Dynamics of the Protein Interactome and Its Importance
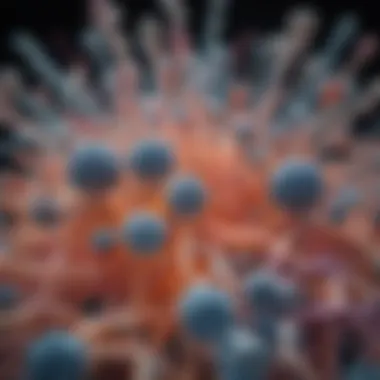
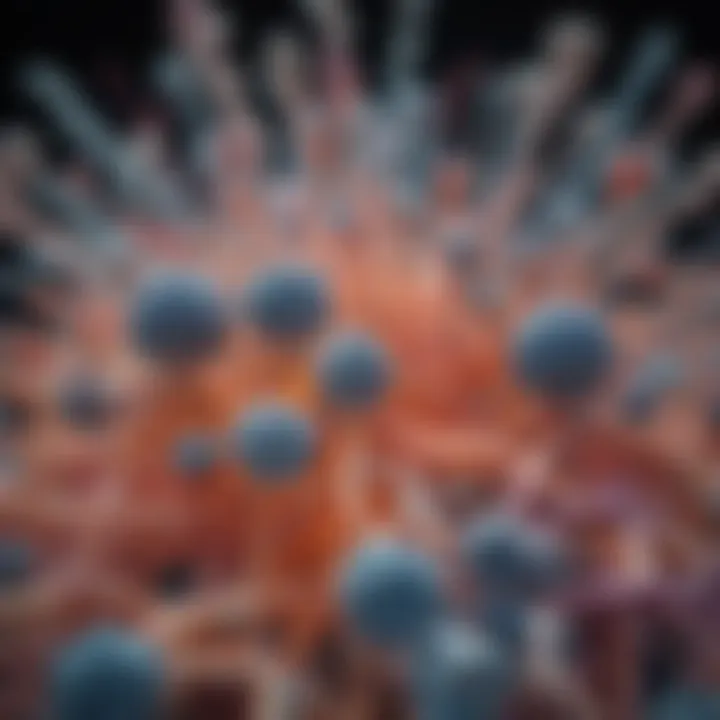
Intro
The protein interactome represents a detailed and interconnected landscape of molecular relationships shaping the behavior of cells and even entire organisms. At its core, the interactome comprises an extensive range of protein interactions that govern fundamental biological processes. Each interaction is a thread in the intricate tapestry of life, illustrating how proteins communicate and collaborate to facilitate cellular function.
Understanding the protein interactome is not merely academic; it holds real-world implications for advances in medicine, biotechnology, and our grasp of many diseases. With increasing sophistication in research methodologies, we are now able to map these interactions with greater precision. The surge of knowledge regarding protein networks has opened new avenues in drug discovery, highlighting where targeted treatments can be developed.
This exploration is timely and essential, especially given the current push in scientific research towards uncovering the mystique behind cellular dynamics. By investigating the various facets of the protein interactome, we not only illuminate the pathways of cellular communication but also set the stage for potential therapeutic interventions in disease pathology.
Understanding the Protein Interactome
In the landscape of molecular biology, understanding the protein interactome is akin to having a map in an unchartered territory. It's not just about individual proteins acting alone; it's the intricate dance of interactions that underlie cellular functions. Proteins rarely work solo; their interactions dictate a myriad of processes within the cells, influencing everything from metabolism to responses to environmental changes. Hence, comprehending this network of protein interactions becomes paramount, especially in the realms of health and disease. Through this exploration, we peel back the layers of complexity surrounding these interactions, revealing not only their nature but also their profound implications.
Definition and Scope
The term "protein interactome" refers to the complete set of molecular interactions involving proteins within a cellular context. This includes direct binding interactions between proteins themselves, but it also extends to interactions with other biomolecules, such as nucleic acids and small metabolites. To illustrate, consider a bustling cityāeach protein is a building, while the interactions among them form the roads connecting these structures. Understanding the interactome is like grasping the cityās layout and how transit routes (interactions) impact the overall function.
Mapping the protein interactome isn't straightforward. It requires a variety of techniques and methodologies, each providing a piecemeal glimpse into the overall picture. The scope spans various dimensions, including the quantitative assessment of interaction strengths and characterizing the dynamic nature of these interactions under different biological contexts.
"The significance of the interactome lies not only in its components but in the relationships formed among these entities."
Historical Context of Protein Interaction Studies
Looking back, the journey of studying protein interactions is quite fascinating. The early days were rudimentary; scientists relied on biochemical methods, many of which were labor-intensive and fraught with limitations. Over time, as technology advanced, more sophisticated methods like yeast two-hybrid screening emerged, revolutionizing the field. This method allows for the detection of potential protein interactions within a living organism, essentially giving a voice to the silent connections that lay dormant in cells.
