Techniques in DNA and RNA Extraction: A Comprehensive Overview
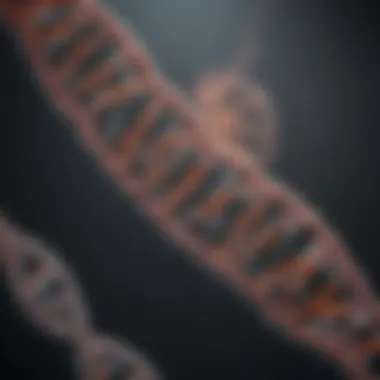
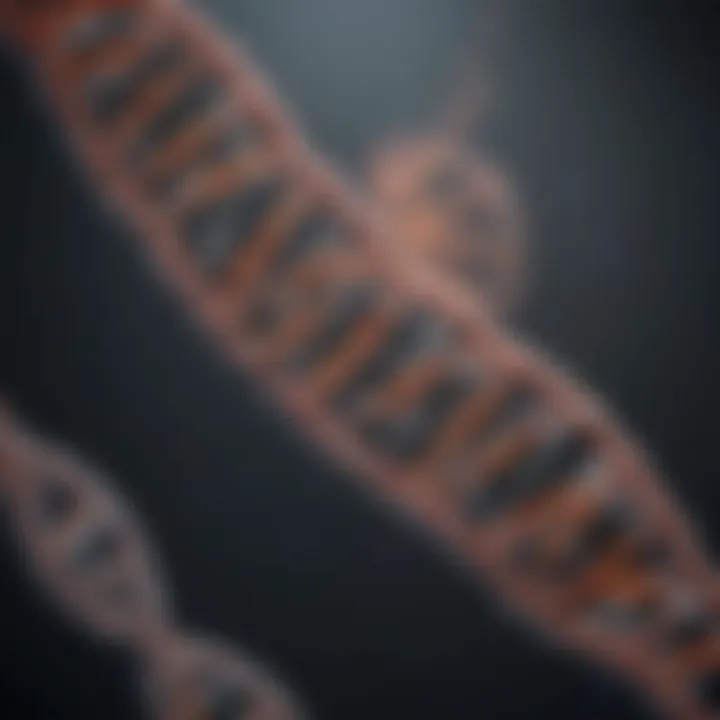
Intro
In the rapidly advancing field of molecular biology, understanding the techniques used in DNA and RNA extraction is essential. These processes are foundational for various applications, ranging from genetic research to forensic analysis. The extraction techniques not only determine the yield and purity of these nucleic acids but also influence the quality of downstream applications such as sequencing and cloning.
Effective extraction methods must be tailored to the specific biological samples involved. Factors such as the type of tissue, the presence of contaminants, and the overall objective of the research play a critical role in method selection. This article aims to provide a comprehensive understanding of the most commonly used techniques, the underlying principles governing these methods, and the ongoing innovations in the field.
Research Overview
Extracting DNA and RNA involves isolating these molecules from cells, which requires a nuanced understanding of biological and chemical principles.
Methodological Approaches
The methodological approaches in nucleic acid extraction can vary significantly based on the sample type and intended analysis. Here are some common strategies:
- Phenol-chloroform extraction: This traditional method uses organic solvents to separate nucleic acids from proteins and other cellular debris. It is known for its effectiveness but can be hazardous and labor-intensive.
- Column-based methods: These involve passing lysates through a silica column, which binds nucleic acids. This technique is favored for its simplicity and speed, making it popular in many labs.
- Magnetic bead-based extraction: This method utilizes magnetic beads coated with nucleic acid-binding materials. This offers a flexible and efficient extraction process, suitable for various sample types.
- Enzymatic digestion: Enzymatic methods utilize specific enzymes to break down cellular components, enhancing the purity of the extracted DNA or RNA. These are particularly useful in samples where polysaccharides or other difficult substances are present.
The choice of method often depends on the balance between yield, purity, and the specific requirements of subsequent applications.
Significance and Implications
Understanding extraction techniques is vital for advancing research in multiple domains:
- Genetics Research: High-quality DNA and RNA extraction allows for accurate genotyping and expression analysis, impacting discoveries in genetics.
- Forensic Analysis: In forensic science, the ability to extract DNA from challenging samples can be critical for solving cases and identifying individuals.
- Biotechnology: In the biotechnology sector, efficient extraction methods facilitate the development of genetically modified organisms and therapeutic agents.
The quality of DNA and RNA extraction can significantly affect the outcome of experiments and applications, emphasizing the need for rigor in method selection.
