The Dynamics of Automotive Motion Explained
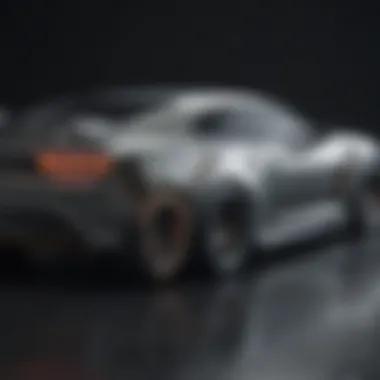
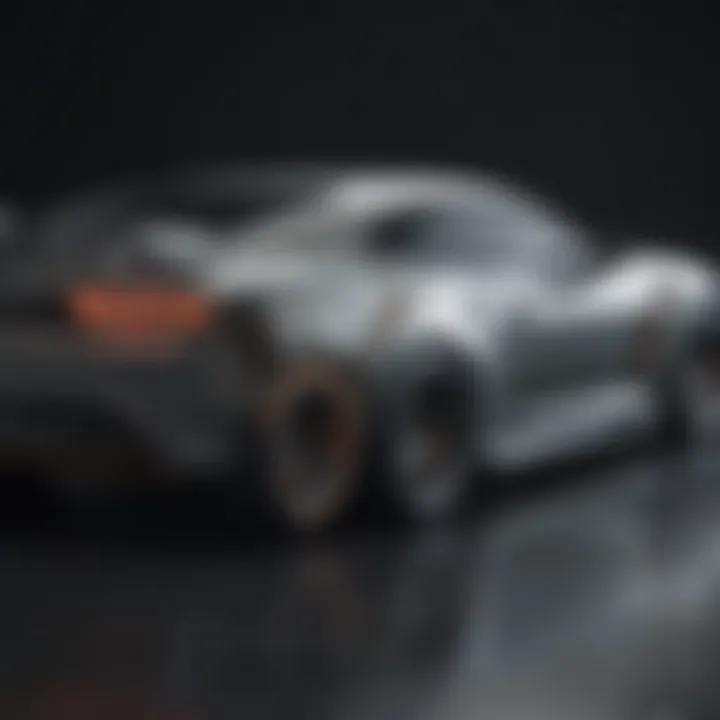
Intro
Automobiles are integral to modern society, allowing for the seamless movement of people and goods. Understanding how cars achieve motion involves delving into various principles of physics and engineering. This exploration not only covers the basic mechanics but also extends to advanced technologies shaping the future of automotive design.
The discussion around automotive dynamics includes concepts such as force, torque, and energy, all fundamental in comprehending how vehicles operate. Additionally, it touches on the latest advancements in propulsion and sustainability. As the automotive landscape evolves, it is crucial to appreciate the interaction between these varied elements.
Research Overview
Methodological Approaches
In approaching the study of automotive motion, researchers employ a range of methods, blending theoretical frameworks with empirical analysis. Experimental setups often include:
- Wind tunnel tests to evaluate aerodynamic properties.
- Simulation models utilizing software to predict vehicle performance under various conditions.
- Field tests on public roads to gather real-world data.
These methodologies contribute to a comprehensive understanding of how different factors influence car dynamics. By integrating data from varied sources, researchers enhance the reliability of their findings.
Significance and Implications
The significance of studying automotive motion extends beyond mere academic interest. Understanding these dynamics has real-world implications, including:
- Enhancing safety features in vehicles, which saves lives.
- Promoting fuel efficiency, thereby reducing environmental impact.
- Informing legislation on automotive standards and regulations.
As advancements in technology continue to disrupt traditional paradigms, the insights gained from this research are pivotal for future innovations in the automotive sector.
Current Trends in Science
Innovative Techniques and Tools
The automotive industry is witnessing a surge in innovative techniques that reshape how we perceive vehicle motion. Some notable developments include:
- Electric propulsion systems, which challenge traditional internal combustion engines.
- Regenerative braking, improving energy efficiency during vehicle operation.
- Advanced driver-assistance systems (ADAS) that utilize sensors and data analytics for enhanced control.
These innovations are not only changing how vehicles move but also influencing consumer preferences and regulatory landscapes.
Interdisciplinary Connections
The exploration of automotive dynamics often requires interdisciplinary approaches. Collaboration between fields such as robotics, materials science, and computer engineering has proven beneficial. This convergence allows for:
- Improved vehicle design through smarter materials that enhance safety and performance.
