Exploring Devices for Radiation Measurement and Safety
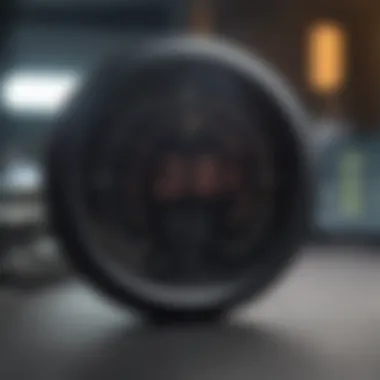
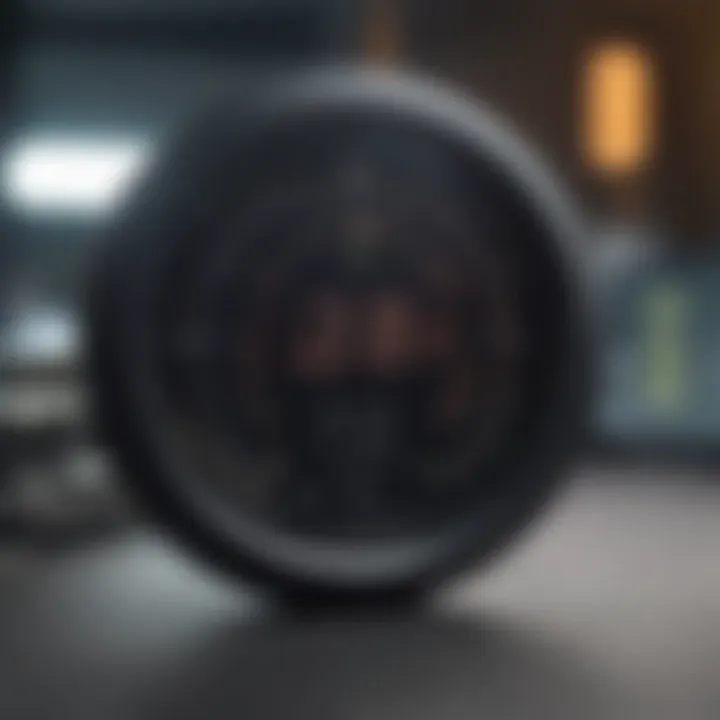
Intro
Radiation exposure is an ever-present concern in numerous fields, from healthcare to environmental monitoring. Understanding the levels of radiation we encounter daily is no small feat. The right devices can make all the difference in ensuring safety and compliance with regulations. Grasping how these devices work is vital for students, researchers, educators, and professionals alike. This article aims to dissect the mechanisms of various radiation measuring devices, laying out their practical applications and significance.
Research Overview
Methodological Approaches
An exploration into radiation measurement begins with the understanding of methodologies used to gather data. The most prominent tools in this arena include dosimeters and Geiger counters.
Dosimeters are personal devices often used by workers in occupations involving radiation exposure. These devices usually employ special materials that react to radiation, changing their properties based on exposure levels. Researchers measure these changes to quantify radiation levels over time.
On the other hand, Geiger counters work by detecting the ionization of gas. When radiation passes through the detector, it causes atoms in the gas to ionize, leading to measurable clicks or readings. This real-time feedback is invaluable for immediate safety assessments in various settings.
Significance and Implications
The importance of accurate radiation measurement cannot be overstated. With health risks associated with excessive radiation exposure, understanding the implications is crucial.
- Occupational Safety: In industries such as nuclear energy or healthcare, monitoring radiation is essential for worker safety.
- Environmental Monitoring: Devices play a critical role in ensuring that ecosystems remain within safe exposure limits.
- Healthcare Applications: In medical scenarios, devices help monitor radiation levels during treatments, such as radiation therapy, ensuring both patient and staff safety.
Radiation measuring devices not only boost individual safety but also enhance regulatory compliance and public trust in industries that handle radiation.
Current Trends in Science
Innovative Techniques and Tools
Innovation is the lifeblood of advancement. Recent years have seen substantial improvements in the design and functionality of radiation measuring tools. For instance, today’s digital dosimeters offer features like data logging and real-time alerts, providing invaluable information at the touch of a button.
Furthermore, smart devices integrated with apps allow users to track exposure levels over time, making safety a more interactive and manageable task.