The adoption of mass spectrometry in the 1990s marked another significant milestone. This powerful tool enabled researchers to not only identify proteins in complex samples but also detail their interactions with high specificity. With this technological progress came the realization that protein interactions are not static. They are highly dynamic and subject to change in response to various stimuli. This revelation prompted scientists to reconsider their approaches, advocating for studies that encompass temporal variations of protein interactions.
As the field has matured, it has led to numerous databases compiling known interactions, serving as invaluable resources for researchers. Moreover, contemporary investigations increasingly incorporate computational models alongside experimental methods to further elucidate the complexities of the protein interactome. The interconnections among proteins, much like the intricate ties in human relationships, deserve deeper understanding, especially as we navigate the interface of health and disease.
The Importance of Protein Interactions
Understanding protein interactions is crucial because these interactions underpin nearly all biological processes. Proteins do not function in isolation; they are part of a highly intricate network, collaborating to drive various cellular activities. Grasping the importance of these interactions aids in grasping how living systems operate at a molecular level.
In cellular biology, protein interactions contribute significantly to the organization and regulation of cellular activities. Each protein has a specific role, often relying on its ability to interact with other proteins to effectuate its function. This collaborative nature ensures a finely-tuned system where processes such as metabolism, cell signaling, and gene expression occur seamlessly.
For instance, when one protein binds to another, it can trigger a series of events that facilitate processes, such as the cell cycle, differentiation, and response to environmental cues. Without these interactions, cellular machinery begins to break down, leading to dysfunction. Therefore, exploring the nature and consequences of these interactions is fundamental both for basic research and for applied sciences.
"Understanding the delicate balance and interdependence of protein interactions is key to unlocking many biomedical mysteries."
Roles in Cellular Functions
The roles that protein interactions play in cellular functions are vast and multifaceted. They help form dynamic complexes that carry out essential cellular tasks. For example, enzymes require specific substrates to facilitate biochemical reactions, which often involves interactions with other proteins, sometimes referred to as cofactors. These interactions guide the biochemical pathways that in turn influence cell targeting, energy production, and various metabolic functions.
In cellular signaling, protein interactions act as a relay system, transmitting information from one part of a cell to another. A classic example can be found within signaling pathways such as the MAPK/ERK pathway, where sequential protein interactions help propagate signals from extracellular stimuli to cellular responses. Here, the intricate dance between proteins ensures that the cells appropriately respond to changes in their environment, maintaining homeostasis and facilitating adaptation.
Moreover, protein interactions foster the assembly of structural complexes, such as cytoskeletal formations that maintain cellular integrity and support intracellular transport mechanisms. For instance, actin and tubulin proteins interact to create the cytoskeleton framework, essential for shape and movement, both in single cells and throughout multicellular organisms.
Influence on Signal Transduction
Signal transduction is one of the critical areas where protein-protein interactions shine. When a signaling molecule, like a hormone or neurotransmitter, binds to its corresponding receptor protein on the cell surface, a cascade of interactions within the cell is set in motion. This initial interaction can spark a sequence involving multiple networks of proteins, leading to diverse outcomesāranging from gene expression changes to metabolic shifts.
For example, in the case of insulin signaling, the interaction between the insulin receptor tyrosine kinase and downstream signaling proteins, such as insulin receptor substrates, is pivotal. It can lead to glucose uptake and promote glycogen synthesis. Disruption in these interactions can result in metabolic disordersāillustrating how critical such protein networks are in maintaining health.
The intricacies of these interactions are vast, often involving phosphorylation modifications that temporarily alter the protein's shape or function, allowing for precise control over cellular responses. Understanding these dynamics can open doors to targeted therapies designed to amend the dysregulations often seen in diseases.
Implications for Disease Mechanisms
Protein interactions do not just dictate normal cellular processes; they are at the heart of many disease mechanisms. Alterations or aberrations in protein interactions can lead to dysfunction within cellular pathways, resulting in diseases ranging from cancer to neurodegenerative disorders.
For instance, in cancer, mutations in oncogenes or tumor suppressors can skew normal signaling pathways, primarily when they disrupt the interactions between key regulatory proteins. These changes can facilitate unchecked cellular proliferation, evasion of apoptosis, and metastasis. Thus, unraveling these protein interaction networks becomes essential for understanding tumor biology and developing therapeutic interventions.
Similarly, in neurodegenerative diseases such as Alzheimer's, misfolded proteins can interact inappropriately, forming aggregates that disrupt normal cellular functions. Recognizing how these protein interactions contribute to disease allows for innovative approaches in drug development, including targeting the specific interactions that lead to pathological states.
