Exploring Cell Structure and Functionality

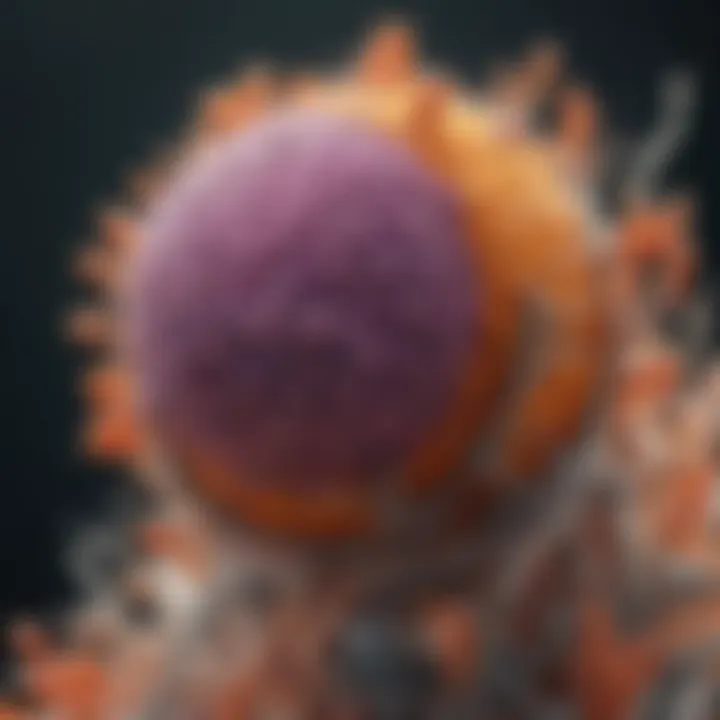
Intro
Cell structure serves as the fundamental basis for understanding biological function. The intricate relationship between various components within cells dictates how they operate and interact with their environment. Each cellular structure, from the plasma membrane to specialized organelles, plays a unique role in maintaining the overall functionality of the cell. By dissecting these relationships, one uncovers the secrets of life.
Research Overview
This section outlines the approaches used in current research regarding cell structure and its functionality. Understanding these elements is crucial for advancements in both scientific knowledge and practical applications in multiple fields.
Methodological Approaches
Research methods in this area include:
- Microscopy Techniques: Advanced imaging techniques such as electron microscopy and fluorescence microscopy allow researchers to observe cellular structures in high detail.
- Biochemical Methods: Techniques like Western blotting and flow cytometry help identify and analyze the proteins involved in cellular functions.
- Genetic Manipulation: Technologies such as CRISPR-Cas9 facilitate the study of gene function in relation to cellular structure.
These methods collaborate to form a comprehensive understanding of how the cellular architecture impacts its functionality.
Significance and Implications
Understanding cell structure is not just an academic exercise. The implications extend to medicine and biotechnology.
- Disease Treatment: Insights into cellular dysfunction can lead to the development of targeted therapies for diseases like cancer.
- Biotechnological Innovation: Knowledge of how cells work can result in advancements in tissue engineering and regenerative medicine.
"The architecture of the cell not only defines its function but also its interactions with other cells."
Current Trends in Science
Research into cell structure is dynamic, with ongoing innovations enhancing our understanding.
Innovative Techniques and Tools
New tools being developed include:
- Super-resolution Microscopy: This allows scientists to view cells at unprecedented resolution, revealing details previously hidden.
- Single-Cell Sequencing: It enables researchers to analyze the genomic data of individual cells, helping to link structure and function at a granular level.
Interdisciplinary Connections
Modern cellular biology intersects with various fields such as:
- Bioinformatics: Analyzing large datasets helps in understanding patterns in cellular behavior.
- Material Science: Insights from cell structure are applied to design biomaterials for medical applications.
This interdisciplinary approach enriches both scientific understanding and practical applications in biotechnology and medicine.
The exploration of cell structure and functionality plays a pivotal role in advancing our biological knowledge and addressing real-world challenges.
