an even distribution of reagents, contributing to efficient phase separation. Monitoring the quality of reagents is also important; using freshly prepared or validated reagents can reduce the chances of degradation. These strategies can lead to improved RNA recovery and overall satisfaction with the extraction process.
RNA Degradation Challenges
RNA degradation presents another significant hurdle post-extraction.
Identification of RNase Contamination
RNase contamination can severely compromise the integrity of RNA samples. Such contamination may often come from the environment or handling procedures. Identifying the presence of RNases is crucial because they can degrade RNA quickly, leading to unusable samples. Frequent checks for RNase presence through control experiments can help maintain the integrity of samples. Being aware of this risk is paramount, as even minute amounts of RNases can lead to substantial RNA degradation.
Improving Handling Techniques
To combat RNase degradation, employing improved handling techniques is vital. A basic yet effective strategy includes wearing gloves and using RNase-free tools and reagents. Avoiding common contamination sources, such as unclean surfaces and improper disposal of biological samples, can minimize risks further. Additionally, maintaining samples on ice during extraction can slow down any enzymatic activity present. These handling protocols ultimately safeguard RNA integrity, resulting in higher-quality samples and reliable downstream applications.
Implications for Downstream Applications
Understanding the implications for downstream applications is crucial for researchers using the chloroform-isopropyl alcohol method for RNA extraction. The extracted RNA quality directly influences the outcomes of subsequent experiments, making it necessary to assess its purity and integrity.
Quality Assessment of Extracted RNA
Spectrophotometric Analysis
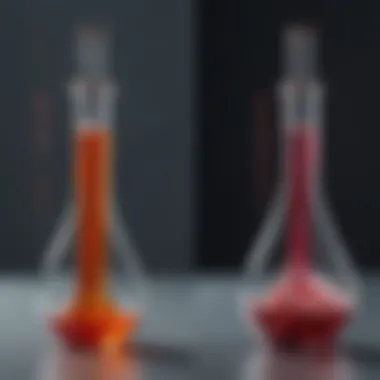
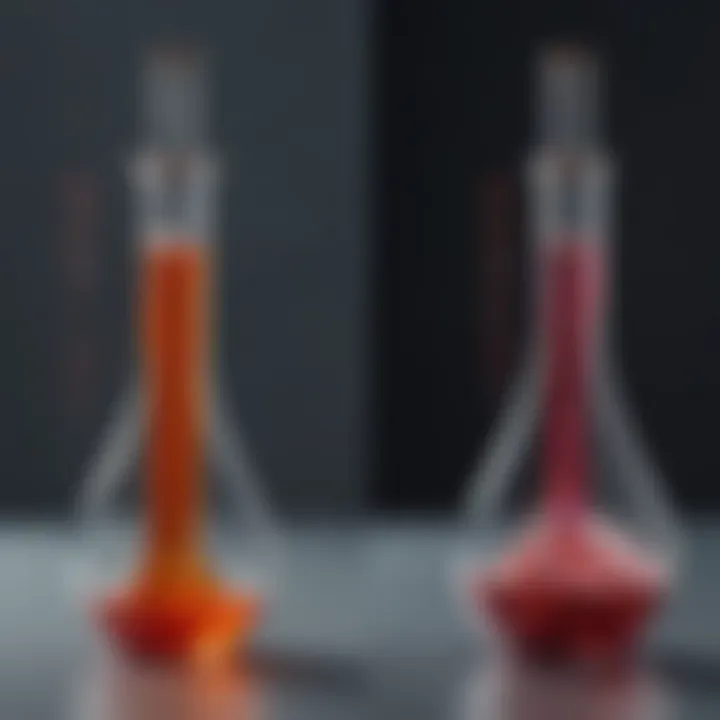
Spectrophotometric analysis provides a reliable method to assess RNA quality. By measuring the absorbance at specific wavelengths, researchers can determine RNA concentration and purity. A common practice is to measure absorbance at 260 nm and 280 nm to calculate the A260/A280 ratio. A ratio close to 2.0 indicates pure RNA, while lower values suggest protein contamination. This method is particularly beneficial due to its simplicity and speed.
The unique feature of spectrophotometry is its non-destructive nature. This allows researchers to obtain multiple readings from a single sample without compromising its integrity. However, it has limitations; it does not provide information about RNA fragment sizes or specific degradation patterns, which usually are also essential for downstream applications.
Agarose Gel Electrophoresis
Agarose gel electrophoresis is another pivotal technique used to evaluate the integrity of extracted RNA. This method separates RNA fragments based on size, allowing researchers to visualize the ribosomal RNA bands. The presence of intact 28S and 18S bands is a clear indicator of high-quality RNA. Agarose gel electrophoresis is admired for its sensitivity, being capable of detecting even minor levels of degradation.
One advantage of this technique is its ability to show RNA integrity in a straightforward visual format. However, it requires more time and sample volume compared to spectrophotometric analysis. Moreover, special equipment and reagents are needed, which may pose challenges for some laboratories.
Applications in Gene Expression Studies
qPCR Techniques
Quantitative PCR (qPCR) techniques are widely utilized for gene expression analysis. This method quantitatively measures RNA by converting it to complementary DNA (cDNA) and amplifying it. The sensitivity of qPCR allows for detection of low-abundance transcripts, making it essential for precise analysis in many experiments. Its high-throughput nature enables researchers to analyze multiple samples efficiently.