Current Trends in Science
In an era of technological advancements, new trends are emerging that enhance extraction efficiencies and expand the applicability of methods.
Innovative Techniques and Tools
Novel technologies are reshaping the landscape of DNA and RNA extraction, including:
- Automated extraction systems: These systems minimize human error, increase throughput, and maintain consistency in results. They are particularly useful in high-throughput settings.
- Microfluidics: This technology allows for manipulating small volumes of fluid, facilitating rapid nucleic acid extraction and analysis. It holds promise for personalized medicine by enabling quick diagnostics.
Interdisciplinary Connections
The interaction between different scientific fields is evident in extraction techniques. For instance, developments in materials science contribute to better binding materials for DNA extraction. Collaborations between biologists and engineers are leading to innovative approaches that enhance both yield and simplicity in extraction processes.
As these trends continue to evolve, they will undoubtedly shape the future of molecular biology and its applications.
Preamble to Extraction Techniques
Understanding extraction techniques for DNA and RNA is paramount for various scientific fields. The extraction of these nucleic acids is foundational in molecular biology, genetics, forensics, and biotechnology. By effectively isolating DNA or RNA from biological materials, researchers can analyze genetic information, investigate diseases, and develop new therapeutic strategies. This article delves into several extraction methods, highlighting their underlying principles, benefits, and considerations.
Importance of DNA and RNA Extraction
DNA and RNA extractions facilitate numerous applications. These include genetic testing, sequencing, cloning, and expression analysis. Practitioners rely on high-quality nucleic acids for accurate results. The importance lies not only in the extraction techniques themselves but also in their ability to produce consistent and reproducible results. A clean extraction leads to higher yield and purity levels, enabling effective downstream applications.
In forensic science, DNA extraction aids in identifying individuals based on genetic material. In clinical diagnostics, RNA extraction becomes essential for detecting pathogens or diagnosing diseases. The versatility of techniques available further emphasizes the necessity of understanding these methodologies, as different applications may require specific approaches to extraction, tailored to the sample type.
Historical Context
Historically, the extraction of nucleic acids has evolved significantly. Early techniques often involved labor-intensive methods that yielded mixed results. Before the 1970s, researchers primarily utilized phenolic compounds for extraction, which, although effective, had many drawbacks such as toxic fumes and less efficient separation of nucleic acids.
The introduction of more refined methods, particularly the silica-based approaches in the late 20th century, marked a turning point. These methods leveraged the unique properties of silica to bind nucleic acids in the presence of specific salts, resulting in much cleaner extracts. Automated systems have further modernized the field, enabling researchers to process multiple samples quickly and efficiently. This journey illustrates the continuous drive for improved techniques, aligned with technological advancements to optimize yield and purity in nucleic acid extraction.
Fundamentals of DNA and RNA
Understanding the fundamentals of DNA and RNA is crucial for researchers and professionals involved in molecular biology. DNA, or deoxyribonucleic acid, and RNA, or ribonucleic acid, are the essential molecules that carry genetic information and play vital roles in biological functions. The study of these molecules provides insights that are foundational for advancements in genetic research, clinical diagnostics, and even forensic science.
This section will discuss the chemical composition of these nucleic acids, their structural differences, and their implications in various extraction techniques.
Chemical Composition
The chemical composition of DNA and RNA is characterized by nucleotides, which are the building blocks of these molecules. Each nucleotide includes three components: a nitrogenous base, a sugar, and a phosphate group. For DNA, the nitrogenous bases comprise adenine (A), thymine (T), cytosine (C), and guanine (G). In contrast, RNA features uracil (U) instead of thymine, substituting A, C, G, and U.
The sugar present in DNA is deoxyribose, while RNA contains ribose. This difference is significant as it affects the overall stability and function of these nucleic acids. DNA’s double-helix structure is resilient, allowing it to store genetic information for long periods. RNA is typically single-stranded, making it more versatile for various biological roles, including as a messenger in protein synthesis.
"Understanding the chemical composition of DNA and RNA informs extraction methods, impacting their efficiency and purity."
This knowledge is critical when selecting appropriate extraction techniques. For instance, methods that rely on chemical lysis will differ based on the molecular structure of DNA or RNA, impacting yields and purity.