- Development of AI-driven systems that optimize routes and improve traffic flow.
By understanding these connections, industry professionals can foster a more integrated approach to automotive innovation.
"The ongoing evolution in automotive technology offers a glimpse into a future where vehicles are smarter, safer, and more environmentally friendly."
As we delve deeper into the interplay of these dynamics, it becomes clear that the journey of understanding automotive motion is not only about learning how cars go, but also about shaping a sustainable and efficient future.
Prelude to Automotive Motion
Understanding automotive motion is critical in grasping how vehicles interact with their environment. This section delves into the complex dynamics that allow cars to function effectively and efficiently. Beyond merely transporting individuals or goods, an appreciation of automotive motion opens up insights into engineering challenges, safety standards, and environmental impact.
As vehicles evolve, so does the necessity to comprehend their motion. This comprehension aids in the design of more efficient cars, improves safety features, and shapes the future of transportation. By exploring the underlying principles of motion, readers can gain a clearer vision of how innovation in this field could address issues such as traffic congestion and pollution.
Historical Context of Automobile Development
The journey of the automobile begins in the late 19th century. Karl Benzโs introduction of the first gasoline-powered vehicle in 1885 marked the inception of the automotive era. This revolutionary step catalyzed a series of innovations that would forever change personal and commercial transportation. Early automobiles were simple and lacked the complexity found in todayโs vehicles. As these machines advanced, so did the understanding of mechanical principles governing motion and control.
Over the decades, the automotive industry saw rapid development. The assembly line technique pioneered by Henry Ford transformed production. It enabled mass manufacturing of vehicles, making cars accessible to the general public. This democratization of mobility brought forth new societal dynamics, from suburban sprawl to the rise of road culture. Such historical perspectives reflect how advancements in automotive motion have influenced daily life and economic structures throughout the 20th century and beyond.
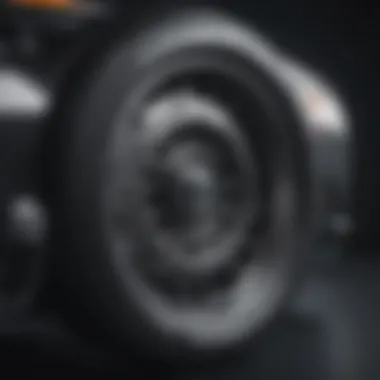
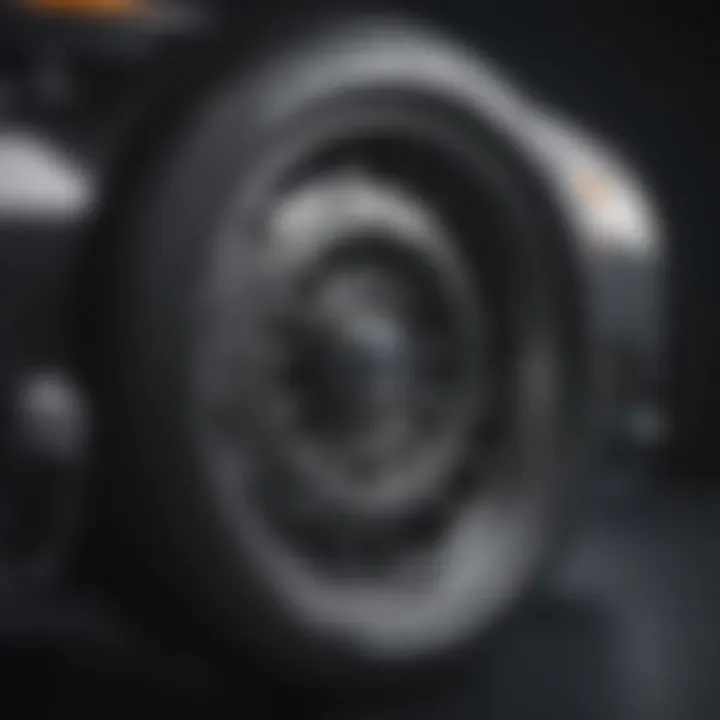
Basic Principles of Motion
Motion can be described through fundamental principles rooted in physics. Key among these are displacement, velocity, and acceleration. Displacement refers to the change in position of a vehicle, while velocity accounts for the speed of this change with direction. Acceleration, on the other hand, reflects how quickly a vehicle can increase its speed.
