Electric Vehicle Life Cycle Analysis: A Detailed Study
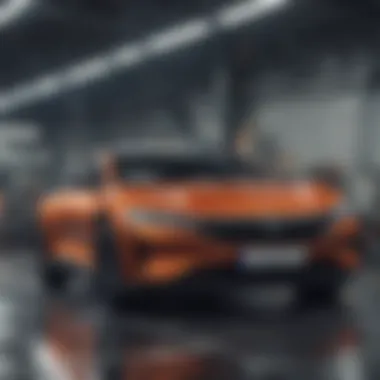
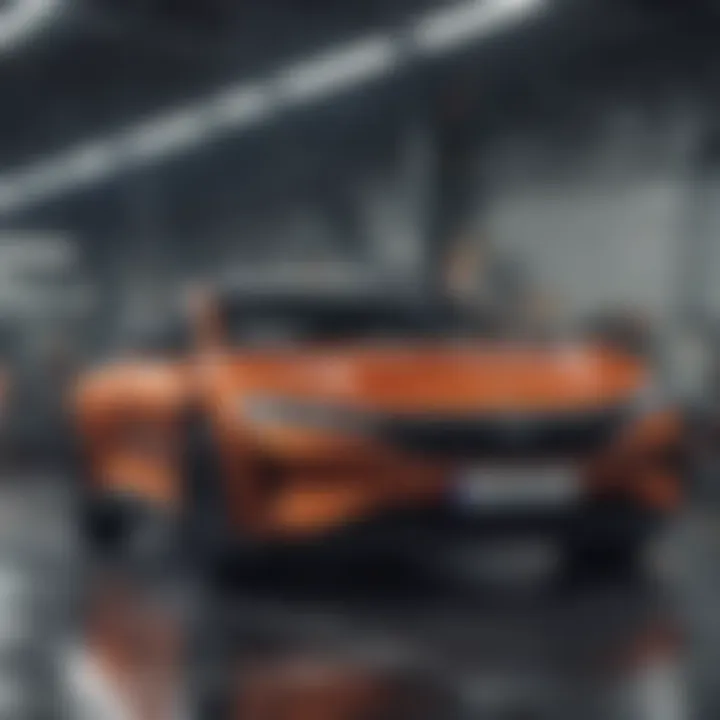
Intro
As society gears up for the electric revolution, the conversation about electric vehicles (EVs) encompasses deep-rooted considerations that go far beyond just how they perform on the road. While one might think about flashy new models or apps that track charge levels, there's a whole world of analysis happening behind the scenes—specifically, the life cycle analysis (LCA). LCA serves as a compass, guiding researchers, policymakers, and consumers alike through every phase of an electric vehicle's existence, from the material resources used in production to the emissions generated during its operational life and finally, the intricacies of end-of-life disposal.
Understanding the full life cycle of EVs sheds light on their environmental impacts, economic implications, and ultimately, their role in the quest for sustainability in transportation. By mapping out the entire process, stakeholders can work to refine existing practices and develop more sustainable solutions that align with the global push for reduced carbon footprints.
This comprehensive exploration offers a chance to dissect not just what makes EVs different, but also how traditional vehicles stack up against their electric counterparts in various metrics of sustainability. The knowledge unearthed throughout this article aims to serve as a vital resource for students, researchers, educators, and professionals navigating this dynamic field.
"To gauge the life cycle of electric vehicles is to understand their potential and limitations in driving global change toward greener transportation options."
The following sections will dive into specific aspects of LCA, including methodological approaches, current trends within scientific inquiry, and the overarching significance of this research for the future of electric mobility.
As we pivot to a more structured analysis, let’s explore the foundational research approaches that illuminate the knowledge surrounding electric vehicle life cycles.
Understanding Life Cycle Analysis in Electric Vehicles
Life Cycle Analysis (LCA) serves as the backbone of understanding the true environmental and economic implications of electric vehicles (EVs). It encompasses the entire journey of a product, from the cradle (raw material extraction) to the grave (end-of-life disposal). In the context of EVs, it's crucial for stakeholders to grasp not only the benefits associated with their use but also the broader impacts at every stage of the vehicle’s life.
The importance of LCA in electric vehicles cannot be understated. First and foremost, it provides a comprehensive framework to evaluate sustainability. For instance, an analysis doesn't stop at just emissions generated during driving. It digs deeper into how the raw materials are sourced, the energy consumed during manufacturing, the emissions related to electricity production for charging, and finally, how components are managed post-use.
Another significant element is the comparison between electric vehicles and traditional internal combustion engine vehicles. This assessment helps clear up the cloud of misconceptions that often accompany EVs. When one considers the entire life cycle, the environmental advantages may shift dramatically. In some cases, conventional vehicles might seem more favorable on a superficial examination; however, LCA sheds light on the hidden costs tied to fossil fuel use.
Benefits of Undertaking a Life Cycle Analysis
- Holistic Insights: LCA presents a detailed account of resource use and emissions across all phases.