Methodologies for Mapping the Protein Interactome
Understanding the protein interactome is complex and multifaceted. A major piece of this puzzle lies in the methodologies we employ to decipher these intricate relationships. Mapping the protein interactome is essential not only for characterizing how proteins interact but also for unmasking the functional significance of these interactions. Each methodologyābe it experimental or computationalābrings to light different aspects of protein dynamics, paving the way for depths of understanding in both basic research and applied sciences.
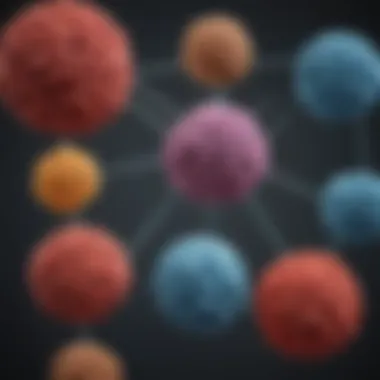
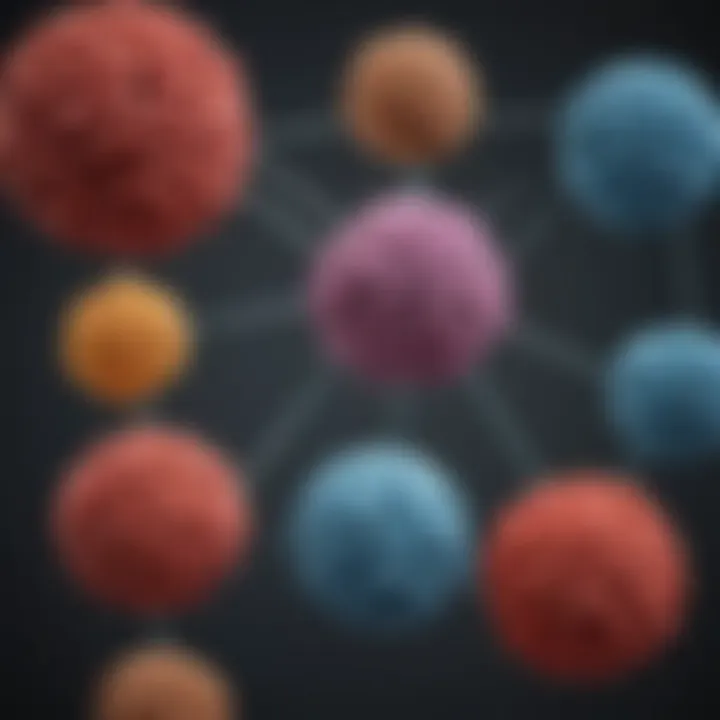
Experimental Techniques
Yeast Two-Hybrid Screening
Yeast Two-Hybrid Screening is a widely embraced experimental strategy that allows scientists to explore protein-protein interactions within a living organism on a scale that is often unimaginable with other methods. The strength of this technique lies in its simplicity and effectiveness: it relies on transcriptional activation through the interaction of two proteins tagged to distinct domains. Only when these proteins come together in the yeast cells do they activate reporter genes, resulting in measurable outcomes. This key characteristic makes it a valuable method for initial screenings of potential interaction partners.
A unique feature of Yeast Two-Hybrid Screening is the capability to screen large libraries of proteins quickly. It enables researchers to identify a variety of interactions efficiently. However, itās important to note that the technique has its limitationsāfalse positives can occur, and not all interactions may be captured due to the complexity of cellular environments. Still, for many, it's a popular choice for generating hypotheses in protein interaction studies.
Co-Immunoprecipitation
Co-Immunoprecipitation (Co-IP) stands as a robust experimental procedure used in protein interaction studies. This method is celebrated for its straightforward premise: it utilizes specific antibodies to pull down a target protein along with its binding partners from a complex mixture like cell lysates. This enables researchers to study cellular contexts closely, and this characteristic makes it beneficial for verifying interactions discovered by high-throughput techniques.
Co-IP allows for a more natural representation of protein interactions within their biological context, accommodating post-translational modifications that could affect interactions. The unique feature here is the specificity afforded by antibodies; they ensure that interactions are observed with a high degree of reliability. Nevertheless, Co-IP is not without challenges, as optimizing conditions can be time-consuming and yield variable results depending on the proteins involved, making it critical to refine protocols with each specific protein pair.
Mass Spectrometry
Mass Spectrometry (MS) serves as a powerful analytical tool that can provide insights into protein-protein interactions at a level of detail that is unmatched when compared to other methods. Its key characteristic lies in its ability to identify and quantify the proteins present in a sample simultaneously. Mass Spectrometry can analyze complex mixtures and reveal not just interactions but also the stoichiometry and dynamics of these molecular relationships.