In automotive terms, several forces come into play that affect movement:
- Engine Power: This initiates movement by converting fuel into kinetic energy.
- Friction: Essential for enabling vehicles to grip the road. Without friction, cars would slide out of control.
- Drag: The air resistance faced by moving vehicles. It acts against forward motion, requiring more power to maintain speed.
Understanding these principles provides a foundation for analyzing more complex dynamics in automotive motion, paving the way for deeper inquiries into engineering and technology.
Physics of Vehicle Motion
The physics of vehicle motion is a critical aspect of understanding how automobiles operate. This section investigates the fundamental principles that govern the movement of cars. Understanding these principles allows engineers to design more efficient vehicles, informs drivers about vehicle handling, and aids in the development of safety features. The fundamental laws of physics dictate how vehicles accelerate, brake, and maneuver. A comprehensive grasp of these concepts is essential for those working or studying in the automotive field. It provides the necessary foundation for advancements in technology and design that are informed by physical laws.
Newton's Laws Applied to Cars
Sir Isaac Newton's three laws of motion are foundational to the understanding of vehicle dynamics. Each law plays a vital role in how cars move and respond to forces.
- First Law (Inertia): A car will remain at rest or in uniform motion unless acted upon by an external force. This means that a parked car will not move until either the driver engages the accelerator or an outside force, like gravity on a steep hill, influences its motion.
- Second Law (F=ma): The acceleration of a vehicle is directly proportional to the net force acting on it and inversely proportional to its mass. In practical terms, a powerful engine allows a car to accelerate quicker, as the force generated by the engine overcomes the mass of the vehicle.
- Third Law (Action-Reaction): For every action, there is an equal and opposite reaction. This principle is crucial when examining how tires push against the road and, in return, how the road pushes back, allowing the vehicle to move forward.
Understanding these laws helps in optimizing performance and improving vehicle safety.
Force, Mass, and Acceleration
Force, mass, and acceleration are interconnected via Newton's second law. The relationship can be summarized as follows:
- Force is what propels the vehicle forward. It originates from the engine via the drivetrain to the wheels. The greater the force generated by the engine, the faster the vehicle can accelerate.
- Mass refers to the weight of the car. A lighter vehicle requires less force to achieve the same acceleration compared to a heavier one. Therefore, engineers focus on reducing mass through lightweight materials while maintaining structural integrity.
- Acceleration is the response of a vehicle to applied force. It indicates how quickly a car can increase its speed.
Understanding this interplay aids in creating more efficient vehicles that meet performance standards while balancing weight and power.
Friction and Traction Dynamics
Friction plays a crucial role in vehicle motion. It is the resistance that one surface or object encounters when moving over another. The dynamics of traction, or the grip between the tires and the road, are essential for safe and efficient driving.
- Types of Friction: The two main types are static friction and kinetic friction. Static friction prevents movement until a certain limit of force is overcome, while kinetic friction occurs once the tires are sliding.
- Coefficient of Friction: This is a numerical value that describes the degree of friction between the road and the tires. High coefficients indicate better traction, essential for functions like braking and cornering.
- Tire Design and Material: The composition and tread pattern of tires significantly influence traction. Tires designed for specific conditions, such as wet or icy roads, exhibit varied performance characteristics.
"An understanding of friction dynamics is crucial for automotive design, playing a conditional role in vehicle safety and performance."
By analyzing these factors, engineers can design systems that enhance stability and control, key elements in automotive motion.
Engineering Behind Automotive Motion
Understanding the engineering behind automotive motion is crucial for grasping how vehicles operate and respond to various driving conditions. The intricate systems involved in modern vehicles govern their performance, safety, and efficiency on the road. Each component must work in harmony, producing a reliable and enjoyable driving experience.
The effectiveness of automotive motion equations stems from the advanced engineering principles that dictate the design and functionality of each vehicle part. For instance, the powertrain system is fundamental to how energy is transformed into motive power. The integration of transmission systems ensures that this energy is used efficiently, while suspension and steering mechanisms provide stability and control. Without attention to these engineering elements, vehicles would not be able to provide the levels of comfort and safety that consumers expect today.
Powertrain Components and their Roles
The powertrain is at the core of any vehicle's motion. It comprises several components, each playing a distinct role in converting energy into movement. The primary elements include the engine, transmission, driveshaft, and differential.