Interdisciplinary Connections
The intersection of fields such as physics, engineering, and health sciences has led to significant advancements in the development of these devices. Collaborations between engineers and healthcare professionals fuel innovations designed to meet the unique needs of different sectors. For example, research initiatives combining materials science with radiation safety have led to the creation of new types of dosimeter materials that are far more sensitive than their predecessors.
"As technology evolves, so must our understanding of radiation measurement, balancing safety with the need for advancement."
Understanding Radiation Exposure
Grasping the concept of radiation exposure is crucial for anyone working in environments where radiation is present, or even for those engaged in scientific research. To make informed decisions regarding health and safety, it's not just about knowing the existence of radiation; understanding its characteristics and effects represents the foundation of safe practice.
Define Radiation and Its Types
Radiation can be thought of as energy that travels through space. It comes in different forms which can be broadly categorized into two groups: ionizing and non-ionizing radiation. Ionizing radiation possesses enough energy to remove tightly bound electrons from atoms, thus creating ions. This can profoundly influence biological tissues and lead to damage at a cellular level. Common examples include alpha particles, beta particles, gamma rays, and X-rays. Non-ionizing radiation, on the other hand, consists of lower energy forms such as radio waves, microwaves, and visible light. While non-ionizing radiation is typically less harmful, it's still essential to measure exposure correctly, especially in research and industrial settings.
Impacts of Radiation on Health
The health impacts of radiation exposure can be quite significant. Short-term exposure to high levels of ionizing radiation can lead to acute radiation syndrome, a condition characterized by nausea, vomiting, and in severe cases, death. Long-term exposure, even to smaller doses, can increase the risk of cancer and damage to organs. Certain research studies even suggest that prolonged exposure may lead to cardiovascular diseases. Understanding these health implications is of utmost importance for developing effective safety protocols. Individuals who work in nuclear power plants or hospitals equipped with radiology departments might find themselves on the front lines of radiation exposure incidents. Consequently, they must be vigilant in monitoring their exposure levels—it's a matter of life and death, quite literally.
Safety Standards and Guidelines
Navigating the labyrinth of safety standards and guidelines can be daunting. Various organizations have established protocols to ensure safe levels of radiation exposure. The International Commission on Radiological Protection (ICRP) and the U.S. Environmental Protection Agency (EPA) both play pivotal roles in setting these guidelines. They recommend dose limits depending on the type of radiation exposure, categorizing it into occupational and public exposure scenarios. For example, the ICRP suggests that occupational exposure should not exceed 20 mSv per year, while the general public should ideally not be exposed to more than 1 mSv annually from man-made sources.
"Understanding and following these standards is not just regulatory compliance; it’s about safeguarding lives and health."
Keeping abreast of national regulations, as well as any updates from global organizations, is essential for anyone involved in radiation-related activities. It not only helps in reducing risks but also fosters a culture of safety and responsibility.
Being proactive about understanding radiation exposure contributes to a more informed, prepared, and ultimately safer environment for all. Whether through compliance with established safety standards or simply ensuring the personal safety of oneself and colleagues, the significance of this understanding cannot be overstated.
The Role of Measurement Devices
In any discussion surrounding radiation exposure, it becomes paramount to underscore the essential role measurement devices play. These instruments are not mere gadgets; they act as the first line of defense against potential hazards posed by radiation in various environments. With radiation emerging from multiple sources—be it natural or artificial—the need to monitor and quantify this exposure cannot be overstated.
The importance of monitoring radiation exposure extends into numerous sectors. In medical settings, where radiation is a prevalent tool for diagnosis and treatment, these devices ensure both patient and practitioner safety. In industrial scenarios, accurate readings support compliance with regulations and safety standards, as numerous manufacturing processes involve radioactive materials. Meanwhile, in environmental contexts, ongoing assessment loses no relevance, especially in sites where nuclear activity or accidents might have occurred.