A unique feature of Mass Spectrometry is the capacity to describe post-translational modifications, offering a nuanced view of how protein interactions can change under different conditions. This depth makes it a beneficial tool for studying interactomes in various contexts, whether in response to stress or during specific cellular events. However, the interpretation of data can be challenging. The complexity of the interactions and the intricacies of the resulting spectra sometimes demand sophisticated bioinformatics knowledge to untangle and make sense of the findings.
Computational Approaches
Advancing our understanding of the protein interactome also necessitates a shift towards computational methods. These approaches enrich the experimental landscape by providing tools that predict, analyze, and visualize protein interactions within large datasets.
Bioinformatics Tools
Bioinformatics Tools have revolutionized how researchers approach the mapping of protein interactions. These tools allow for the integration and accessibility of vast amounts of biological data, enabling scientists to predict potential interactions even before conducting experimental assays. A distinguishing characteristic of these tools is how they leverage existing databases, employing algorithms to infer interactions based on various biological data types, like genomic sequences and expression profiles.
Additionally, they provide a beneficial platform for exploring large networks, offering a computational lens through which complex biological interactions can be visualized more straightforwardly. Yet, it's crucial to remain cautious; the predictions made by bioinformatics tools must still be validated experimentally, as computational models can only go so far.
Network Analysis Software
Network Analysis Software is pivotal in understanding the broader implications of protein interactions. This software allows scientists to construct, analyze, and visualize protein interaction networks, yielding insights that might not be immediately apparent from isolated studies. The key characteristic of this software is its ability to reveal structural properties and network dynamics, enhancing our understanding of functional modules within the interactome.
A unique and valuable aspect of Network Analysis Software is the facilitation of community detection, identifying clusters of interacting proteins. This can provide crucial insights into cellular pathways and functions. Nevertheless, the inherent complexity of biological systems means that interpreting these networks can often be an uphill battle, requiring careful consideration of the biological context in which these interactions exist.
Types of Protein Interactions
Understanding the different types of protein interactions is crucial for grasping the nuances of cellular function and dynamics. Not all protein interactions are created equal; they vary significantly in their stability, duration, and molecular implications. This section seeks to illuminate the distinctions between these types and provides a deeper look at their roles within the protein interactome.
Transient vs. Stable Interactions
In the realm of protein interactions, transient and stable interactions play vastly different roles, each with its own set of characteristics and functionalities.
Transient interactions are often short-lived. These fleeting connections are essential for processes like signal transduction, where proteins need to interact temporarily to relay messages within the cell. Think of them like a handshakeāitās quick, but it establishes a connection that can have significant repercussions. They generally involve lower binding affinities, making them suitable for dynamic processes that require rapid alterations in protein activity.
On the other hand, we have stable interactions, which are longer-lasting and typically occur between proteins that form part of larger complexes. These interactions provide structural integrity, such as in the case of cytoskeletal proteins, or they might stabilize enzymatic functions critical for metabolic pathways. Imagine these stable interactions like a long-term partnership; theyāre built to last, ensuring that the complex functions effectively and predictably over time.
When dissecting protein interactomes, recognizing the balance and interplay between transient and stable interactions is fundamental. Itās like observing a dynamic dance; both partners contribute uniquely, dictating the overall performanceāone guiding the rhythm, while the other anchors the choreography.
Homooligomeric and Heterooligomeric Complexes
Protein assemblies often fall into two main categories based on their composition: homooligomeric and heterooligomeric complexes. These terms might sound complex, but they simplify our understanding of protein partnerships.
Homooligomeric complexes consist of identical protein subunits. An example of this would be the hemoglobin protein in red blood cells. It is made of identical units and plays a critical role in oxygen transport. These homooligomers are vital in cases where uniformity is required for function and stability.
Conversely, heterooligomeric complexes encompass different protein subunits. This diversity allows for a more intricate network of interactions, where each unique unit contributes a specific function. These complexes underline many biological processes, such as the assembly of G-protein coupled receptors, which facilitate countless signaling pathways. This heterogeneity is akin to a well-cooked dish, where various ingredients mingle to achieve a richer flavor profile.
Thus, understanding these complexes aids in elucidating the responsibilities of individual proteins in larger functional assemblies.
Receptor-Ligand Interactions
Diving deep into receptor-ligand interactions reveals a world where specificity is paramount. These interactions are crucial in cellular communication and often serve as the first step in signal transduction pathways.
The basic premise of this interaction involves a receptorātypically a protein located on the cell surfaceāand a ligand, which could be a hormone, neurotransmitter, or any other signaling molecule. When a ligand binds to its respective receptor, it triggers a conformational change that activates a cascade of intracellular events. This process is as precise as a lock and key: only the right ligand can fit into its designated receptor, ensuring that signals are correctly transmitted within cells.