Intro to Cellular Biology
Cellular biology is a fundamental field that examines the basic structural and functional unit of life: the cell. The importance of this subject, particularly in the context of understanding how structure influences functionality, cannot be overstated. This article aims to dissect various aspects of cellular biology, offering a detailed exploration of how cellular components interact and contribute to the overall life processes.
The study of cells encompasses multiple levels, from the basic definition of cell structure to the complexity of cellular communication and metabolism. Understanding cellular biology is crucial for students, researchers, educators, and professionals as it lays the groundwork for advancements in medical science, biotechnology, and environmental sciences.
A clear grasp of how cellular structures operate and adapt provides insights into not only basic biology but also the application of these principles in fields such as genetics, microbiology, and biochemistry. Thus, readers will find that the connection between cell structure and functionality is a rich topic, vital for achieving a comprehensive understanding of life itself.
Definition of Cell Structure
Cell structure refers to the arrangement and organization of various components within the cell. This definition encompasses multiple elements, including the cell membrane, organelles, cytoskeleton, and genetic material. Each component plays a significant role in maintaining the integrity and functionality of the cell.
Specifically, the cell membrane acts as a selective barrier, regulating the entry and exit of substances. Organelles like the mitochondria, endoplasmic reticulum, and Golgi apparatus perform specialized functions crucial for the survival and replication of the cell. Furthermore, the cytoskeleton provides structural support and facilitates movement within the cell. Understanding these components is essential because the specific organization leads to the efficiency of cellular processes.
Importance of Cell Functionality
Cell functionality refers to the processes and activities that a cell undertakes to sustain life. It includes metabolism, energy production, cellular communication, and response to external stimuli. Each of these functions is inextricably linked to the cell's structure, where specific arrangements optimize the performance of these tasks.
The significance of cell functionality extends beyond basic survival. For instance, in medical biology, understanding how cells communicate can lead to breakthroughs in treatment methods for diseases.
Additionally, the study of cellular function aids scientists in developing targeted therapies in cancer treatment or understanding metabolic disorders. The interdependence of structure and functionality serves as a foundation for innovations in biotechnology and genetic engineering, making it a critical area for ongoing research.
Overview of Cell Types
Understanding the different types of cells is fundamental in cellular biology. Cells are the building blocks of life, and their diversity reflects the complexity of biological systems. In this section, we will explore the major categories of cells: prokaryotic and eukaryotic. Each type has distinct structural features and functionalities, which play a crucial role in the organism's overall physiology.
Prokaryotic Cells
Prokaryotic cells are characterized by their simplicity. They lack a defined nucleus, and their DNA floats freely in the cytoplasm. These cells are generally smaller compared to eukaryotic cells, ranging from 0.1 to 5.0 micrometers in diameter. One of their most notable features is the presence of a rigid cell wall, which provides structural support and protection.
The most common examples of prokaryotic cells are bacteria and archaea. Prokaryotes reproduce asexually through a process called binary fission, which allows for rapid population growth under favorable conditions. They are often found in diverse environments, from extreme conditions like hot springs to the human gut.
Key Features of Prokaryotic Cells:
- Lack nucleus and membrane-bound organelles
- Usually smaller in size
- Reproduce through binary fission
- Cell wall present
- Examples: Bacteria, Archaea
Eukaryotic Cells
Eukaryotic cells represent a more complex cellular organization. Unlike prokaryotic cells, eukaryotes have a defined nucleus that houses their genetic material. This allows for more sophisticated regulation of gene expression. Eukaryotic cells also contain various membrane-bound organelles, such as mitochondria and the endoplasmic reticulum, each performing specific functions vital for cellular operation.
Eukaryotes can be further divided into single-celled organisms, such as yeast and protozoa, and multicellular organisms, including plants and animals. Their larger size, typically ranging from 10 to 100 micrometers, allows them to develop specialized structures and functions.