Measurement devices provide numerous benefits:
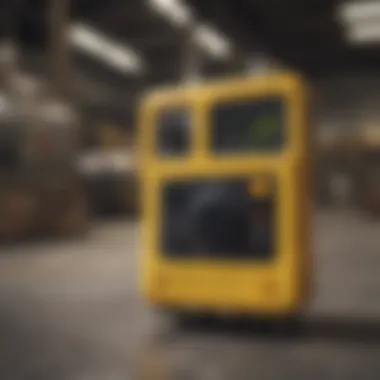
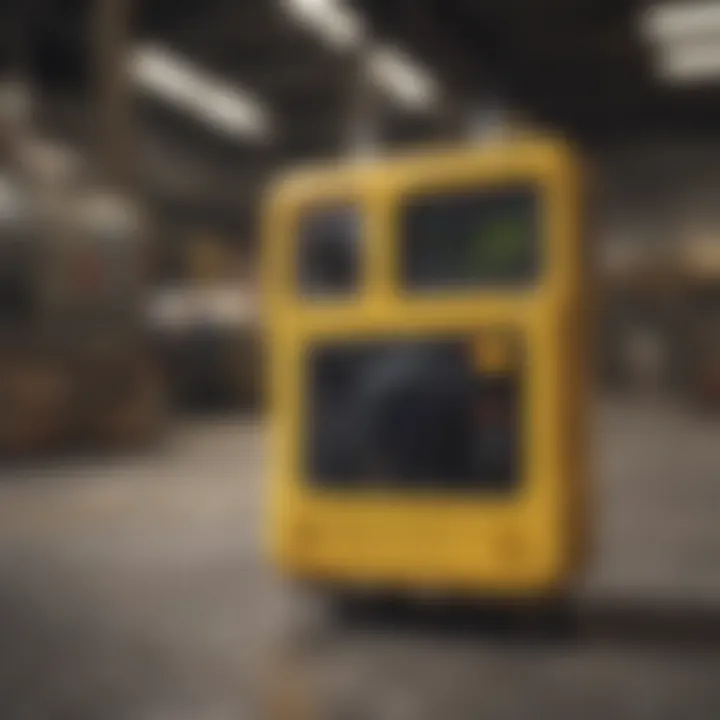
- Health Protection: They help mitigate health risks associated with excessive radiation exposure.
- Regulatory Compliance: Many industries are bound by laws that require continuous monitoring of radiation levels. Measurement devices facilitate adherence to these standards.
- Research and Understanding: Accurate readings contribute to the body of research on radiation effects, improving overall knowledge and safety protocols.
Ultimately, measurement devices not only enhance safety but also foster a culture of awareness regarding radiation's implications in our lives. The details surrounding these devices can greatly affect decision-making in occupations and personal scenarios alike.
Importance of Monitoring Radiation Exposure
Monitoring radiation exposure involves tracking gamma rays, alpha and beta particles emitted from various radiation sources. Without monitoring, workers and the public remain at risk, often without adequate knowledge of surrounding radiation levels.
In healthcare, for example, effective and routine monitoring of radiation exposure for professionals can lower the risk of harmful effects over time. Nurses, radiologists, and technicians engaging with X-rays and other isotopes benefit profoundly from using dosimeters, which record their exposure. This ensures they don’t exceed recommended limits.
In industries like nuclear power, monitoring isn't just about employee safety; it's also crucial for environmental stewardship. When radiation is released into the atmosphere, discrepancies can lead to serious consequences, including contamination that could linger for decades. Regular exposure monitoring systems ensure proactive measures are taken before situations become critical.
Overview of Measurement Technologies
A variety of technologies exist for measuring radiation exposure, each suited for different applications and levels of detection. This diversity in measurement offers flexibility and specificity when selecting the right tool for the task at hand.
- Dosimeters: These are personal devices worn to quantify exposure over time. They can provide historical data on exposure levels, giving a comprehensive view of an individual's radiation experience.
- Geiger Counters: Known for their audible clicks, these handheld devices offer real-time readings and are commonly used in fieldwork. Their portability and ease of use make them a favorite among safety officers.
- Ionization Chambers: These devices detect radiation by measuring the number of ion pairs created in a gas-filled chamber. They are particularly effective for high-radiation environments.
- Scintillation Detectors: Utilizing phosphors to detect radiation, these devices boast exceptional sensitivity and are often used in laboratories and security applications.
- Radiation Spectrometers: These sophisticated tools analyze the energy of the radiation making them valuable for research and nuclear security applications.
"Accurate and consistent radiation measurement can mean the difference between safety and danger in many critical fields."
Each technology serves a unique purpose and adds a layer of safety in its respective field. With ongoing advancements, the accuracy and reliability of these devices continue to improve, further solidifying their necessity in our efforts to manage and understand radiation exposure.
Types of Radiation Detection Devices
Understanding the various types of radiation detection devices is crucial for anyone involved in the fields of health physics, environmental monitoring, or industrial safety. These devices not only help in tracking radiation levels but also play a significant role in ensuring safety protocols are followed effectively. Different detection devices come with unique mechanisms, each designed for specific applications and settings. An awareness of these tools underscores their importance in protecting individuals and the environment from potential hazards associated with radiation exposure.