Such specificity allows cells to respond appropriately to diverse environmental cues, which is critical for homeostasis and adaptive responses. The regulatory nature of these interactions underscores the importance of studying them in the context of drug developmentāmany therapeutic strategies aim to mimic or block these receptor-ligand interactions to treat various diseases. Understanding how these interactions operate at a molecular level can open the door to innovative treatments and targeted therapies.
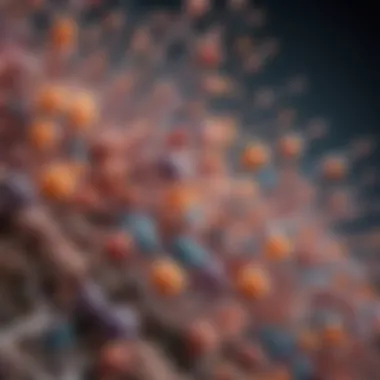
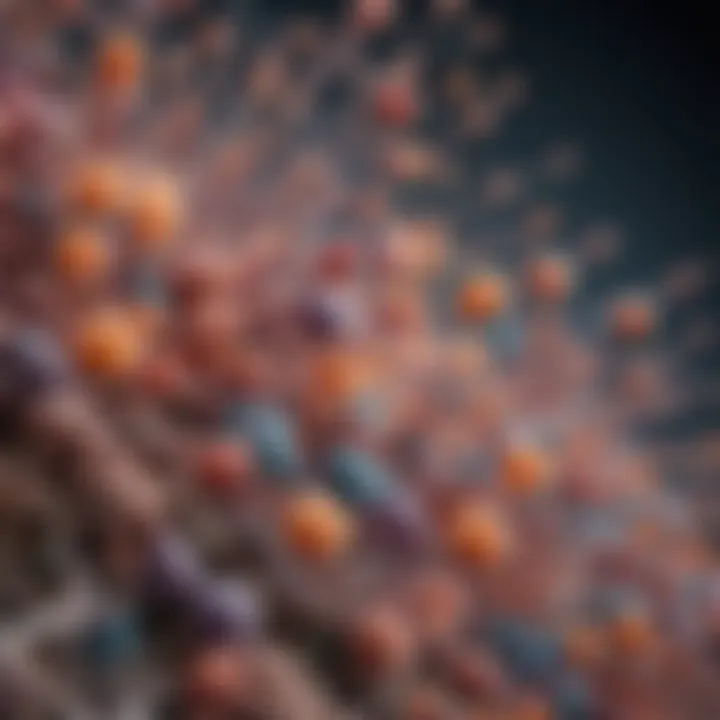
"Receptor-ligand interactions are the gateways to cellular responses, illustrating how specificity can dictate the fate of a cell."
In summary, examining the types of protein interactions expands our comprehension of cellular mechanics and informs future directions in research and potential therapeutic avenues.
Tools and Technologies in Protein Interaction Studies
Understanding the tools and technologies used in protein interaction studies is essential for grasping how scientists can map the protein interactome effectively. These tools not only provide insights into the intricate webs of protein interactions but also enhance the precision of experimental results. Each technological advancement carries a host of benefits, considerations, and potential pitfalls that researchers need to navigate through.
The application of advanced methodologies has dramatically affected the scope and depth of our understanding of protein interactions. Modern techniques enable researchers to analyze protein behavior in real time, thus uncovering dynamic interactions that were previously elusive.
Next-Generation Sequencing
Next-Generation Sequencing (NGS) has fundamentally transformed the field of protein interaction analysis. It allows for the simultaneous sequencing of millions of DNA fragments, thereby pinpointing variations that might play a role in protein interactions. The main advantage of NGS lies in its high throughput and accuracy, enabling researchers to obtain large amounts of data quickly.
In practical terms, NGS can assist in characterizing binding affinities and protein complexes, allowing for a comprehensive view of how proteins operate within a biological framework. Notably, the integration of NGS with other methodologies enables enhanced resolution of protein interaction maps, assisting scientists in discerning causal relationships in complex biological scenarios.
However, researchers must also be wary. The flood of data generated by NGS can be overwhelming, making it imperative to have robust bioinformatics tools in place to interpret results accurately. Additionally, high costs associated with NGS platforms can pose a challenge for some research institutions.
CRISPR-Based Screening
CRISPR-based screening is another cutting-edge tool that has gained considerable traction in the realm of protein interaction studies. This technology, originally developed for genome editing, allows scientists to create targeted disruptions or modifications in genes, assessing the consequent changes in protein interactions. The advantage of CRISPR lies in its precision; it allows for the specific targeting of proteins of interest, offering insights into their function and interaction edges.
Utilizing CRISPR in screening can yield high-throughput results, streamlining the process of identifying protein partners and understanding cellular pathways. For instance, targeted gene knockouts using CRISPR can elucidate the roles of specific protein interactions in various signaling pathways.