- Engine: This is the heart of the powertrain. Whether it is an internal combustion engine or an electric motor, the engine generates power that drives the vehicle. Each type of engine operates based on different principles, leading to variations in power delivery and efficiency.
- Transmission: This component transfers the engineโs power to the wheels. It allows the driver to control vehicle speed and torque, adapting to different road conditions and driving scenarios.
- Driveshaft: Linkage is established between the transmission and the wheels through the driveshaft, transferring power so the vehicle can move forward or backward.
- Differential: This unit allows the wheels to rotate at varying speeds, which is essential during cornering. It helps in maintaining stability and traction.
Each powertrain component must be appropriately matched to ensure optimal performance. Disparities in these elements can lead to inefficiencies and reduced driving pleasure.
Transmission Systems: Types and Functions
Transmission systems play an essential role in controlling how the power generated by the engine is utilized. There are several types of transmission systems, including automatic, manual, and continuously variable transmissions (CVT). Each system offers unique characteristics and benefits.
- Manual Transmission: This system provides drivers with complete control over gear selection. While it requires more effort, many enthusiasts prefer it for the enhanced connection to the vehicle.
- Automatic Transmission: Automatically shifts gears based on speed and throttle input, making it user-friendly and smooth for most drivers.
- Continuously Variable Transmission (CVT): This innovative system adjusts gear ratios seamlessly, maximizing efficiency. It often results in better fuel economy at the cost of some traditional driving engagement.
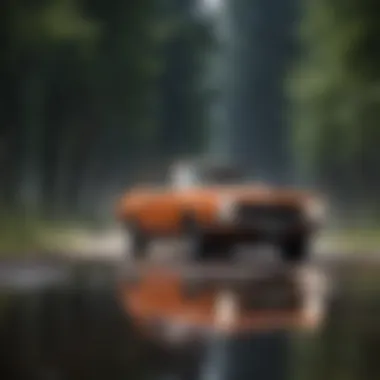
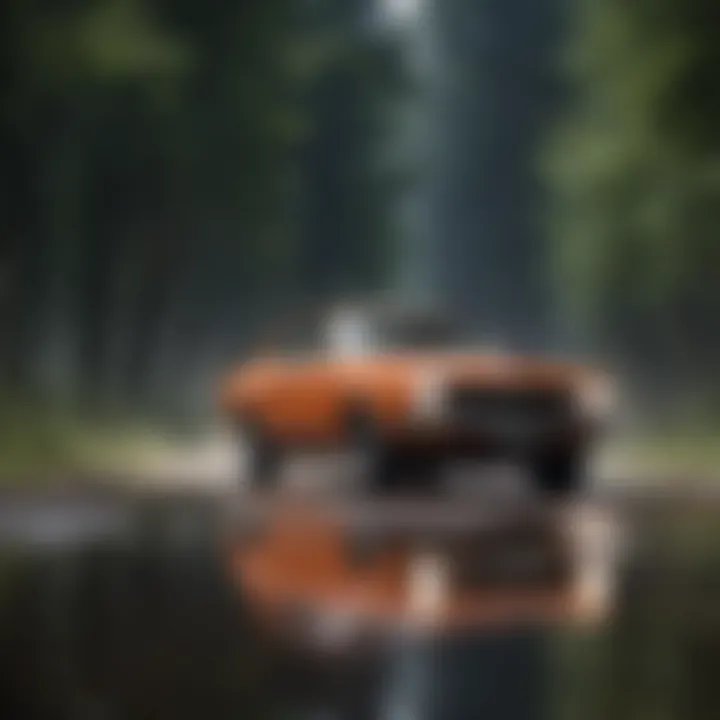
The choice of transmission can significantly affect vehicle performance, fuel economy, and overall driving experience. For instance, a well-tuned automatic transmission can enhance acceleration and reduce fuel consumption, while a manual transmission might deliver a more engaging driving dynamic.
Suspension and Steering Mechanisms
The suspension and steering systems are pivotal in ensuring vehicle stability, handling, and comfort. Properly designed suspension systems absorb road shocks, maintaining tire contact with the ground for effective traction.
- Suspension Types: Different suspension designs include independent suspensions, solid axles, and air suspensions, each offering distinct advantages for vehicle dynamics. Independent suspension systems, for instance, improve ride comfort and handling by allowing each wheel to move independently, thereby mitigating the effects of road imperfections.