The essential characteristic of qPCR is its quantitative capability. This distinguishes it from traditional PCR and makes it a preferable choice for studies involving gene expression. The unique feature of real-time data collection allows researchers to analyze the results as they occur. Some disadvantages include the potential for contamination and the need for specific primers, which can complicate the process.
RNA Sequencing
RNA sequencing (RNA-seq) represents a powerful approach for capturing a comprehensive view of the transcriptome. This method allows researchers to examine and quantify RNA in a highly detailed manner. It unveils the complexity of gene expression profiles, including alternative splicing events and non-coding RNA contributions.
One key advantage of RNA-seq is its ability to provide vast amounts of data, revealing insights into rare transcripts that other methods may overlook. Its unique feature is the capability to detect novel transcripts and gene fusions. However, the process can be resource-intensive, with higher costs and extensive bioinformatics support needed for data analysis.
In summary, the implications for downstream applications highlight the importance of RNA integrity, allowing researchers to harness the potential of various molecular biology techniques effectively.
Comparison with Alternative RNA Extraction Methods
In the pursuit of extracting high-quality RNA, various methodologies have emerged, each with its distinct advantages and limitations. The comparison of the chloroform-isopropyl alcohol method to alternative RNA extraction techniques is significant for researchers who must choose the most suitable approach for their specific needs.
The importance of understanding these comparisons lies in the diversity of biological samples and the differing requirements for downstream applications. Each RNA extraction method may deliver varying quantities and purity of RNA, which directly affects the results of gene expression analysis, sequencing, or other investigative procedures. Thus, selecting the right method influences the reliability of experimental outcomes.
Silica Column Methods
Silica column techniques have gained popularity due to their simplicity and effectiveness. These methods typically use a silica-based column which binds RNA in the presence of chaotropic salts. The process involves lysing the cells, passing the lysate through the column, and subsequently washing and eluting the RNA with an appropriate buffer.
Benefits of Silica Column Methods:
- User-friendly Protocol: Researchers often find silica column extractions straightforward, making them accessible to various skill levels.
- Quick Processing Time: Generally, the entire RNA extraction can be completed in about 30 minutes.
- Good RNA Yield: Silica methods often yield a decent amount of RNA suitable for downstream applications.
However, there are considerations to keep in mind:
- Cost: They may be more expensive than chloroform-isopropyl alcohol extraction, especially when multiple samples need processing.
- Loss of RNA: Some RNA, particularly small RNAs, can be lost during the washing steps.
Magnetic Bead Techniques
Magnetic bead-based methods represent another alternative for RNA extraction. This technique employs magnetic beads coated with materials designed to capture RNA. After mixing cells with these beads, a magnet is used to isolate the beads from the solution, thereby capturing the RNA.
Benefits of Magnetic Bead Techniques:
- High Sensitivity and Purity: They generally offer high sensitivity in extracting RNA, even from low-abundance targets.
- Flexibility in Applications: Suitable for various sample types, including those that are difficult to lyse effectively.
- Automation-Friendly: Magnetic bead extraction can easily be adapted for high-throughput systems, making it attractive for larger experiments.
On the downside, researchers must consider:
- Longer Protocol Times: Compared to silica columns, bead extraction protocols may require more time, impacting overall efficiency.
- Complexity of Workflow: The handling and storage of magnetic beads can complicate experimental setups for less experienced researchers.
The choice of RNA extraction method can significantly influence the quality of obtained RNA and thus affect the validity of subsequent research.
Finale and Future Directions
The importance of the chloroform-isopropyl alcohol method in the context of RNA extraction cannot be overstated. This technique offers a reliable means of isolating RNA from various biological samples. Its efficiency stems from the careful balance of chemical properties and the straightforward protocol that it employs. As researchers, understanding these elements is essential.
In summary, this article highlights the critical steps and considerations necessary for successful RNA extraction using chloroform and isopropyl alcohol. Well-executed protocols can lead to high-quality RNA, which is integral for downstream applications critical to molecular biology, genomics, and transcriptomics.
Summary of Key Findings
- The chloroform-isopropyl alcohol method allows for effective separation of RNA from DNA and proteins, crucial for obtaining pure RNA.
- Sample preparation and handling techniques play a pivotal role in the yield and quality of extracted RNA. Different biological samples require tailored lysis methods for optimal results.
- Troubleshooting common issues, such as low yield or contamination, is essential. Specific strategies can mitigate these challenges to enhance reliability in RNA retrieval.
- Quality assessment methods, such as spectrophotometric analysis and agarose gel electrophoresis, provide validation for the success of the extraction process.
Potential Innovations in RNA Extraction
As science progresses, the field of RNA extraction continues to evolve. Potential innovations include:
- Automated Extraction Systems: Advances in laboratory automation can streamline the RNA extraction process, reducing human error and increasing throughput.
- Green Chemistry Approaches: Developing environmentally friendly alternatives to chloroform could minimize hazardous waste while maintaining extraction efficiency.
- Microfluidics: Utilizing microfluidic technologies can enhance control over reagent interactions, leading to finer separation and improved yields.
- CRISPR-based Methods: Integrating CRISPR technology may offer ways to selectively isolate specific RNA types, further enhancing the versatility of extraction methods.
These innovations hold promise for improving RNA extraction protocols and, by extension, the quality and quantity of data produced in molecular research. As these techniques advance, they will likely offer more accessible, efficient, and safe methods for researchers worldwide.