Nonetheless, itās crucial to consider the off-target effects that CRISPR may introduce, which could lead to confounding results. Thus, validating findings from CRISPR screens with additional techniques is both prudent and necessary.
Single-Cell Analysis Techniques
Single-cell analysis techniques are an emerging frontier in the study of protein interactions, allowing for the examination of individual cells rather than relying on averaged results from bulk samples. This method reveals the heterogeneity in protein expression and interactions among seemingly identical cells.
Such specificity is invaluable when studying complex tissues or during states such as disease progression. With single-cell RNA sequencing, researchers can unravel the intricate tapestry of protein interactions on a cell-by-cell basis, providing a more nuanced view of biological processes.
Moreover, these techniques can be coupled with imaging technologies to visualize protein interactions in live cells, further enriching the insights garnered. However, the technical challenges and costs associated with single-cell methods are considerable, requiring not only meticulous handling of samples but also advanced computational tools to analyze the resulting data.
"Single-cell technologies represent a leap towards understanding the granular relationships in biological systems, which is often masked in bulk analysis."
In summary, the tools and technologies used to study the protein interactome are varied and powerful. Each tool, from NGS to CRISPR-based screening and single-cell analysis, offers unique insights, but they come with their own set of challenges. Therefore, a thorough understanding of these methodologies is paramount for researchers aiming to draw meaningful conclusions in the dynamic field of protein interactions.
Implications for Drug Development
Understanding the protein interactome holds significant weight in drug development, mapping a crucial pathway that intertwines cellular mechanisms with therapeutic strategies. The interactions that proteins engage in are not just academic curiosities; they are the very fabric of biological function. Dissecting these associations allows researchers to foresee how drugs might behave in a biological context, unveiling both their potential efficacy and their pitfalls.
Target Identification
Target identification is the bedrock of rational drug design. It begins by pinpointing specific proteins or complexes that are involved in disease pathways. Hereās where knowledge of protein interactions becomes paramount. The idea is straightforward yet profound: the more we know about the relationship between proteins, the better we can identify which might serve as effective targets for drug action.
For example, consider cancer therapy. Oncogenic mutations often lead to aberrations in protein interactions, setting off a chain reaction that leads to tumorigenesis. In such cases, targeting a protein that has pivotal interactions with mutated forms can impede the growth of cancer cells. Notably, the identification process can leverage bioinformatics tools that analyze databases of known protein interactions, facilitating faster and more accurate identification of potential drug targets.
To flesh this out, targeted therapies such as trastuzumab, which is used against HER2-positive breast cancer, are prime examples. It works by binding to the HER2 protein, one that dabbles a lot in interaction webs, and mitigating its function.
Drug Repurposing Strategies
The innovative field of drug repurposing, or repositioning existing drugs for new therapeutic uses, thrives on insights gleaned from the protein interactome. Here, the knowledge of how various drugs interact with different protein networks can reveal unexpected therapeutic opportunities. In a climate where developing entirely new medications can be time-consuming and costly, repurposing is often viewed as a pragmatic approach.
For instance, the antimalarial drug chloroquine, originally designed for a specific application, has shown promise in other diseases by interacting with various protein pathways involved in inflammation and immune responses.
- Benefits of Drug Repurposing:
- Cost-Effectiveness: Using existing drugs can save time and resources required for clinical trials of entirely new drugs.
- Safety Profiles: Established medications often have known side effects and toxicity, which can reduce the risk when they are applied to new indications.
- Fast-track Development: With many repurposing paths, existing data can expedite the process of getting a drug into clinical practice.
The synergy between our understanding of protein dynamics and drug development strategies emphasizes the crucial intersection of biology and pharmacology. Not only does it provide a roadmap for future research, but it also engages the scientific community to look beyond conventional targets.
"The interplay of proteins is often a reflection of how diseases manifest and progressādeciphering this can open new avenues in drug development."
In summary, the implications of studying protein interactions extend beyond pure scientific curiosity; they carve out real-world applications that are transforming drug development. Moving forward, continued research and integration of protein interaction networks into drug design will undoubtedly usher in the next generation of therapies aimed at complex diseases.