- Informed Decision-Making: With accurate data, manufacturers, consumers, and policymakers can make educated choices regarding sustainability.
- Innovative Drivers: Understanding the impacts can spur technological improvements to minimize adverse effects and boost efficiency.
Yet, it’s not without its complexities. Achieving accuracy in LCA requires robust data collection and adept modeling. Variability in energy sources, differing regional practices, and evolving technologies can all influence the outcomes. Addressing these variables adds layers to the analysis but ultimately enriches the understanding of EV life cycles, making it a compelling and critical study area.
Definitions and Importance of LCA
Life Cycle Analysis, commonly referred to as LCA, is a systematic evaluation of the environmental impacts of a product through its entire life cycle. By considering each stage - from material extraction, manufacturing, distribution, usage, to disposal - LCA ensures a balanced view of the product's associated environmental footprint.
LCA plays a vital role in identifying areas for improvement to increase sustainability. For example, if one finds that the use of non-renewable materials during the manufacturing phase significantly correlates with emissions, this could initiate a search for alternatives.
Moreover, companies increasingly use LCA as part of Corporate Social Responsibility (CSR) strategies. Companies can enhance their market positioning by openly discussing their commitment to minimizing environmental footprints, thus appealing to a growing demographic of environmentally-conscious consumers.
Historical Context of LCA in Transportation
The concept of life cycle assessment in transportation is not a new phenomenon. The roots can be traced back to the 1960s and 1970s, coinciding with the rise in environmental awareness. Early efforts primarily focused on understanding the environmental impacts of traditional vehicles powered by fossil fuels.
As electric mobility began gaining traction in the late 20th century, the need to apply LCA to EVs emerged. Researchers recognized that while electric vehicles were touted for their reduced operational emissions, a complete picture required examining the entire lifecycle. For instance, the Tesla Roadster introduced in 2008 accelerated conversations around how the full life cycle of electric vehicles could be assessed, moving beyond just tailpipe emissions.
As methodologies for conducting LCAs improved over time, they began to influence policy frameworks and industry standards. Today, LCA has become integral in guiding the automotive industry towards greener practices, ensuring that the transition to electric mobility is both informed and sustainable.
The interplay between technological advancements and LCA findings leads us into a future where vehicles not only reduce emissions but are also crafted from environmentally-friendly materials, complementing efforts toward sustainability in the automotive sector.
Phases of Electric Vehicle Life Cycle
The life cycle of electric vehicles (EVs) is a multifaceted journey that extends far beyond the moment of ignition. Grasping the various phases of this life cycle is crucial for comprehending the total ecological and economic consequences of these vehicles. Each segment, from raw material extraction to end-of-life management, holds significance in determining the sustainability quotient of electric mobility. It’s not just about transitioning from gas to electric; it’s about evaluating every step in this cyclical process. This assessment allows for informed decisions on improving practices, reducing costs, and enhancing the overall beneficial impacts of EVs on our planet.
Raw Material Extraction
Mineral Resources and Battery Production
In the realm of electric vehicles, the battery is often viewed as the heart of the technology. To make these batteries, a slew of raw materials is needed, primarily lithium, cobalt, and nickel. These minerals are essential for developing efficient and long-lasting batteries. As the demand for EVs explodes, so does the need for sourcing these critical materials.
The key characteristic here is the intricate dance involved in extracting these minerals. Mining operations, whether done responsibly or otherwise, carry a weighty environmental footprint. On one hand, securing these materials is vital for creating energy-dense batteries, which in turn promotes the market growth of EVs. However, the often tough and sometimes hazardous conditions surrounding mining operations reveal the environmental and social challenges that come along with it.
Unique to this extraction phase is the heightened global scrutiny over ethical sourcing. Issues related to child labor in cobalt mines and ecological degradation in lithium extraction underscore the need for responsible practices. Thus, while the minerals are indeed a beneficial choice for advancing EV technology, their production poses significant ethical dilemmas.
Environmental Impacts of Mining
The environmental impacts of mining cannot be brushed aside. Each step in the extraction of materials required for battery production influences local ecosystems dramatically. The devastation of landscapes, loss of biodiversity, and pollution of water sources present pressing concerns. These activities contribute to habitat loss and can trigger health issues for nearby communities—as evident in several locations around the world.
This facet of mining is often perceived as a necessary evil in the pursuit of cleaner transportation. Current efforts try to mitigate these impacts through more responsible mining practices and advanced technologies. One such movement involves using less invasive extraction techniques and investing in reclamation projects to restore mined areas.
Ultimately, the unique feature of this topic is that while mining is indispensable for EV production, it must also evolve through stricter regulations and innovative practices that minimize its environmental toll. This creates a balance—advancing technologies without sacrificing ecological integrity.
Manufacturing Processes
Energy Consumption in Vehicle Assembly
Electric vehicle manufacturing is not just a straightforward assembly line; it encompasses complicated processes that demand significant energy. This energy consumption can vary widely based on the manufacturing facility’s operational efficiencies and methods. The production of parts such as motors and batteries alone requires substantial energy input.