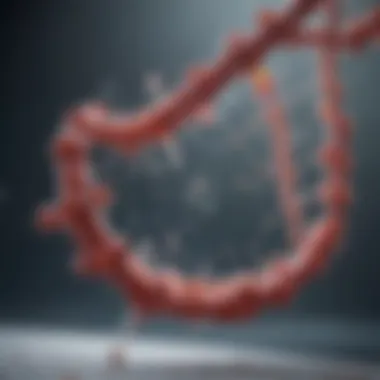
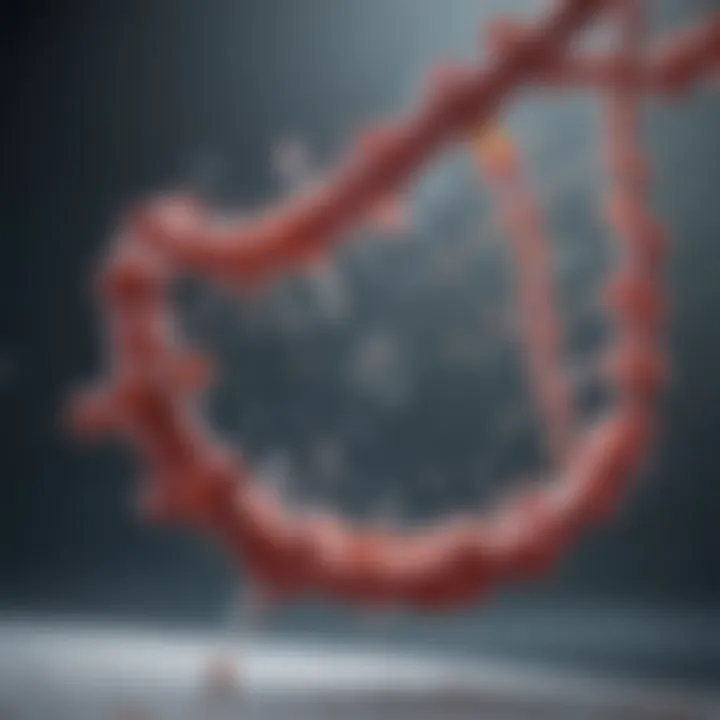
Structural Differences
The structural differences between DNA and RNA are significant and influence their biological roles.
- Double-helix vs. Single-stranded: DNA’s double-helix structure consists of two intertwined strands of nucleotides, giving it stability and protecting genetic information. Conversely, RNA’s single-stranded form allows it to fold into various shapes, enabling a broad range of functions within the cell.
- Base Pairing: In DNA, adenine pairs with thymine, and cytosine pairs with guanine. This pairing is crucial for DNA replication and transcription. In RNA, adenine pairs with uracil, creating different bonding patterns and implications for stability and function.
- Size and Length: Typically, DNA molecules are much longer than RNA. Genomic DNA can be millions of nucleotides long, whereas RNA transcripts are usually much shorter, tailored for specific protein synthesis tasks.
Understanding these structural nuances is essential for evaluating extraction techniques. The method selected often relies on the form of the nucleic acid involved, its stability under certain conditions, and the specific application of the extracted material.
These fundamentals set the stage for the subsequent examination of extraction techniques. Given the critical roles that DNA and RNA play in biotechnological applications, a deep understanding of their composition and structure directly influences the methodology used in their extraction.
Common Extraction Techniques
In the realm of molecular biology, common extraction techniques play an essential role. These methods are crucial for isolating and purifying DNA and RNA from various biological sources. Researchers need reliable extraction techniques to obtain high-quality nucleic acids for downstream applications. By understanding the specific extraction methods, individuals can make informed choices that improve the yield and purity of extracted samples.
Common extraction techniques each have unique benefits and considerations. The choice of method may depend on several factors, including the type of sample, the desired purity levels, and the intended application of the extracted nucleic acids. Below, we will explore several widely used extraction methods in detail.
Phenol-Chloroform Method
One of the oldest and most established techniques is the phenol-chloroform method. This technique utilizes a mixture of phenol and chloroform to separate nucleic acids from proteins and other contaminants. The combination creates two distinct phases during centrifugation. The aqueous phase contains the nucleic acids, while denatured proteins and lipids migrate to the organic phase.
This method is noteworthy for its effectiveness in achieving high purity levels. However, it requires careful handling due to the toxic nature of the chemicals involved. The protocol often includes multiple washing steps to ensure contaminants are minimized, making it time-intensive but reliable in yielding pure samples.
Silica-Based Methods
Silica-based extraction methods have gained significant traction due to their simplicity and speed. These techniques commonly use silica membranes or columns, which selectively bind DNA or RNA in the presence of high salt concentrations. When the sample is washed with an appropriate buffer, contaminants can be removed, leaving behind purified nucleic acids.