Network Analysis of Protein Interactions
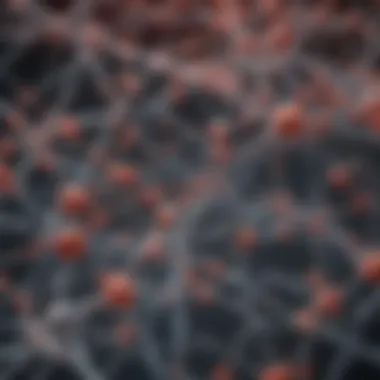
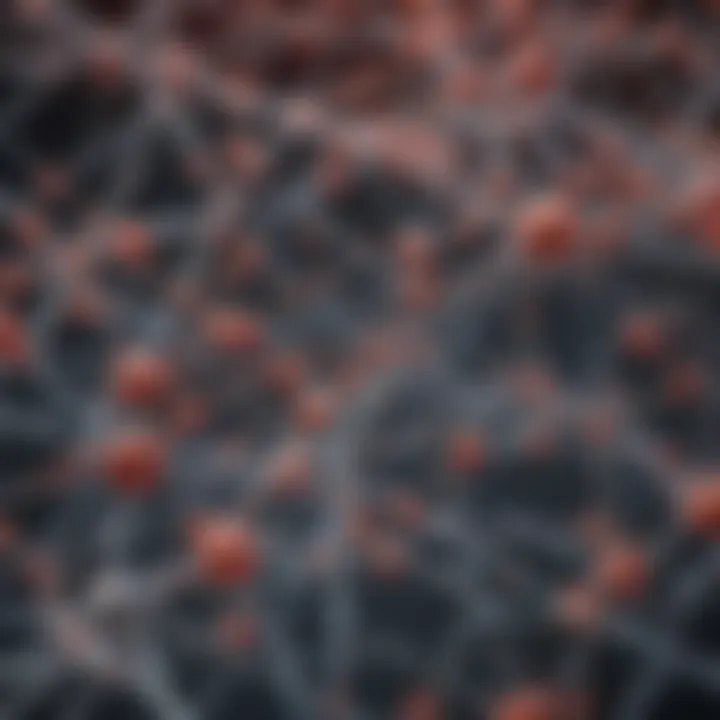
Understanding the complex landscape of protein interactions requires us to delve into network analysis. The significance of this topic lies in its ability to visualize and interpret the intricate web of interactions that proteins engage in within cellular environments. Given that proteins rarely act alone, instead forming networks that contribute to various biological functions, analyzing these relationships becomes crucial. Network analysis enables researchers to dismantle these connections, revealing insights that might otherwise remain obscured.
Using network analysis, scientists can identify key players within biological systems, often referred to as hubs. These hubs have the potential to exhibit higher degrees of connectivity, playing critical roles in maintaining cellular homeostasis. For instance, when investigating signal transduction pathways, identifying strategic nodes can expose vulnerabilities that may influence drug design or disease treatment.
Furthermore, visualizing protein interactions through network diagrams allows for a more intuitive understanding of how perturbations could propagate through the interactome. Factors such as biological variability, technical discrepancies, and environmental influences could alter these networks. Consequently, accounting for these aspects when performing network analysis becomes vital for obtaining reliable data.
"Without network analysis, the complexity of protein interactions might yield results that are less meaningful or actionable."
Graph Theory Applications
Graph theory serves as one of the cornerstones of network analysis in protein interactions. Each protein can be viewed as a node in a graph, while the interactions between them are the edges connecting these nodes. This modelling presents several practical applications, including the identification of important characteristics of the interactome, such as degree centrality, betweenness centrality, and clustering coefficients.
- Degree Centrality: This measures the number of connections a protein has. A protein with high degree centrality is crucial in maintaining the stability of the network.
- Betweenness Centrality: This indicates how often a protein acts as a bridge along the shortest path between two other proteins. Identifying these proteins can help understand pathways that are critical for cellular signaling.
- Clustering Coefficients: This allows researchers to understand how densely connected a subset of proteins is within the larger network.
By applying these principles, researchers can prioritize proteins for further functional studies or therapeutic targeting.
Community Detection in Protein Networks
Community detection refers to the process of identifying clusters or groups of proteins that interact more frequently with each other than with the rest of the network. This can be crucial in predicting the functional modules of a protein interactome. The idea is that proteins within these communities likely have related functions, providing insight into biological processes.
Several algorithms exist for community detection, including Girvan-Newman and Louvain methods. Each of these approaches employs different strategies to optimize how clusters are identified while accounting for the unique structure of the network. By recognizing these communities, researchers can form hypotheses regarding the underlying biology and explore therapeutic avenues that target specific groups of interactions.
In summary, network analysis not only unravels the dynamics of protein interactions but also provides the tools necessary to conduct impactful research in this field. As researchers continue to leverage technologies in network analysis, our understanding of the protein interactome will undoubtedly evolve, shaping future studies and potential applications in medicine.
Challenges in Studying the Protein Interactome
Understanding the complexities within the protein interactome requires grappling with various challenges. These challenges not only add layers of difficulty to research but also bring crucial insights that can significantly alter our approach to biology. The importance of addressing these challenges is paramount as they shape how we interpret protein interactions and their implications for cellular functions and disease mechanisms.