Highlighting the nuances, manufacturing plants are increasingly implementing energy-efficient practices, aiming to reduce their carbon footprint significantly. For instance, some facilities are adopting renewable energy sources, thus contributing to a greener production phase. While these changes do require an upfront investment, the long-term savings in both energy costs and emissions present a strong case for their implementation.
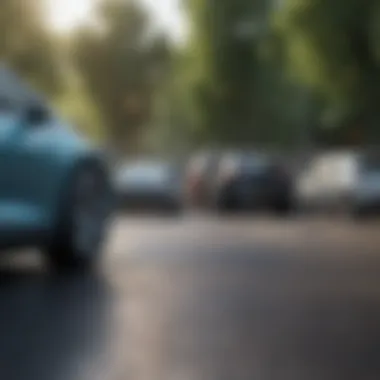
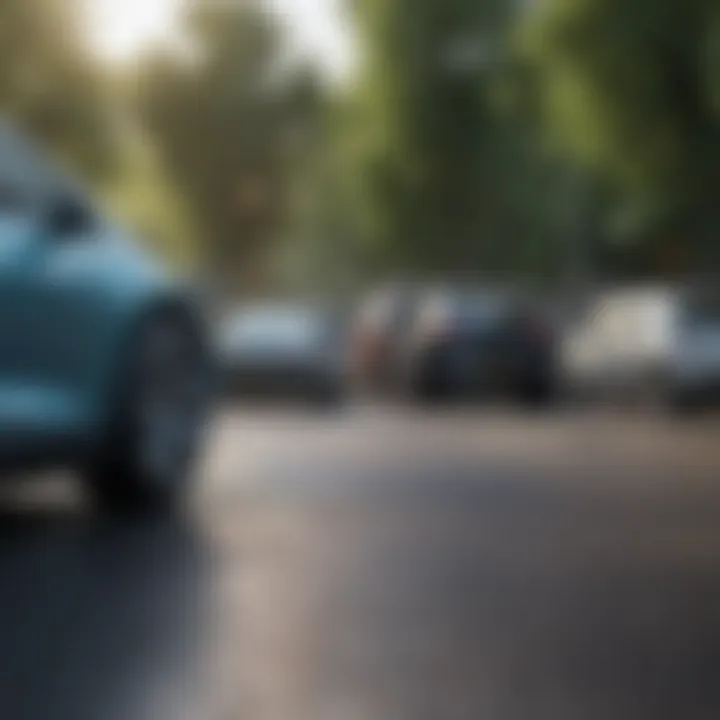
In this narrative, the unique feature lies in how energy consumption is being reframed—shifting from a necessary drawback to a critical aspect of sustainable production. Reduced energy use during assembly not only lowers costs but also aligns with the overarching goals of environmental stewardship for EV manufacturers.
Carbon Footprint of Manufacturing Facilities
Just as the energy consumption merits attention, so too does the carbon footprint present in manufacturing facilities. These factories, especially if powered by fossil fuels, can contribute a significant amount of greenhouse gas emissions. Understanding this aspect allows for a deeper comparison between electric and traditional vehicle production.
A noticeable characteristic of these manufacturing footprints is the potential for optimization. Manufacturers are increasingly aware of the need to minimize their contribution to climate change. Various plants are analyzing their operations to identify inefficiencies and transition to practices that lower emissions. This proactive approach illustrates the industry's recognition of its role in sustainable development.
For the average consumer, the unique feature is how they can select EVs not just on performance but also on the environmental stewardship of their manufacturers. By choosing companies that invest in sustainable practices, consumers are participating in a larger movement towards reducing the carbon footprint associated not just with vehicle usage but its entire life cycle.
Distribution and Transportation
Logistics of EV Delivery
As electric vehicles make their journey from the production line to consumers, logistics plays a pivotal role in ensuring efficiency. Coordinating the transportation of vehicles involves several layers, including warehousing, freight transport, and local delivery—often interwoven with supply chain dynamics that are quite intricate.
The spotlight here is on how these logistics contribute to the electric vehicle's overall sustainability. Poor logistics can lead to increased emissions as vehicles need to travel longer distances or be transported inefficiently. Conversely, companies that adopt streamlined logistics systems can dramatically cut down their carbon emissions. For instance, implementing local distribution hubs reduces the distance vehicles must travel, showcasing how operational efficiency can support larger sustainability goals.
This unique feature implies that logistics can act as either an Achilles’ heel or a boon for sustainability in electric vehicle delivery. Emphasizing efficiency in this area can greatly enhance the green credentials of EVs, pushing the industry further towards its environmental objectives.
Impact of Transportation on Emissions
Transportation’s impact on emissions is a crucial consideration in the discussion of electric vehicles. The journey to a consumer’s driveway doesn't come without consequences; the emissions from transporting vehicles can potentially negate the benefits attained in urban environments through lowered emissions during usage.
Examining this dilemma elucidates the importance of assessing the entire transportation network. By optimizing routes, using low-emission transport fuels, and focusing on regional manufacturing, companies can substantially mitigate the negative environmental impacts of moving vehicles.