The benefits of silica-based methods include reduced processing time and ease of use. They can be easily automated, making them suitable for high-throughput applications. However, the effectiveness can sometimes vary depending on sample composition, which is something researchers need to consider when selecting a method.
Magnetic Bead-Based Techniques
Magnetic bead-based techniques are another innovative option for DNA and RNA extraction. This method involves the use of magnetic beads coated with specific functional groups that bind nucleic acids. When mixed with a biological sample, the beads capture the nucleic acids, which can then be isolated using a magnet.
The advantage of magnetic bead methods is their flexibility and convenience. They are suitable for various sample types and are often amenable to automation. Extraction processes can be completed rapidly, although the binding efficiency can vary with different samples. Considering these factors is important for researchers looking to optimize their extraction protocols.
Column Purification
Column purification techniques enable straightforward extraction of nucleic acids using porous columns packed with specialized resins. The biological sample is applied to the column, allowing nucleic acids to bind to the resin while contaminants wash away. Following washing, nucleic acids can be eluted using low-salt buffers or water.
This method is particularly useful for yielding high-quality nucleic acids quickly and effectively. However, researchers should be aware of potential variability in results based on factors such as sample type and column specifications. Overall, column purification provides a robust extraction alternative that many researchers find beneficial for specific applications.
Emerging Technologies in Extraction
Emerging technologies in DNA and RNA extraction represent a significant leap forward in efficiency, precision, and adaptability. These advancements are crucial for researchers seeking to optimize their methodologies while handling various biological samples. As the demand for high-quality genetic material grows across fields such as genetics, forensics, and clinical diagnostics, so too does the necessity for modern extraction techniques that enhance both yield and purity.
Automated Extraction Systems
Automated extraction systems have transformed the landscape of molecular biology. These systems use robotics to streamline the extraction process, minimizing human error and increasing reproducibility. The introduction of automation allows for high-throughput extraction, making it possible for labs to process numerous samples in a fraction of the time compared to manual methods.
One notable benefit of automated systems is their ability to maintain consistency across experiments. By eliminating variations that may arise from manual handling, automated systems ensure more reliable results. Additionally, many of these systems incorporate cutting-edge technology such as real-time monitoring and integrated software for data analysis.
However, there are considerations to keep in mind. The upfront cost for automated systems can be significant. Institutions must weigh the initial investment against the long-term benefits of increased efficiency and output. Moreover, staff may require training to operate these sophisticated systems effectively.
Microfluidics
Microfluidics is another innovative approach that has gained traction in the field of DNA and RNA extraction. This technology involves manipulating very small volumes of fluids, often in the microliter range. It allows for the miniaturization of extraction processes, which can drastically reduce the amounts of reagents used while also decreasing waste.
The principle behind microfluidics is its ability to provide precise control over biological or chemical interactions. This level of control can lead to enhanced extraction efficiency and improved purity of the nucleic acids obtained. By reducing the scale of operations, microfluidics also permits faster processing times, which is essential when dealing with large sample sizes or urgent diagnostic applications.
Beyond technical benefits, microfluidic devices can be integrated with other systems. For example, combining microfluidics with automated systems can create a powerful platform for large-scale genetic analysis. Moreover, these devices can be designed to function in remote settings, offering new possibilities for field research.
Emerging technologies in extraction are not just tools for research; they define the cutting edge of biochemical exploration.
Sample Preparation Considerations
Sample preparation is a vital step in DNA and RNA extraction, affecting the yield and purity of nucleic acids. Understanding the specifics of each sample type helps in optimizing extraction processes. Several factors come into play, including the nature of the biological sample, its storage, handling techniques, and the extraction method employed. Each of these elements directly impacts the efficiency of the extraction process, which is crucial for downstream applications such as genetic analysis and diagnostics.
Type of Biological Samples
Plant Samples
Plant samples are often utilized for DNA and RNA extraction due to their widespread availability. The key characteristic of plant samples is their complex cell structure, which includes rigid cell walls. This can pose challenges during extraction, as the presence of polysaccharides and phenolic compounds can inhibit the purity of the extracted nucleic acids. However, the richness of genetic diversity in plants makes them a beneficial choice for genetic study and agricultural research.
One unique feature of plant samples is their ability to store and accumulate a variety of metabolites that can affect the extraction. For instance, while some compounds may enhance flavor and aroma, others can lead to lower yields or degraded samples. Proper sample processing is necessary to minimize these disadvantages while taking advantage of the high genetic variability found in plant species.