- Steering Mechanisms: Steering systems enable maneuvering and control, critical for safety. Traditional rack-and-pinion systems and modern electronic power steering systems (EPS) both have their unique benefits, including feedback and precision in control.
An effective suspension and steering setup enhances driving experience, comfort, and safety, making these systems essential for automotive designers.
"The fusion of engineering elements in automotive motion is more than mere mechanics; it embodies advanced technologies and essential principles that define vehicle dynamics."
Types of Propulsion Technologies
Understanding the various types of propulsion technologies is critical in comprehending how cars operate and move through their environment. These systems are the backbone of automotive dynamics, influencing performance, efficiency, and the environmental impact of vehicles. This section explores three primary propulsion technologies: internal combustion engines, electric vehicles, and hybrid systems. Each of these technologies has its unique characteristics and implications, making it essential for stakeholders in the automotive space to grasp their nuances.
Internal Combustion Engines
Internal combustion engines (ICE) continue to dominate the automotive market, despite the rise of alternative technologies. ICEs work by converting fuel into mechanical energy through a series of controlled explosions in cylindrical chambers. The core components include the engine block, pistons, crankshaft, and valves. The design of these engines allows for robust power generation, making them suitable for various applications.
However, ICEs have significant drawbacks. They emit greenhouse gases and pollutants that contribute to climate change and affect air quality. Furthermore, they are inherently less efficient than electric counterparts. Efforts to improve fuel economy and reduce emissions have led to innovations such as turbocharging and direct fuel injection, yet the fundamental problems persist. The future of ICEs lies in their adaptation to stricter emissions standards and the integration of cleaner fuels.
Electric Vehicles and Their Mechanisms
Electric vehicles (EVs), powered by electricity stored in batteries, represent a paradigm shift in automotive propulsion. Unlike ICEs, EVs produce zero tailpipe emissions, making them a cleaner option for urban environments. They consist of crucial components like electric motors, battery packs, and regenerative braking systems. The increasing efficiency of electric motors and improvements in battery technology, particularly with lithium-ion cells, have led to greater driving ranges and quicker charging times.
One important aspect of EVs is their potential for reducing dependency on fossil fuels. As energy grids increasingly incorporate renewable energy sources, the environmental benefits of EVs are likely to grow. However, challenges remain. Battery production is resource-intensive and often involves materials that raise ethical and environmental concerns. Furthermore, the charging infrastructure is still developing in many regions, hindering widespread adoption.
Hybrid Systems: A Combination Approach
Hybrid systems aim to combine the benefits of internal combustion engines and electric motors. These vehicles typically use an ICE alongside an electric motor and battery storage. This integration enables hybrids to provide greater fuel efficiency and reduced emissions compared to traditional ICE vehicles. There are different types of hybrid configurations, including series, parallel, and plug-in hybrids, each with specific advantages in terms of power and efficiency.
Hybrids can function using multiple power sources, which allows for versatility in various driving conditions. For instance, during urban driving, the electric motor can handle most of the propulsion, while the ICE kicks in during highway driving for improved efficiency. Nevertheless, hybrids also face challenges similar to those of both ICEs and EVs. The complexity of the systems can lead to higher maintenance costs, and the need for proper waste management of batteries remains a pressing issue.
Hybrid systems exemplify the transitional phase of automotive technology, balancing traditional and innovative approaches to propulsion.
Understanding these propulsion technologies is vital as the automotive industry continues to evolve. As environmental regulations become more stringent and the demand for cleaner transportation solutions increases, the significance of these systems will only grow. The landscape of automotive propulsion is changing, influencing research, development, and consumer choices in a more environmentally conscious direction.
The Role of Technology in Automotive Motion
Technology plays a vital part in how automotive motion is understood and implemented in the contemporary world. As demands for performance, safety, and efficiency increase, automotive technology continues to adapt. Innovations not only enhance vehicle operation but also influence how we think about mobility and environmental responsibility. Understanding the role of technology helps us appreciate the complexity of modern vehicles and the ways they are becoming integrated with smart infrastructure and sustainable practices.
Advancements in Computer-Aided Design
Computer-Aided Design (CAD) has revolutionized how automotive engineers approach vehicle development. CAD tools allow for precise modeling and simulations, enabling engineers to visualize components before any physical prototype is created. This digital innovation improves efficiency during the design phase, allowing for faster iterations and refinements.