The critical examination of this section unveils how intertwined EV logistics and emissions are. By emphasizing responsible transportation practices, the industry can enhance the overall lifecycle benefits of electric vehicles. This area thus presents both a challenge and an opportunity for advancement in sustainable practices within the sector.
Usage Phase
Energy Sources for Charging
When it comes to the lifespan of an electric vehicle, the phase where it is actually driven often garners the most attention. However, the source of energy for charging is a vital element of this equation. EVs may run on electricity, but the environmental benefit hugely depends on whether that energy comes from renewable or fossil-fuel sources.
A key characteristic of this situation is the potential disparity in emissions based on energy sourcing. Charging EVs using coal-powered plants largely undermines their sustainability claims, while using renewable energy sources like solar or wind can significantly enhance their positive impact. Thus, the energy source becomes a make-or-break aspect in evaluating the lifecycle emissions of electric vehicles.
A unique feature of charging infrastructure involves advancements and initiatives that encourage the use of green energy. Growing investments in solar charging stations are notable examples, where consumers can charge their vehicles using clean energy. Therefore, the charging aspect opens avenues for exploration within the industry that align consumer use with sustainable developments.
Driving Patterns and Efficiency
How a vehicle is driven can drive significant differences in energy consumption and emissions. Driving patterns, such as acceleration rates, braking behavior, and speed, influence the overall efficiency of electric vehicles. These elements are not merely trivial; they are fundamental to evaluating real-world usage impacts.
One interesting characteristic is how education regarding driving habits can serve as an immediate area for improvement. Initiatives that educate drivers on efficient driving can optimize energy usage. Insights tallying the efficiency gains drawn from smoother driving highlight a proactive approach individuals can adopt that translates into lower emissions.
Unique to this discussion is that while EVs generally perform well in efficiency, those benefits can skyrocket when drivers are attuned to maintaining efficient practices. Thus, driving behavior is not just a personal choice but a significant factor in maximizing the sustainability potential of electric vehicles.
End-of-Life Management
Recycling of Components
As electric vehicles reach the end of their usable life, the management of their components becomes a pressing issue. Recycling is not only a waste management process; it's an avenue for resource recovery that ensures valuable materials like lithium, cobalt, and plastic are re-entered into the supply chain.
What stands out about component recycling is its ability to close the loop in the lifecycle of electric vehicles. By effectively reclaiming these materials, the EV industry can potentially lessen the need for virgin resource extraction; this, in turn, minimizes the overall environmental impact. This aspect underscores the necessity for robust recycling systems and consumer awareness about recycling protocols.
However, a unique challenge lies in the complexity of recycling processes for batteries versus traditional automotive components. Although advancements are underway, processes are still developing for safe and efficient recycling of lithium-ion batteries, emphasizing the need for further research and investment.
Disposal Challenges and Solutions
As promising as recycling might seem, it does not erase the difficulties associated with end-of-life management. Battery disposal can pose significant environmental hazards if handled improperly. Dealing with hazardous materials and ensuring compliance with environmental regulations heightens the challenge.
The unique aspect here revolves around the importance of transparency in disposal. A well-defined process involving environmentally-friendly decommissioning methods plays a pivotal role in addressing these challenges. Encouraging manufacturers to build vehicles with end-of-life management in mind can also pave the way for innovations in disposal.
Identifying solutions such as legislative mandates, consumer education, and the establishment of dedicated recycling facilities can mitigate risks significantly. As such, the end-of-life stage becomes not just a challenge but an opportunity to rethink how to responsibly manage and repurpose critical components of electric vehicles.
Environmental Considerations
The exploration of Environmental Considerations within the realm of electric vehicles (EVs) is pivotal to understanding their broader implications for sustainability. This section highlights several key elements that underscore the environmental impact of EVs compared to traditional internal combustion engine vehicles. By focusing on greenhouse gas emissions and resource depletion, we can paint a more comprehensive picture of the ecological footprint that these vehicles leave behind.
Life Cycle Greenhouse Gas Emissions
Comparative Analysis with Conventional Vehicles
When placing electric vehicles side-by-side with conventional vehicles, the comparative analysis showcases several noteworthy differences in greenhouse gas emissions over their life cycles. A crucial aspect to consider is the emissions produced during the manufacturing phase of EVs versus that of traditional vehicles. While the production of electric vehicles involves substantial initial emissions—primarily tied to battery production—the operational phases sway the scales significantly. One key characteristic is that EVs generate zero tailpipe emissions, which dramatically cuts local pollutants contributing to urban smog.
Another unique feature of this analysis lies in the sourcing of energy used for charging. In regions powered predominantly by renewable sources, the overall emissions of EVs can be reduced further, making them a superior choice in the long run. The trade-off here is that in areas reliant on fossil fuels for electricity, the environmental benefits can diminish. Thus, it’s vital to indicate that the location of charging stations and energy mix directly affects the emissions profile of the EV.