Dosimeters: Types and Uses
Dosimeters serve a vital function by measuring accumulated radiation exposure over time. They are indispensable in areas where workers are routinely exposed to radiation, such as hospitals and nuclear facilities.
- Types of Dosimeters:
- Thermoluminescent Dosimeters (TLDs): These utilize materials that emit light when heated, thus indicating the amount of radiation exposure. They are known for their accuracy and can be reused after being read.
- Optically Stimulated Luminescence (OSL) Dosimeters: Similar to TLDs, these dosimeters use aluminum oxide to measure radiation exposure, which can be stimulated by light rather than heat for readout, allowing for more versatile uses.
- Electronic Dosimeters: These provide real-time data on radiation exposure, making them popular for immediate feedback. They can alert users when exposure exceeds preset levels.
Choices among these types often depend on the specific occupational hazards present and the required precision of the readings. In settings where safety is paramount, such as during radiation therapy, dosimeters ensure that exposure limits are not exceeded, safeguarding both patients and staff.
Geiger Counters: Mechanism and Applications
Geiger counters are perhaps the most well-known radiation detection device, often recognized for their characteristic ticking sound that indicates the presence of ionizing radiation. The mechanism behind a Geiger counter involves a gas-filled tube that conducts electricity momentarily when ionizing radiation passes through it. The device then creates audible clicks or visual signals for each detected particle or photon.
Applications:
- Environmental Monitoring: Used to assess background radiation levels in various locations, particularly those near nuclear plants or other sources of radiation.
- Industrial uses: Employed to ensure proper safety measures in industries that deal with radioactive materials.
- Public education: These devices frequently end up in educational kits, helping people understand radiation in practical terms.
While they provide a good general sense of radiation levels, it's worth noting that Geiger counters may not give precise measurements of the specific types of radiation or their energy levels.
Ionization Chambers: Functionality and Usage
Ionization chambers are sophisticated instruments that measure the charge created by ionizing radiation. Commissioned in laboratories and hospitals, they offer a different approach compared to other detection devices.
Functionality:
The ionization chamber consists of a sealed enclosure filled with gas. When radiation passes through it, ionization occurs, producing charge carriers. The resulting current is proportional to the radiation dose.
Usage:
- Radiation therapy: Integral in measuring doses delivered to cancer patients during treatment sessions.
- Radiation protection: Used for compliance testing in environments where radiation exposure is a concern.
- Calibration of other devices: Ionization chambers serve as reference standards for calibrating other types of radiation measuring instruments.
Scintillation Detectors: Principles of Operation
Scintillation detectors harness the property of certain materials to emit light when exposed to radiation. The emitted light is then converted into an electrical signal for measurement.
Principles of Operation:
When ionizing radiation interacts with the scintillating material, it excites the atoms, leading to the release of photons. A photo-multiplier tube can be employed to amplify these light signals for analysis.
Applications:
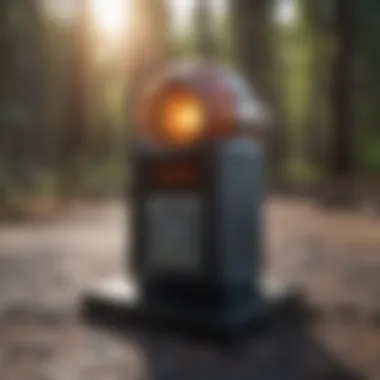
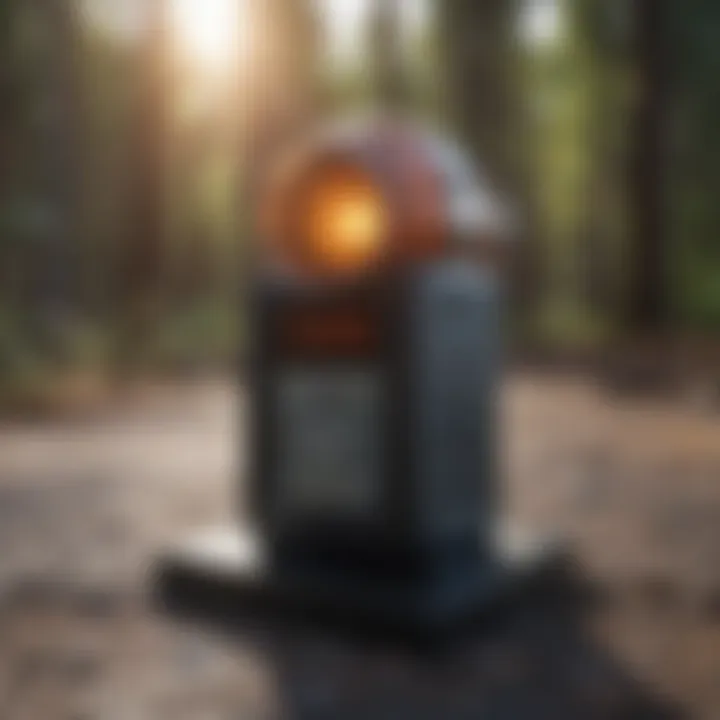
- Medical Imaging: Integral in positron emission tomography (PET) and certain types of nuclear medicine scans.