Technical Limitations
When it comes to technical barriers, researchers often encounter pitfalls that can obscure their findings. For instance, the sensitivity and specificity of the techniques employed to map protein interactions can vary dramatically. Traditional methods like yeast two-hybrid screening may yield false positives or negatives due to the environment where the interactions take place. It's like trying to identify dancers in a crowded room but overlooking those just standing by the wall; sometimes the most crucial participants might be hard to spot.
Moreover, high-throughput technologies, while valuable, are not foolproof. Mass spectrometry, for example, excels in identifying proteins but can struggle with quantifying protein abundance accurately. Incorrect quantification can lead to misleading conclusions about interacting partners and their functional significance. In addition, the reproducibility of results across different experimental setups remains a glaring issueāresults can be as inconsistent as the weather.
Another aspect is the integration of data from various sources. Merging data sets from different laboratories can be like assembling a jigsaw puzzle where each piece seems to belong to a different picture. Disparities in methodologies and experimental conditions can create inconsistencies that complicate our understanding of the interactome.
Biological Variability
On the biological side of things, variability among biological samples can also pose a significant challenge. Individual differences in cellular environments, be it in culture conditions or organismal genetics, can influence protein interaction landscapes. This variability is akin to cooking; the same recipe can yield different results if the ingredients or cooking methods slightly change.
The dynamic regulations of protein interactions also contribute to this complexity. Proteins do not exist in a static state; they undergo modifications, some induced by environmental factors, which can alter their interaction profiles. For instance, phosphorylation can change a protein's shape, which may favor certain interactions under specific conditions. Thus, what may appear as a stable interaction could be far more transient than previously thought, making it imperative for researchers to consider context.
Finally, the challenge of studying protein interactions in in vivo systems, as opposed to cell cultures, exposes researchers to even more variability due to the organism's entire biological network influencing protein interactions. Just like planning a road tripāitās one thing to have a smooth drive on a test route, but quite another when you hit the actual highways filled with unpredictability.
"Navigating the intricacies of the protein interactome is akin to charting unknown waters; persistence and adaptability are key to uncovering the unseen currents of cellular interaction."
Ultimately, addressing these challenges provides invaluable opportunities for refining our methodologies and deepening our understanding of the protein interactome. It calls for interdisciplinary approaches that combine biochemistry, computational biology, and systems biology to dissect these complex webs of interactions effectively.
Future Directions in Protein Interaction Research
The exploration of the protein interactome continues to evolve, significantly influenced by technological advances and the growing understanding of cellular biology. Clarity in protein interactions opens up boundless avenues for pharmaceutical research and the mechanism of diseases, making this an increasingly vital area of focus. As new methodologies develop, researchers are not only enhancing their understanding of protein dynamics but also discovering novel applications in health and medicine.
Integration of Multi-Omics Approaches
Multi-omics is making waves in the scientific community, heralding a shift from traditional methods toward a more integrated view of biological data. This exciting direction involves combining various omics disciplinesāsuch as genomics, proteomics, transcriptomics, and metabolomicsāto create a more comprehensive landscape of cellular interactions. By fusing these layers of information, researchers can achieve a more holistic understanding of the complex cellular network.
- Benefits:
- Enhanced Accuracy: By utilizing different omics data, one can cross-verify protein interactions and ensure findings are not merely coincidental.
- In-depth Insight: Multi-omics facilitates a deeper understanding of the context and conditions under which protein interactions occur, influencing pathways and biological functions.
- Disease Mechanisms: Characters tied to specific diseases can be better understood by mapping interactions across various omic layers, potentially revealing targets for therapeutic interventions.
The inclusion of multi-omics approaches substantially aids in disentangling the intricate tapestry of proteins in various biological responses, illuminating the way forward for targeted digitized healthcare.
Predictive Modeling of Protein Interactions
In conjunction with advancements in data collection methods, predictive modeling has surfaced as a vital tool in protein interaction research. This technique leverages computational power to forecast potential interactions between proteins based on existing data. Utilizing algorithms and machine learning, these models can predict how proteins will behave in various conditions or environments.
- Significance:
- Streamlined Research: Predictive modeling alleviates the bottleneck in experimental validation by enabling researchers to focus on the most promising interaction candidates.
- Cost-Effective: It reduces the resources spent on high-throughput experiments, directing funding toward hypotheses that are most likely to yield significant findings.
- Real-time Scenarios: These models can help in simulating interactions under various stressors, providing insights into how proteins may behave within diseases or under therapeutic treatments.
The application of predictive modeling in protein interaction studies can drastically change the landscape, equipping researchers with the tools to foresee the consequences of proteinsā dynamics, rather than waiting to observe them.
In summary, future directions in protein interaction research stand at the intersection of innovative technologies and traditional biological inquiry. By embracing multi-omics and predictive models, scientists have the potential to unlock new insights into protein dynamics. This not only paves the way for advanced drug discovery but also enhances the understanding of underlying mechanisms of diseases, ultimately pointing us toward better health solutions.