Mitigating GHG Emissions through Innovation
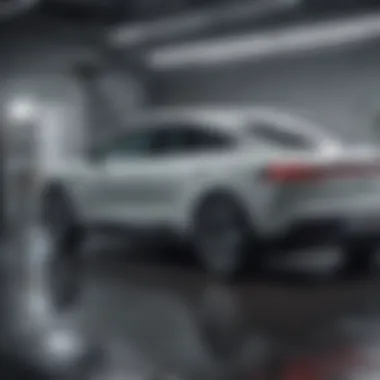
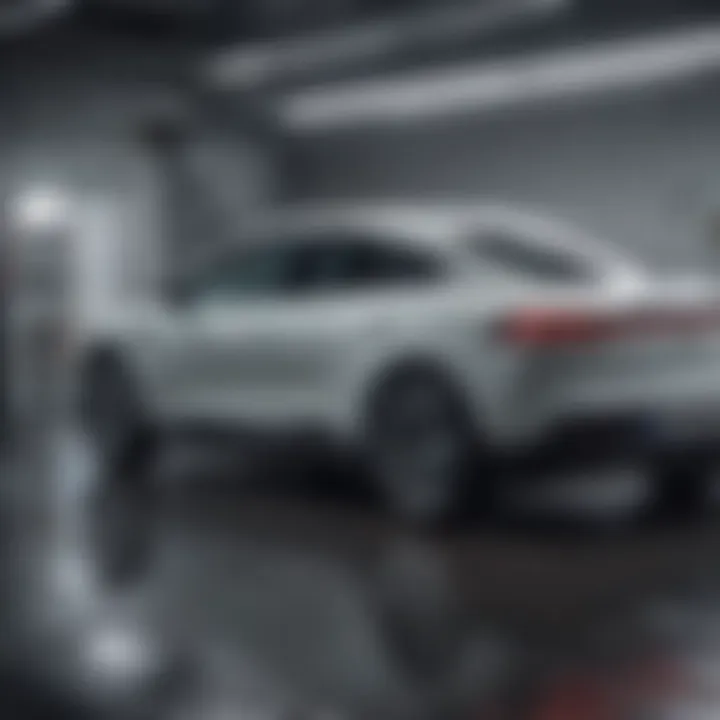
Innovation plays a critical role in mitigating GHG emissions associated with electric vehicles. Newly emerging battery technologies and improvements in energy efficiency are essential in reducing the carbon footprint throughout the vehicle’s life cycle. One of the most beneficial characteristics of these innovations is their ability to enhance battery lifespan, thereby reducing the need for frequent replacements, which inadvertently cuts down subsequent manufacturing emissions.
A defining unique feature of these advancements is the development of solid-state batteries which promise higher energy densities and lower chances of failure. The advantages are evident; however, challenges remain in scaling up production efficiently and affordably. This innovation ultimately contributes to our goal of minimizing the overall GHG emissions footprint and aligns with future policy directions pushing for cleaner transportation alternatives.
Resource Depletion and Sustainability
Impact on Rare Earth Elements
Shifting away from the emissions theme, the influence on rare earth elements in the context of electric vehicle production presents another concern. The extraction and processing of rare earth metals, crucial for many EV components, complicate the sustainability narrative. Importantly, these processes can lead to significant ecological damage, as mining these metals often involves extensive land disruption and waste generation.
The key characteristic of this issue is that the demand for rare earth materials is closely tied to rapid growth in electric vehicle manufacturing. As such, the sustainability of sourcing these minerals becomes paramount. There exists a fine balance between promoting electric mobility innovations while ensuring that such practices do not gravely harm the environment.
Sourcing Alternatives and Innovations
Addressing the sustainability issue requires exploring sourcing alternatives and innovations to reduce reliance on rare earth elements. Recent advancements in battery recycling technologies are a step toward mitigating the demand for new materials. This move is largely driven by a sector grappling with the ramifications of extracting and processing scarce and often harmful materials. A significant advantage here is that refined recycling processes not only recover valuable materials but also reduce the carbon footprint of new battery production.
However, a unique aspect of these innovations is their nascent stage; widespread implementation and adoption remain hurdles to overcome. By prioritizing sustainable sourcing, the industry can aim for a future where EVs contribute positively to environmental goals instead of becoming just another source of pollution and resource exploitation.
"The true measure of sustainability in electric vehicles lies in not just zero emissions during use, but in a holistic approach to their entire lifecycle."
In closing, understanding these environmental considerations provides insight into the seamless interplay between innovation and sustainability for electric vehicles. It encourages continued research and adaptation of practices aimed at aligning technological progress with ecological responsibility.
Economic Implications
The economic implications of electric vehicles (EVs) stretch far beyond mere purchase prices. A thorough understanding of these factors is vital for grasping the broader context of electric mobility adoption. Within this framework, cost-benefit analysis plays a crucial role, enabling prospective buyers to evaluate not just initial expenses but also the expected long-term savings. Moreover, government incentives can significantly alter the financial landscape surrounding EV purchases, making them more accessible to the everyday consumer. Simultaneously, market dynamics shape consumer behavior, influencing decisions that either spur or slow EV adoption.