- Quality assurance: Used in laboratories to assess radiation of samples, ensuring accurate readings.
- Radiological emergencies: Effective in identifying the presence of radioactive contamination post-incident.
Radiation Spectrometers: A Comprehensive Overview
Radiation spectrometers go beyond simple detection by analyzing the energy levels of the detected radiation. This characteristic makes them particularly valuable in research and safety applications. They consist of various configurations, including semiconductor detectors and scintillation spectrometers, each offering distinct advantages.
Why they matter:
Understanding the specific energy of radiation can inform decisions regarding safety protocols and potential health risks. For example, distinguishing between alpha, beta, and gamma radiation can guide remediation efforts in contaminated areas.
Key Applications:
- Research Institutions: Used in particle physics and astrophysics to identify materials or measure isotopic concentrations.
- Nuclear Industry: Ensures compliance with safety regulations regarding radioactive waste.
- Environmental Studies: Essential for tracking radioactive pollutants and studying their effects in ecosystems.
Comprehension of these devices is paramount not just for professionals working directly with radiation, but also for anyone involved in safety management, environmental research, and public health.
Applications Across Various Fields
Measuring radiation exposure plays a crucial role across various realms, including medical, industrial, and environmental sectors. The importance of these applications cannot be overstated, as they contribute significantly to health, safety, and regulatory compliance. Monitoring radiation not only protects individuals from harmful effects but also ensures that processes meet rigorous safety standards. This exploration provides insight into how measurement devices are utilized in both everyday and emergency scenarios, enhancing our ability to manage and mitigate the impacts of radiation.
Medical Applications of Radiation Measurement
Radiation Therapy Monitoring
Radiation therapy monitoring is vital in ensuring effective cancer treatment while minimizing harm to surrounding healthy tissues. The precise measurement of radiation dosage delivered to the patient is quintessential in achieving the treatment goals. This aspect of monitoring is particularly attractive due to its direct impact on patient outcomes; appropriate dosage can pivot a treatment from failure to success. One key characteristic of radiation therapy monitoring is its ability to adapt to varied patient anatomies and treatment plans, making it a beneficial choice in modern oncological therapy. A unique feature of this monitoring is the incorporation of advanced dosimetry systems that utilize real-time feedback, thus allowing adjustments during treatment sessions. However, the constant need for calibration can introduce complexities in clinical settings.
Nuclear Medicine
Nuclear medicine represents an impressive intersection of technology and healthcare wherein radiation plays a diagnostic and therapeutic role. The field relies on precise measurements to ensure accurate imaging and effective treatment delivery, particularly with radiopharmaceuticals. One of its core strengths lies in the non-invasive nature of nuclear imaging, which allows physicians to visualize and assess organ function without extensive surgery. A distinguishing feature of nuclear medicine is its use of radiotracers, which illuminate abnormalities within the body’s systems, providing unparalleled insights. Despite its advantages, there are inherent risks associated with radiation exposure to both the patient and the medical staff, meaning stringent safety protocols are essential.
Radiology Safety
Radiology safety is an echo of medical ethics, underscoring the commitment of practitioners to safeguard both patients and staff from unnecessary radiation exposure. This aspect involves strict measures like shielding, distance, and time management to reduce risk. What sets radiology safety apart is the continuous evolution of guidelines and protocols aimed at minimizing exposure while maximizing diagnostic quality. For instance, advanced equipment now often includes built-in radiation dose management systems. This feature provides real-time data monitoring, enabling immediate adjustments to optimize safety. Although the technology promises substantial benefits, achieving compliance with consistently evolving safety standards can be challenging.