Key benefits of CAD in automotive motion include:
- Increased accuracy in measurements and design specifications.
- Enhanced collaboration across different engineering teams through shared digital models.
- Cost reduction by minimizing the need for physical prototypes.
As CAD technology advances, integration with other software remains essential. This integration allows for aerodynamic testing and structural analysis in a virtual environment, leading to more fuel-efficient and safer vehicles.
The Impact of Autonomous Driving Systems
Autonomous driving systems represent one of the most significant technological leaps in the automotive industry. These systems utilize a combination of sensors, cameras, and algorithms to enable vehicles to navigate without human input. The potential impacts of this technology are profound, ranging from changes in road safety to shifts in urban planning.
Benefits include:
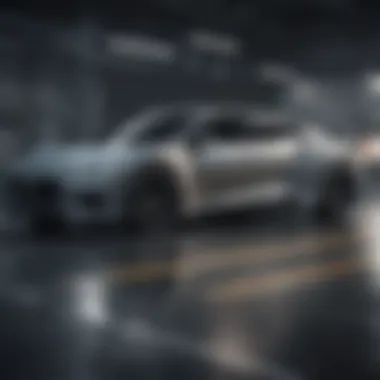
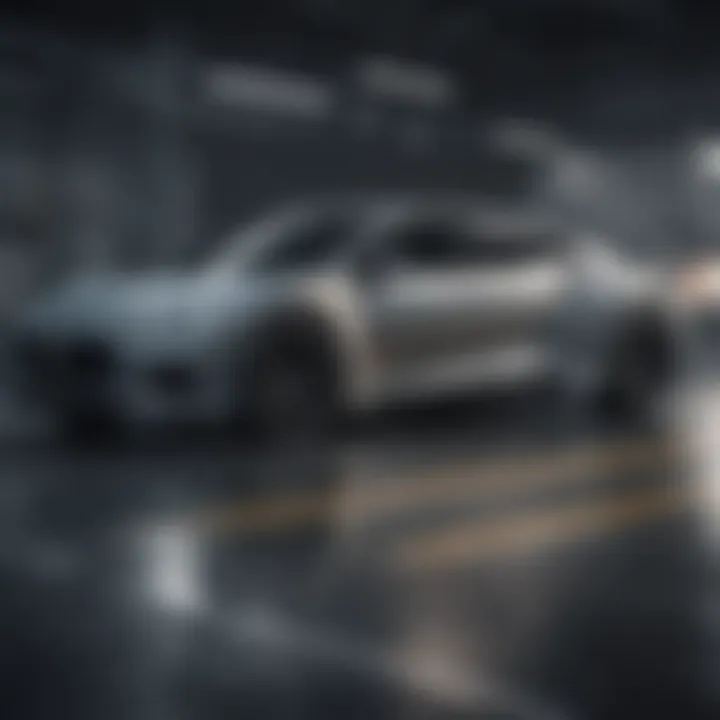
- Reduction in accidents due to human error, which is a leading cause of crashes.
- Increased mobility for individuals unable to drive, such as the elderly or disabled.
- Efficient traffic flow, reducing congestion and emissions through improved route management.
While the promise of autonomous driving is significant, challenges remain. These include regulatory hurdles, cybersecurity risks, and the need for public acceptance. Collaboration among technology developers, automotive manufacturers, and lawmakers is crucial to address these challenges.
Smart Technologies in Traffic Management
The integration of smart technologies into traffic management systems enhances how vehicles interact with their environment. Smart technology includes traffic signals that adapt based on real-time traffic conditions and vehicle-to-infrastructure communication. These advancements aim to improve overall traffic efficiency and safety.
Benefits of smart traffic management systems include:
- Reduced waiting times at intersections, saving fuel and decreasing emissions.
- Dynamic routing capabilities that help drivers avoid congestion.
- Improved emergency response through prioritized signal changes for emergency vehicles.
As urban areas grow, efficient traffic management becomes increasingly important. The use of data analytics and artificial intelligence can further optimize these smart systems, enabling cities to modernize their infrastructure effectively.
"The application of technology in automotive motion is not merely a trend but a necessary evolution toward a more integrated and efficient transportation system."