Cost-Benefit Analysis of EVs
Initial Investment vs. Long-Term Savings
The initial capital needed to purchase an electric vehicle can be a sticking point for many potential buyers. Typically, EVs have higher upfront costs compared to traditional gasoline vehicles. However, the compelling aspect of this scenario lies in the long-term savings. Many consumers forget to consider the lower operating costs associated with EVs. For example, one of the standout characteristics of electric vehicles is their energy efficiency. Charging an EV often costs less than filling a gas tank, especially in regions where electricity from renewable sources is abundant.
This dynamic between initial investment and long-term savings illuminates an essential aspect of understanding the overall economic framework surrounding EVs. It renders the choice of investing in an EV more appealing as individuals calculate ongoing savings related to fuel and maintenance expenses, which tend to be lower for these vehicles.
On the downside, prospective buyers may feel overwhelmed by the higher upfront costs, leading them to delay their purchase. Still, highlighting the unique feature of EVs, their longevity, significantly compensates for the higher initial financial output, assuring an economically sound investment over time.
Government Incentives and Subsidies
Government incentives play an intriguing role in making electric vehicles a more appealing choice for buyers. Offering rebates and tax credits, these programs are designed to lower the financial barriers associated with the purchase of an EV, effectively addressing the initial investment hurdle. The enticing attribute of these incentives is their potential to enhance affordability. Some programs can even cover thousands of dollars off an EV's purchase price.
However, the distinct feature of government subsidies is their variability across regions and changes in political leadership, occasionally leading to confusion among buyers. End users may be unsure about what incentives they are eligible for, which can slow down the purchasing process. Therefore, it’s important to consider the updates in policies regularly to stay informed about the economic benefits that can significantly impact the overall costs associated with electric vehicle ownership.
Market Dynamics and Consumer Behavior
Trends in Electric Vehicle Adoption
The electric vehicle market is witnessing a noticeable shift, with trends indicating a steady increase in adoption. Factors such as rising fuel prices, consumer awareness about environmental issues, and the improved range of available models contribute to this budding enthusiasm. Collectively, these trends encapsulate the changing attitudes towards sustainable transportation.
The attractive characteristic of this trend is its self-reinforcing nature; as more people adopt EVs, manufacturers are encouraged to invest in technology, which in turn leads to better products. The unique feature here is the expanding variety of options available, from luxury vehicles to budget choices. However, this expanding landscape can also confuse consumers, making it difficult to navigate through the numerous available models.
Factors Influencing Consumer Decisions
Certain determinants have significant sway over consumer decisions regarding electric vehicle purchases. These factors range from personal financial situations to broader societal influences, such as the accessibility of EV charging infrastructure. A key aspect of consumer behavior is how the fear of range anxiety may deter potential buyers.
Additionally, Marketing plays a substantial role in shaping perceptions about electric vehicles. As companies invest in more robust marketing campaigns, public understanding and acceptance of EVs continue to grow.
A unique consideration is consumer education—easing misconceptions about electric vehicles and highlighting benefits can encourage more purchases. Therefore, factors influencing consumer decisions become a vital element in assessing the adoption rate of EVs, providing insight into ways stakeholders can foster a more supportive environment for sustainable transportation computing.
Technological Advances in EVs
Technological advancements play a pivotal role in the electric vehicle (EV) sector, shaping the future of transportation. Innovations in battery technology and charging infrastructure not only enhance performance but also support the overarching goal of sustainability. By exploring these developments, we gain a comprehensive insight into how they affect the life cycle analysis of EVs, detailing both their advantages and areas for improvement.
Battery Technology Innovations
Developments in Lithium-ion and Beyond
The battery technology landscape has evolved significantly, with lithium-ion batteries leading the charge. As the most widely adopted battery type, they offer a high energy density and decent cycle life, making them a go-to choice for many manufacturers. One key characteristic of lithium-ion batteries is their ability to recharge quickly compared to older technologies. This feature positions them as a beneficial option for developers seeking efficient energy solutions.
However, it’s worth noting that lithium-ion batteries are not without their downsides. They often rely on cobalt, which raises ethical concerns regarding mining practices. Additionally, the environmental impact of extracting and processing lithium can’t be brushed aside.
Another interesting development is the emerging research into solid-state batteries. These have the potential to enhance safety and efficiency, as they eliminate the flammable liquid electrolyte present in lithium-ion batteries. Yet, this technology is still in its infancy and faces scalability challenges that need addressing.
Impact on Life Cycle Analysis
The integration of innovative battery technologies significantly affects life cycle analysis outcomes. A prominent characteristic of the latest advancements is the emphasis on reducing environmental footprints. Next-gen batteries often prioritize materials that have less harmful extraction processes, which is crucial for improving overall sustainability.
This focus allows manufacturers to optimize performance across the vehicle's life cycle, from production through end-of-life recycling. However, transitioning to new battery technologies can require upfront investment and adjustments in production lines. The unique features of these advancements can lead to longer battery life and lower maintenance costs, although acquiring that technology may be an initial hurdle for some players in the market.