Industrial Use of Radiation Detecting Devices
Quality Control in Manufacturing
Quality control in manufacturing often incorporates radiation detection as a standard practice, particularly in industries involving materials that emit radiation or can be altered by it. The key characteristic here is the application of non-destructive testing methods that use radiation to inspect the integrity of materials and products. This practice is beneficial as it preserves the usability of the items tested. A unique feature of this application is its ability to provide immediate feedback, allowing for swift corrections in production processes. Nonetheless, the costs associated with integrating such technology can be a barrier for smaller manufacturers.
Radiation Safety in Construction
Radiation safety in construction is another field where monitoring devices are indispensable. Workers may be exposed to natural or artificial radiation, making it necessary to employ detection devices on-site. The key element in this scenario is the early detection of radiation sources, facilitating prompt action to safeguard health. This application is crucial as it helps maintain compliance with occupational safety regulations. Unique to this field is the constant monitoring of varying site conditions, which can change rapidly. The downside, however, is that investing in detection equipment and training can be burdensome for project budgets.
Environmental Monitoring
Environmental monitoring utilizes radiation detection to assess radiation levels in various ecosystems, ensuring public safety and ecological balance. By measuring radioactivity in soil, air, and water, authorities can devise strategies to mitigate contamination and protect human health. The key characteristic of environmental monitoring is its broad scope; it encompasses both natural and anthropogenic radiation sources. This versatility makes it particularly relevant in our current age of ecological awareness. A unique feature lies in its application during regulatory reporting, which helps in compliance with environmental laws. However, the complexity of data interpretation and the need for continuous monitoring can prove challenging for organizations tasked with these responsibilities.
Environmental Monitoring and Research
Assessing Natural Background Radiation
Assessing natural background radiation is fundamental to understanding the baseline radiation levels in different regions. This process contributes to public health knowledge and frameworks for radiation safety. The key characteristic of this assessment is the establishment of reference values which can inform both public policy and individual health decisions. This component of environmental research is particularly valuable as it denotes the normal radiation levels people are exposed to every day. However, unique challenges arise from varying geological and environmental factors that can complicate data collection.
Radioactive Waste Management
Radioactive waste management encompasses the processes utilized to handle and dispose of waste from nuclear facilities and medical applications. Its contribution to safety in the field is crucial, as it prevents accidental exposure to radiation. A significant characteristic of this area is the stringent regulatory frameworks that govern waste handling, underscoring its importance in public safety and environmental protection. A unique aspect is the development of advanced containment technologies that isolate waste from the environment. Unfortunately, these solutions often come with high financial costs and political hurdles which can complicate implementation.
Emergency Response Planning
Emergency response planning associated with radiation involves preparation for potential exposure incidents, whether from nuclear accidents or radiological dispersal devices. The contribution here is clear: it lays the groundwork for immediate and effective action in protecting public health. A key characteristic of emergency planning is the establishment of protocols that facilitate quick mobilization of resources. This realm places considerable emphasis on training and drills, thus enhancing readiness and resilience against radiation emergencies. The unique feature of effective response plans is their adaptability to various scenarios, allowing for tailored responses depending on specific threats. Yet, the constant need for updates and drills can stretch resources and attention within organizations.
Recent Advances in Technology
The field of radiation measurement has seen significant strides in recent years. These advancements are not just fluff—they carry profound implications for safety practices, research accuracy, and the efficiency of radiation monitoring. By integrating cutting-edge materials and smart technology, scientists and engineers have improved how devices detect and quantify radiation. This section will dive into those changes, emphasizing how they reshape our understanding and management of exposure risks.