In summary, the role of technology in automotive motion encompasses a variety of advancements that not only improve vehicle functionality but also address broader societal needs. From CAD's impact on design to the near-future potential of autonomous systems and smart traffic management, technology shapes our perception of mobility and its implications.
Environmental and Societal Considerations
Environmental and societal considerations are becoming increasingly crucial in the context of automotive motion. As car usage expands globally, it brings along a series of implications that affect both the environment and society at large. This section will explore carbon emissions and their impacts, sustainable transport future, and the role of public policies and automotive regulation in shaping the field.
Carbon Emissions and their Impacts
Carbon emissions from vehicles contribute significantly to air pollution and climate change. When cars burn fossil fuels, they release carbon dioxide and other greenhouse gases into the atmosphere. These emissions can lead to various health issues such as respiratory diseases and cardiovascular problems among the population.
Moreover, excessive carbon emissions exacerbate global warming, leading to extreme weather events, rising sea levels, and damage to ecosystems. According to the International Energy Agency, road transportation accounted for about 24% of global CO2 emissions from fossil fuel combustion in 2019. This statistic underscores the pressing need for strategies to reduce automotive emissions.
The automotive industry's shift to electric and hybrid vehicles helps address these challenges. By transitioning to cleaner energy sources, emissions can be reduced, improving air quality and contributing to a healthier environment. However, it is crucial to ensure that the electricity used for these vehicles comes from renewable sources to maximize the benefits.
The Future of Sustainable Transport
As concerns over climate change and environmental degradation grow, the future of sustainable transport becomes a vital topic. There is a strong drive towards developing transport systems that minimize the ecological footprint while meeting the mobility needs of society.
Key aspects of sustainable transport include:
- Promoting Electric Vehicles: Increasing the adoption of electric vehicles can significantly reduce emissions.
- Encouraging Public Transport: Investing in robust public transportation systems assists in decreasing the number of individual cars on the road.
- Integrating Alternative Modes: Options such as cycling and walking need emphasis as they are eco-friendly methods of transportation.
Additionally, innovative technologies like autonomous driving could further enhance efficiency by optimizing traffic flow and reducing congestion. Better traffic management can lead to shorter travel times and reduced emissions.
Public Policies and Automotive Regulation
Public policies play a pivotal role in guiding the automotive industry towards environmentally friendly practices. Government regulations can set the standards for emissions, fuel efficiency, and safety. As we move into an era of stricter climate policies, automakers must adapt to stay compliant and to gain a competitive advantage.
Some important regulatory measures include:
- Emission Standards: Governments may impose limits on the amount of CO2 vehicles may emit. This encourages manufacturers to innovate towards low-emission technologies.
- Subsidies for electric vehicles: Financial incentives help promote the adoption of cleaner vehicles among consumers.
- Urban Planning Regulations: Smart urban planning can reduce reliance on cars by enhancing public transport and non-motorized transport networks.
By understanding the implications of vehicle motion on the environment and society, stakeholders can drive the automotive industry toward a more sustainable future.
Ending
The topic of automotive motion is critical in understanding the principles that enable vehicles to function efficiently in a complex world of ever-evolving technology. This article highlights the key components that influence how cars operate, from basic physical laws to advanced engineering solutions.
Summarizing Key Insights
Throughout this discussion, several key insights emerge. Understanding Newton's laws of motion is fundamental; they govern vehicle acceleration, speed, and deceleration. Moreover, the role of friction and traction is crucial for safety and performance. The engineering aspects explore the intricate design of powertrains and transmissions, showcasing their importance in delivering power from engines to wheels effectively. Furthermore, the evolution of propulsion technologies, including internal combustion engines and electric systems, reflects the move towards more sustainable practices in automotive design. The integration of smart technologies has also dramatically transformed traffic management, enhancing safety and efficiency.
"Innovations in automotive engineering showcase a constant pursuit of improved performance and sustainability."
Future Directions in Automotive Research
As we look to the future, automotive research is shifting towards several promising areas. Autonomous vehicle technology presents challenges and opportunities related to safety and urban infrastructure. The potential of hydrogen fuel cells combined with electric batteries indicates a forward trajectory in propulsion technologies.
Sustainability is becoming an urgent focus, guiding research into more efficient energy management and material use. Public policy will shape the deployment of these technologies, as regulations can greatly influence innovation. Continued research into consumer behavior will also be vital, helping to align technological advancements with public acceptability.