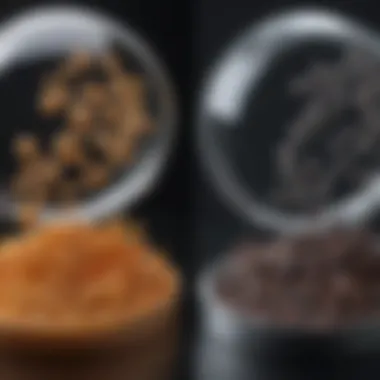
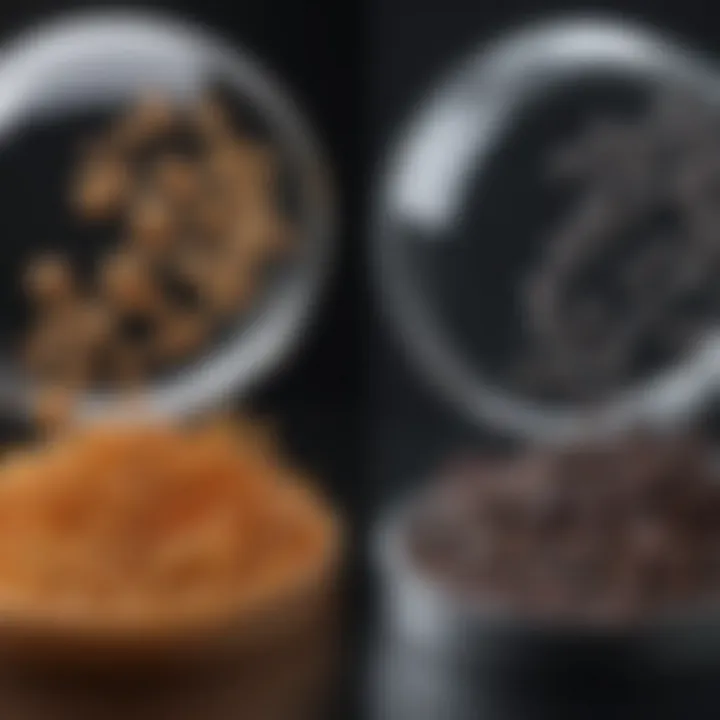
Animal Tissue
Animal tissue is another significant source for DNA and RNA extraction, particularly in fields like genetics and medical research. The key characteristic here is the relative ease of isolating nucleic acids due to less complex structures compared to plant cells. Animal tissues typically yield high-quality DNA and RNA, making them a popular choice in this article.
A unique feature of animal tissue is its sensitivity to handling conditions. Factors such as temperature and time can drastically affect the integrity of the extracted nucleic acids. Fresh samples are preferred, and special storage conditions are often required to ensure their stability. This can be an advantage, as high-quality results tend to be achievable when standard protocols are followed.
Cell Cultures
Cell cultures serve as model systems for DNA and RNA extraction due to their controlled environment and uniformity. Cultured cells provide a consistent source of genetic material, which is crucial for experiments that demand reproducibility. The key characteristic of cell cultures is their ability to grow and proliferate, allowing for larger sample sizes over time.
However, cell cultures can also pose challenges. Contamination is a common issue that can lead to variable results. Additionally, different cell types may require specific handling and extraction protocols, complicating the process. Nevertheless, the advantages of using cell cultures in research—like controlled experimental conditions and standardization—make them a valuable choice in nucleic acid extraction workflows.
Storage and Handling
Proper storage and handling of biological samples is crucial for preserving the integrity of nucleic acids. Factors such as temperature, humidity, and duration of storage can all impact the quality of the samples. For optimal results, it is necessary to adhere to specific guidelines for each sample type. Using suitable containers and maintaining conditions that prevent degradation and contamination will ultimately support the success of DNA and RNA extraction processes.
In molecular biology, the preparation of samples is not just a procedural step, but a foundation supporting the validity of subsequent analyses.
By prioritizing sample preparation considerations, researchers can enhance the quality of their work and improve the reliability of their findings.
Efficiency and Yield Parameters
Efficiency and yield are critical components in DNA and RNA extraction, as they directly influence the utility of the extracted genetic material. Optimizing extraction processes ensures that researchers and practitioners receive high-quality, usable samples for their work. The importance of these parameters lies in their effects on downstream applications, which may include genetic research, diagnostics, and forensic analysis. Therefore, understanding the factors affecting yield and evaluating extraction efficiency are essential for achieving reliable and reproducible results.
Factors Affecting Yield
Sample Size
The sample size affects the total amount of DNA or RNA collected during extraction. A larger sample size generally leads to higher yields, as it provides more target material for the extraction process. This characteristic makes sample size a beneficial consideration when aiming for increased yield. However, using larger samples can also introduce variability in results due to the potential for increased contamination and degradation of genetic material. Thus, it is essential to balance sample size with the quality of the sample to achieve desirable outcomes in extraction efficiency.