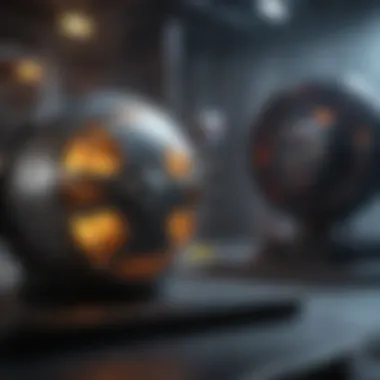
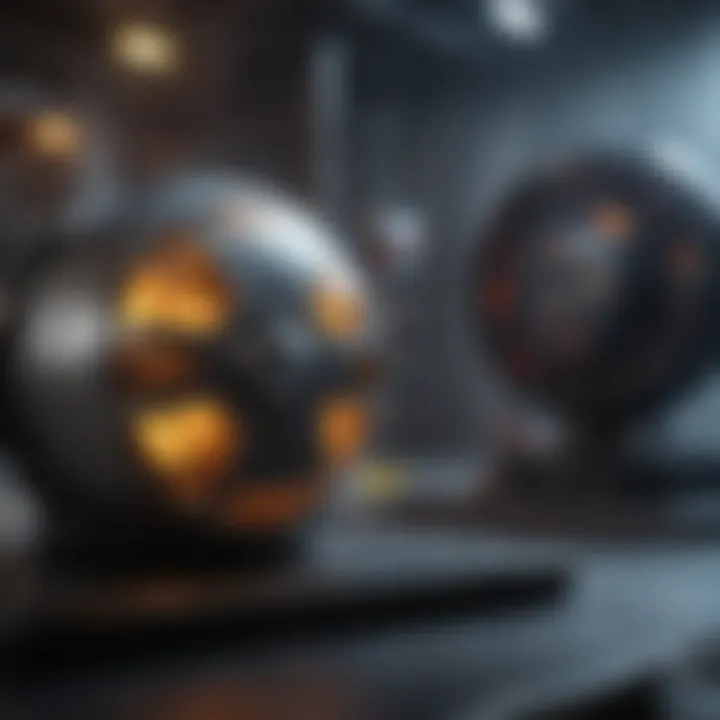
Innovative Materials and Design in Radiation Devices
Every time a new device hits the market, it seems to carry a story of innovation behind it. Recent developments often focus on the use of novel materials that enhance the effectiveness of radiation detection. For instance, silicon-based detectors have gained traction due to their superior sensitivity and speed. Compared to traditional devices, these silicon detectors can offer quicker response times, allowing for more real-time monitoring.
Additionally, the incorporation of nanomaterials has opened up new avenues in design. Graphene, for example, has shown promise in creating ultra-thin detectors that maintain high sensitivity while being lightweight and portable. These advancements mean that professionals can carry their monitoring equipment with ease without compromising performance. Moreover, new designs often integrate ergonomic features that enhance usability for technicians working in demanding environments, promoting both efficiency and safety.
Advances in 3D printing technology also deserve a mention here. Customizable parts can be manufactured rapidly, allowing for the development of devices tailored specifically for certain tasks or environments. This kind of flexibility represents a leap forward compared to the rigid designs of older radiation detectors.
Digital Technology and Data Analysis
The transition towards digital technology has revolutionized how we analyze radiation data as well. Modern radiation devices are often equipped with sensors that are capable of not just measuring exposure levels but also integrating with software that provides real-time data analysis. This synergy allows professionals in the field to make informed decisions quickly.
For example, devices now often have built-in GPS features, which can help track exposure data geographically, an essential factor in contamination assessment during environmental studies. These digital tools can analyze patterns over time, providing insights that would have been painstakingly slow to obtain manually.
Moreover, the implementation of cloud computing offers an expansive platform for data storage and sharing among researchers and institutions globally. The ease with which vast amounts of data can be collected and analyzed encourages collaborative projects that further enhance safety protocols and technological advancements in the field.
"Digital technology is changing how we perceive radiation safety, emphasizing actionable data over mere numbers to inform decision-making and policy."
The marriage of cutting-edge materials and advanced data processing capabilities marks a significant turning point in radiation measurement technology. As these technologies evolve, they are likely to set the pace for future developments, ensuring that safety in radiation-exposed environments remains a top priority.
Maintenance and Calibration of Radiation Devices
Maintaining and calibrating radiation measuring devices is not just an afterthought; it's a linchpin in ensuring the reliability of measurements and, ultimately, safety. In fields where precise radiation data is pivotal, any deviation can have serious implications. Thus, understanding the nitty-gritty of maintenance and calibration is crucial for students, researchers, educators, and professionals alike.
Regular Maintenance Practices
Regular maintenance encompasses a series of practices aimed at ensuring devices remain in optimal condition. Here’s a rundown of essential maintenance tasks:
- Routine Inspections: Examine devices for physical damage or wear. A cracked Geiger counter or a malfunctioning dosimeter can lead to inaccurate readings.
- Cleaning: Ensure components are free from dust and contaminants. The sensors in radiation detectors are sensitive; any obstruction might skew results.
- Battery Checks: Devices often rely on batteries for power. Regular checks can prevent unexpected failures.