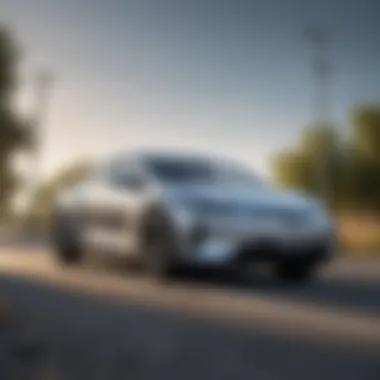
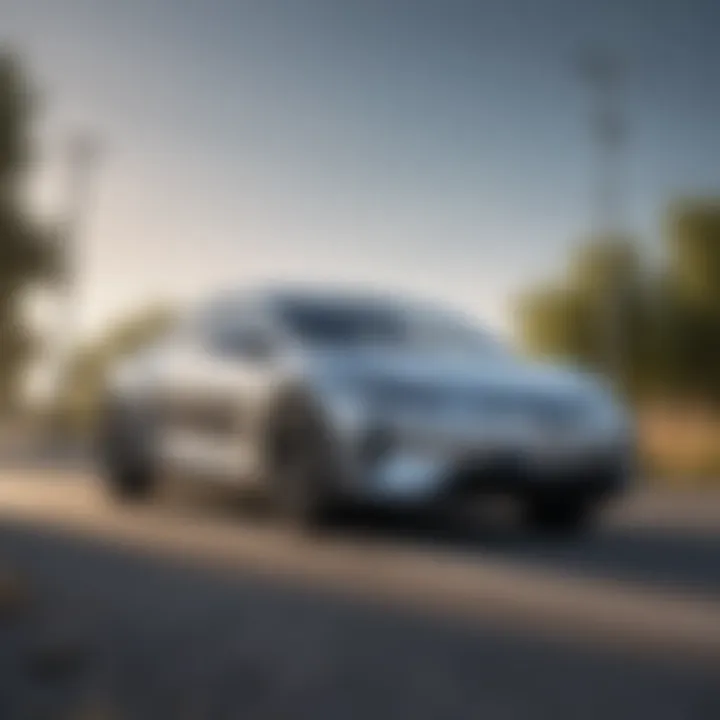
Charging Infrastructure Enhancements
Role of Fast Chargers
Fast chargers are altering the landscape of electric vehicle usability. Their primary role is to reduce the time required to charge an EV significantly, making long-distance travel more feasible. A key characteristic of fast charging stations is their ability to deliver high power levels, allowing vehicles to charge up to 80% in as little as 30 minutes.
This feature is particularly vital for users hesitant about EV adoption due to range anxiety. Nevertheless, widespread installation of fast charging stations hinges on significant investment in infrastructure, and there’s ongoing debate about their environmental impacts, particularly concerning energy sources.
Integration with Renewable Energy Sources
The integration of renewable energy sources into charging infrastructure represents a step toward decarbonization of the EV sector. This connection enhances the sustainability narrative, as it allows EVs to draw energy from wind and solar installations, minimizing emissions during operation.
A significant benefit of this alignment is its potential to lower overall life cycle emissions, if carefully implemented. However, a challenge remains in ensuring a consistent energy supply, as renewables can be intermittent. Addressing these issues is crucial for maximizing the sustainability benefits of electric vehicles.
In summary, technological advances in batteries and charging infrastructure not only reshape the operational landscape of electric vehicles but also serve as essential components of a comprehensive life cycle analysis. As these technologies develop, both their positive and negative effects warrant careful consideration to guide future improvements in electric mobility.
Comparative Analysis with Alternative Mobility Solutions
The exploration of alternative mobility solutions is crucial in understanding the wider implications of transitioning from traditional vehicles to electric vehicles (EVs). This analysis not only compares EVs with other technologies but also sheds light on their potential role in revolutionizing transportation. This section discusses the two main alternative solutions: Hydrogen Fuel Vehicles (HFVs) and public transportation.
Comparison with HFVs (Hydrogen Fuel Vehicles)
Hydrogen Fuel Vehicles offer an interesting alternative to electric vehicles, particularly when it comes to emissions reduction. They run on hydrogen gas, which can be produced from various sources, including natural gas and renewable energy. Unlike conventional fuel systems, these vehicles emit only water vapor, making them enticing for urban planners and policy-makers alike.
One key aspect is the infrastructure required for HFVs. The current supply chain is much less developed than the electric charging network, which poses a notable hurdle. While charging points for EVs are becoming ubiquitous, hydrogen fueling stations are still few and far between.
From an economic perspective, examining operational costs can yield valuable insights. Generally, HFVs have higher upfront costs compared to EVs, which might deter some consumers. However, as technology evolves, hydrogen production appears to be moving towards cost-competitive pricing. This balance of investment versus operational expense plays a critical role in consumer choice and market shift.
Public Transportation and Shared Mobility
Public transportation systems and shared mobility models, such as carpooling or ride-sharing, emerge as significant players in reducing urban traffic and emissions.