Extraction Method
The extraction method chosen plays a significant role in determining the efficiency and yield of nucleic acid extraction. Different techniques, such as phenol-chloroform, silica-based methods, and magnetic bead approaches, impact the final yield due to their inherent characteristics. Phenol-chloroform extraction, for instance, is well-regarded for its ability to yield high-quality nucleic acids but can be time-consuming and hazardous. In contrast, magnetic bead methods provide simplicity and speed, though they may result in lower yields depending on the sample type and conditions. Understanding the nuances of each method is crucial for selecting the appropriate strategy for specific applications.
Purity Levels
Purity levels are integral to assessing the usability of extracted DNA or RNA. High purity ensures that the extracted genetic material is free from contaminants that may interfere with subsequent analyses. This factor highlights the importance of purification steps during extraction, as higher purity often correlates with increased yield and efficiency. Additionally, purity levels are a critical criterion for various applications, such as sequencing or qPCR, where even slight contaminant presence can affect results. Balancing yield with purity is necessary to meet the demands of diverse applications in genetic research and diagnostics.
Evaluating Extraction Efficiency
Evaluating extraction efficiency involves assessing both the quantity and quality of the extracted nucleic acids. Quantitative methods such as UV spectrophotometry can measure absorbance ratios, helping users determine the concentration and purity of the target nucleic acids. Qualitative assessments, like agarose gel electrophoresis, visualize the integrity of extracted materials, allowing researchers to confirm that the nucleic acids are suitable for analysis.
In summary, efficiency and yield parameters are essential considerations in DNA and RNA extraction. By understanding the factors that influence yield, such as sample size, extraction methods, and purity levels, researchers can make informed choices that enhance the quality of their extracted genetic materials.
Purity Assessment Techniques
Purity assessment techniques are essential for ensuring the integrity and usefulness of extracted DNA and RNA. These techniques enable researchers to evaluate the purity of nucleic acid samples, which directly impacts the success of downstream applications such as cloning, sequencing, and polymerase chain reaction (PCR). Identifying and quantifying contaminants helps researchers avoid false results and improve reproducibility. Understanding the methods for assessing purity is a crucial step in optimizing extraction processes and ensuring the reliability of experimental outcomes.
UV Spectrophotometry
UV spectrophotometry is a widely used method for assessing the purity of nucleic acids. This technique takes advantage of the unique absorption characteristics of DNA and RNA at specific wavelengths. Typically, DNA absorbs light at 260 nm, while proteins absorb at 280 nm. By measuring the absorbance at these wavelengths, researchers can calculate the A260/A280 ratio, which serves as an indicator of nucleic acid purity.
An optimal A260/A280 ratio for pure DNA is around 1.8, and for RNA, it is approximately 2.0. If the ratio is significantly lower, it may suggest protein contamination, while higher values may indicate the presence of phenol or other organic solvents.
Benefits of UV Spectrophotometry:
- Quick and Simple: The method is relatively quick and easy to perform, requiring minimal sample handling.
- Quantitative Measurement: It provides direct quantitative data about nucleic acid concentration and purity.
- Non-destructive: The sample integrity is maintained, allowing for subsequent analyses.
However, it is important to note that UV spectrophotometry can only provide a general evaluation of purity. It does not differentiate between various types of contaminants, making it necessary to complement this method with further techniques for thorough assessment.
Agarose Gel Electrophoresis
Agarose gel electrophoresis is another powerful technique used for assessing nucleic acid purity and integrity. This process involves the separation of DNA and RNA fragments in an agarose gel matrix under an electric field. Larger fragments migrate more slowly than smaller ones, thus allowing for the determination of size and quantity.
Monitoring the appearance of bands on the gel provides insights into the sample quality. Sharp and well-defined bands indicate high purity and intact nucleic acids. Conversely, fragmented bands may suggest degradation or contamination.
Key Points about Agarose Gel Electrophoresis:
- Visualization: The technique allows for visual inspection of nucleic acids, often using dyes such as ethidium bromide for fluorescence.
- Resolution: It can resolve a range of DNA or RNA fragment sizes, facilitating the assessment of sample integrity.
- Comprehensive Evaluation: By assessing both size and quality, this method provides a more detailed evaluation compared to UV spectrophotometry alone.