- Firmware Updates: If the device involves software, keep it updated to benefit from improvements and fixes that enhance performance.
- Documentation: Keeping a maintenance log helps in tracking the history of the device and can indicate whether patterns of wear are developing, streamlining future maintenance efforts.
Implementing a structured maintenance schedule not only prolongs the life of the device but also boosts confidence in the measurements being taken. It’s about staying one step ahead rather than reacting when something goes wrong.
Importance of Calibration
Calibration, on the flip side, refers to the process of adjusting the accuracy of a measurement device. Without regular calibration, the precision of data can waver dramatically, which could put safety protocols at risk. Here are some important aspects of calibration:
- Accuracy Assurance: Calibration is essential for ensuring that measurements reflect true values. A poorly calibrated device can misinform users about radiation exposure, leading to dangerous situations.
- Regulatory Compliance: Many industries are bound by stringent regulations that require equipment to meet specific calibration standards. Failure to comply can lead to legal repercussions and reputational damage.
- Periodic Intervals: Calibration should be conducted at regular intervals, but the frequency can depend on usage, environmental conditions, and the sensitivity of the device.
- Follow Manufacturer's Guidance: Each device comes with its recommendation for calibration practices. It's imperative to refer to those guidelines to maintain adherence to proper standards.
- Documentation of Calibration: Just like regular maintenance, records of calibration activities help track performance over time and can be crucial during audits or inspections.
A well-calibrated device builds trust. When stakeholders know the equipment delivers reliable results, they can make informed decisions without second-guessing.
Key Insight: Regular maintenance and rigorous calibrationwork hand-in-hand. Together, they ensure that measuring devices for radiation exposure not only function properly but also deliver accurate readings. The right practices can enhance safety across numerous fields, from healthcare to environmental management.
Regulatory Considerations
In the realm of radiation measurement, regulatory considerations play a pivotal role. They help ensure that devices used for measuring radiation exposure are accurate, reliable, and safe for both users and the general public. The significance of this aspect cannot be understated. Compliance with these regulations not only fosters confidence in the measuring devices but also protects health and safety in various operational environments.
The framework set by various international bodies outlines the guidelines that manufacturers and users must follow. Regulatory bodies such as the International Atomic Energy Agency (IAEA) and the United States Nuclear Regulatory Commission (NRC) establish foundational standards that all measuring devices must meet.
Benefits of adhering to regulatory considerations include:
- Reduced Risk: Adhering to global standards minimizes the possibility of mismeasurement, which could lead to unintended exposure to harmful radiation levels.
- Increased Credibility: Instruments that meet regulatory standards often have a recognized validation, providing users, whether in medical, environmental, or industrial fields, with the assurance of performance.
- Uniformity Across Borders: Regulations help standardize practices internationally, enabling consistent operation and measurement protocols across different regions.
Neglecting these considerations can have grave consequences ranging from health risks to financial liabilities for companies. Thus, regulatory considerations are not just legal obligations; they are fundamental to effectively managing radiation safety.
International Standards for Radiation Measurement
International standards serve as the backbone for ensuring that radiation measuring devices function to their optimal capacity. Documents like the ISO 4037 series guide manufacturers on the testing methods, calibration processes, and operational integrity of radiation measuring devices.
Key points in the international regulatory framework include:
- Calibration Procedures: Standards dictate how devices should be calibrated to ensure accuracy.
- Performance Testing: Regular assessments and audits are mandated to verify that devices perform as required.
- Documentation and Reporting: Detailed records must be maintained to monitor device performance over time.
The creation of these international standards brings together various stakeholders—from engineers to policymakers—paving the way for more robust devices that cater to safety across diverse applications.
Compliance and Reporting Requirements
Compliance is more than just a checkbox exercise; it’s a commitment to uphold safety and accuracy in radiation measurement. Organizations must embrace reporting requirements to maintain accountability.
- Routine Checks: Devices are often required to undergo routine checks and validations. This can mean quarterly assessments or annual reviews, depending on the risk level associated with their use.
- Incident Reporting: Any radiation-related incidents involving measurement devices need to be reported to the respective regulatory bodies. This helps maintain a comprehensive database and allows for necessary adjustments in regulations as new information becomes available.
- Training and Procedures: Users of measurement devices must be trained in compliance protocols to ensure they understand not just how to use the equipment but also how to adhere to the regulatory requirements.
"Staying compliant isn’t just about following rules; it’s about safeguarding lives and ensuring technology works as intended."