Impact on Urban Emissions
The impact on urban emissions is a vital discussion point. By shifting more people into a single transport option, cities can dramatically reduce the number of vehicles on the road, which directly correlates to lower emissions. Evidence suggests that cities with robust public transit networks experience a decrease in greenhouse gas emissions. Additionally, optimizing routes and utilizing electric buses can further cut down emissions.
The key characteristic of this approach is efficiency. When multiple individuals share a vehicle (or public transport), the overall carbon footprint per person decreases. The integration of clean technology into public transit offers a dual benefit: reducing emissions while maintaining or enhancing mobility.
"Cities, if they are to truly be sustainable, must prioritize public transportation. It's not just about moving people but about creating a healthier environment for all."
Future of Mobility in Smart Cities
The concept of Smart Cities embodies a holistic view of urban planning that integrates cutting-edge technology with transportation solutions. Here, the future of mobility will likely be defined by a combination of electric vehicles, HFVs, and enhanced public transport systems.
The uniqueness of smart mobility solutions lies in their adaptability and focus on user experience. Advanced apps can inform users about the best ways to travel, factoring in real-time data on traffic patterns and vehicle availability. Moreover, the implementation of autonomous vehicles is gradually becoming part of the bigger picture.
While these advancements can help reduce congestion and pollution, there are challenges. Data privacy and the need for infrastructure investment are major concerns. Furthermore, without careful planning, there's a risk that new technologies could perpetuate existing inequalities in access to transportation.
Future Directions in EV Life Cycle Analysis
Examining the future directions in Electric Vehicle (EV) Life Cycle Analysis (LCA) is not just a passing fancy; it is a necessity amid growing environmental concerns, advancements in technology, and shifts in consumer preferences. Understanding this facet helps stakeholders, from policymakers to manufacturers, shape strategies that are both sustainable and economically viable. Delving into emerging research areas and recommendations for policy is pivotal for enhancing the effectiveness of LCA in assessing EVs.
Emerging Research Areas
As the EV landscape continues to evolve, several research areas have gained traction. One prominent focus is on improving battery recycling techniques. While the current methods offer some recycling capabilities, there’s a pressing need for innovation to extract valuable materials without harming the environment. Advances in this area could dramatically reduce resource consumption, making the entire lifecycle of electric vehicles more sustainable.
Another area ripe for exploration is the impact of artificial intelligence (AI) and machine learning. These technologies can optimize supply chains and predictive maintenance, thereby increasing the longevity of EVs. Moreover, research into the sustainability of alternative materials can potentially lessen dependency on rare earth elements used in battery production.
Additionally, understanding user behavior can lend insight into usage patterns, helping refine energy management systems. By employing behavioral science to analyze driving habits, we can better tailor EV design and infrastructure towards practical, energy-efficient solutions.
Policy Implications and Recommendations
The future of EV LCA also hinges considerably on policy frameworks. Creating robust legislative avenues presents a chance to not only push EV adoption but also ensure that sustainability principles remain at the forefront of technological advancement.
Legislative Frameworks for Sustainability
Legislative frameworks are essential for establishing the groundwork necessary for sustainability in EV development and management. These frameworks can encourage manufacturers to embed sustainable practices within their operations. A key characteristic of these frameworks is their adaptability; they can evolve in response to technological advances and environmental findings. For instance, we could see policies that provide tax incentives for companies adopting circular economy practices in EV production.
The unique feature of these frameworks is their capacity to integrate various governance levels—from local regulations encouraging community charging stations to national policies promoting research and development in battery technology.
While such legislation can encourage innovation, potential downsides include bureaucratic inertia, which might slow down the very advancements they aim to champion. Therefore, careful implementation and regular revisions are critical to ensure alignment with technological trends and environmental needs.
Collaboration between Stakeholders
Collaboration between various stakeholders is a crucial aspect of advancing EV LCA. It includes manufacturers, government entities, non-profits, and consumers, each contributing a unique perspective or resource.
One facet that often emerges from stakeholder collaboration is the pooling of resources and knowledge. This cooperative effort can lead to ground-breaking approaches in both technology and policy. The key characteristic of such collaborations is their capacity to foster innovation; when varied interests come together, solutions are often more comprehensive and effective.
A unique feature of stakeholder collaboration is the ability to harness diverse expertise and perspectives, facilitating a holistic approach towards EV LCA. However, balancing the interests of different parties can be challenging. Each stakeholder may prioritize different goals—some focusing on profit, while others might insist on environmental conservancy. Navigating this landscape calls for strong communication and shared mission commitments to steer the collaboration effectively.
"The future of Electric Vehicle technology is not just about cars; it’s about building a sustainable ecosystem."
In summary, the future directions in EV Life Cycle Analysis are shaped by research innovations and policy recommendations. Addressing environmental concerns while balancing economic factors requires a multifaceted approach that transcends traditional boundaries. Emerging technologies and collaborative frameworks can pave the way for more sustainable practices in the electric vehicle industry.