This technique also facilitates the assessment of molecular weight, which is often necessary for certain applications in molecular biology. Overall, agarose gel electrophoresis is a reliable method for determining nucleic acid purity, making it a standard practice in many laboratories.
The ability to accurately assess nucleic acid purity is crucial for achieving reliable results in molecular biology experiments.
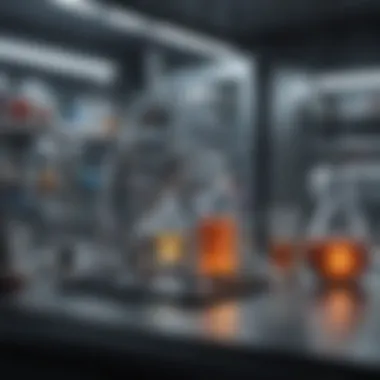
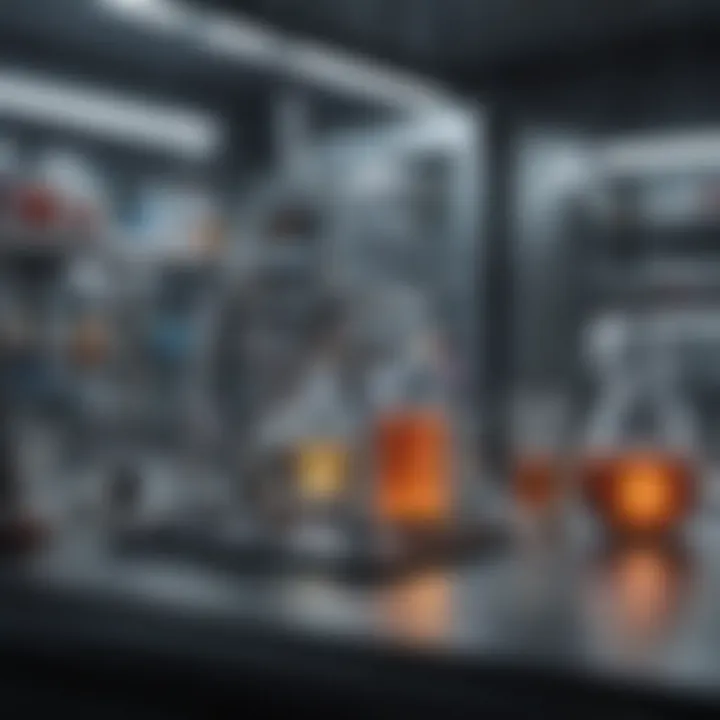
Both UV spectrophotometry and agarose gel electrophoresis play vital roles in purity assessment. Depending on laboratory needs, these methods can be used independently or in conjunction to achieve a comprehensive evaluation of the nucleic acid quality.
Applications of Extracted DNA and RNA
The extraction of DNA and RNA serves pivotal roles across various domains in modern science. Understanding these applications is essential for researchers and practitioners. They find utility in fields such as genetic research, forensic science, and clinical diagnostics. By delving into these applications, we can appreciate the profound impact that effective extraction techniques have on advancing knowledge and solving real-world problems.
Genetic Research
In genetic research, extracted DNA and RNA is fundamental for a variety of studies. Researchers use these nucleic acids to clone genes, conduct sequencing projects, and study genetic mutations. High-quality DNA enables precise analysis, leading to discoveries about gene function. RNA extraction is equally crucial, especially for gene expression studies. It allows examination of how different genes are expressed under various conditions.
- Enhanced understanding of diseases through genetic analysis.
- Development of gene therapies and other treatment strategies.
Through these pursuits, researchers can not only comprehend diseases better but also develop innovative approaches to tackling them. The application of extracted DNA and RNA continues to expand as technology improves.
Forensic Science
Forensic science relies heavily on DNA extraction for criminal investigations and legal matters. The ability to collect and analyze genetic material from crime scenes has transformed the field of criminology. DNA profiles generated from extracted samples can connect suspects to crimes or exonerate the innocent.
Some notable applications include:
- Identification of individuals through DNA fingerprinting.
- Solving cold cases by re-examining old DNA samples with modern techniques.
Furthermore, DNA evidence can be pivotal in court, offering strong proof that can lead to convictions or acquittals. The precision and reliability of forensic applications underscore the importance of effective extraction techniques.
Clinical Diagnostics
In clinical settings, extracted DNA and RNA plays a crucial role in diagnostics. Genetic tests can detect hereditary conditions, infectious diseases, and even predispositions to certain illnesses. The ability to analyze RNA is particularly important in understanding ailments at a molecular level, such as cancer.
Key benefits in clinical diagnostics include:
- Early detection of diseases through genetic screening.
- Tailoring of personalized medicine based on genetic profiles.
The integration of extracted nucleic acids into clinical practice enhances the capability to diagnose and treat patients effectively. This precision medicine approach represents a significant leap forward in patient care and outcomes.
The importance of extracting DNA and RNA transcends the laboratory and reaches into everyday life.
Troubleshooting Common Issues
Troubleshooting common issues is a critical component in the process of DNA and RNA extraction. Even small discrepancies in methodology can lead to significant problems in yield and quality of the extracted nucleic acids. Addressing these issues promptly ensures that research outcomes remain valid and reproducible, catering to both academic and practical applications in molecular biology. Understanding potential pitfalls can help researchers improve their extraction processes and achieve more consistent results.
Contamination Problems
Contamination is perhaps the most prevalent challenge faced during DNA and RNA extraction. This refers to the introduction of unwanted nucleic acids or other compounds into the extracted sample, which can affect the integrity and usability of the results. Sources of contamination can vary significantly:
- Environmental Contaminants: Dust, airborne microorganisms, and residues from previous experiments can easily disrupt the purity of extracted nucleic acids.
- Biological Contaminants: Cross-contamination from nearby samples or improper handling can inadvertently introduce foreign substances into the sample.
- Reagent Contamination: Using reagents or consumables that are not certified for nucleic acid work can lead to contamination issues.
To mitigate these risks, it is essential for researchers to implement strict laboratory protocols. For instance, adopting a clean working environment, wearing gloves, and using sterilized equipment helps reduce contamination risk. Additionally, frequent validation of reagents and careful planning during sample handling can further minimize contamination.
Low Yield Concerns
Low yield of extracted DNA and RNA is another common issue faced during these extraction processes. Low yield means the quantity of nucleic acids obtained is less than expected, which can hinder downstream applications. Some factors contributing to low yield include:
- Sample Size: Smaller samples inherently produce lower yields, especially if the biological material is limited.
- Extraction Method: Not all extraction methods are efficient for every type of sample; thus, selecting an inappropriate technique can lead to insufficient nucleic acids.
- Storage and Handling Conditions: Improper storage of samples prior to extraction or delays in processing can lead to degradation of nucleic acids, thus affecting yields beforehand.
To address low yield concerns, researchers can evaluate their choice of extraction method. If necessary, they should consider switching to a technique that better accommodates the sample type. Additionally, optimizing conditions such as incubation time and temperature during the extraction process can significantly enhance nucleic acid yield. Regularly assessing the effectiveness of the extraction protocol enables ongoing refinement, ensuring maximum recovery of DNA and RNA.
Future Directions and Innovations
The area of DNA and RNA extraction continues to evolve rapidly. As research in molecular biology demands higher efficiency and precision, the future of extraction techniques plays a crucial role in meeting these expectations. The innovations emerging in this field not only enhance yield and purity but also adapt to various types of biological samples.
Moreover, continued advancements in extraction methods can revolutionize several scientific domains, including genetics, forensic science, and clinical diagnostics. A focus on future trends provides insights into how extraction techniques may become more efficient and accessible while addressing current challenges.
Next-Generation Sequencing Impact
Next-generation sequencing (NGS) has fundamentally changed how researchers view DNA and RNA extraction. Traditional methods often fell short of meeting the rigorous standards required for sequencing. However, with NGS protocols, more sophisticated extraction methods are emerging. These methods prioritize the integrity of nucleic acids, because the quality of extracted samples directly impacts the sequencing results.
"As sequencing technologies advance, so must our extraction methods to ensure compatibility and optimal outcomes."
The focus on NGS-integrated extraction techniques is critical because it:
- Improves overall data accuracy.
- Reduces the time for sample preparation.
- Minimizes contamination risks, leading to more reliable outcomes.
The integration of NGS with automation also streamlines workflows, allowing for higher throughput and consistency.
Biotechnological Advances
Biotechnology has introduced various innovations that promise to enhance DNA and RNA extraction techniques. For instance, improved reagents and extraction kits are now available, catering to specific kinds of samples. This tailored approach leads to better yields and higher purity levels, which are essential in downstream applications.
Furthermore, advancements in robotic automation have seen an increase in efficiency in laboratory environments. These systems can execute extraction protocols with minimal human input, thereby reducing error rates. Biotechnological developments are aimed at:
- Enhancing extraction specificity through better capture molecules.
- Utilizing machine learning to optimize extraction protocols based on sample data.
- Improving scalability, allowing labs to adapt to varying project sizes with ease.