Key Features of Eukaryotic Cells:
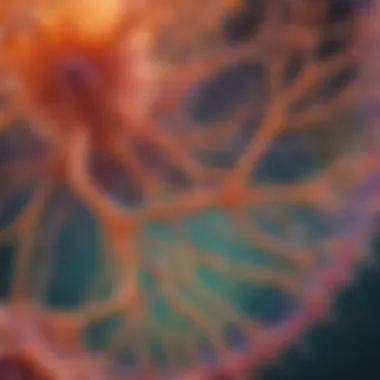
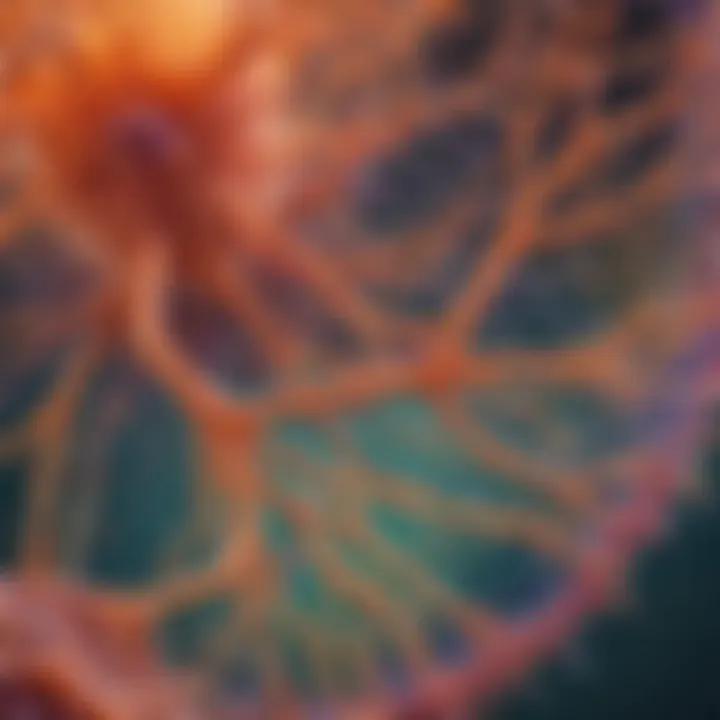
- Defined nucleus and membrane-bound organelles
- Larger in size compared to prokaryotes
- Can be unicellular or multicellular
- Examples: Plants, Animals, Fungi, Protozoa
Differences Between Cell Types
The distinction between prokaryotic and eukaryotic cells is profound and reflects their evolutionary paths. Below are significant differences:
- Nucleus: Prokaryotic cells do not have a nucleus; eukaryotic cells do.
- Size: Prokaryotic cells are generally smaller than eukaryotic cells.
- Organelles: Prokaryotic cells lack membrane-bound organelles, while eukaryotic cells have them.
- Reproduction: Prokaryotes reproduce asexually, while eukaryotes can reproduce both sexually and asexually.
Understanding these differences helps in various biological contexts, from microbiology to medicine, as they relate directly to how organisms interact with their environments and each other.
The diversity in cell types is not just a fundamental aspect of biology, but it also forms the basis for much of the functional diversity seen in life forms.
The examination of cell types allows researchers and students to appreciate the intricacies of life at a microscopic level. Consequently, this enhances not just academic inquiry but also provides a foundation for advancements in biotechnology, medicine, and environmental science.
Cell Membrane Structure
The cell membrane serves as a vital boundary, defining the boundaries of the cell. It is the first layer of defense against the external environment. This structure plays a crucial role in maintaining the overall integrity of the cell. It is important for various cellular functions, as it not only separates the cell from its surroundings but also facilitates communication between cells. A better understanding of this structure reveals how the cell interacts with its environment and how it responds to internal and external stimuli.
Composition of Cell Membranes
Cell membranes are primarily composed of phospholipids, which arrange themselves into a bilayer. This configuration is key for its performance. Embedded within this bilayer are proteins, cholesterol, and carbohydrates. Proteins serve various functions, from transport to signal reception. Cholesterol molecules help to stabilize the membrane's fluidity, which is critical for proper function. Carbohydrates attached to proteins and lipids contribute to cell recognition and communication.
- Phospholipids: Form the foundational structure of the membrane.
- Proteins: Facilitate various cellular processes and aid in communication.
- Cholesterol: Maintains the fluidity of the membrane.
- Carbohydrates: Play a role in recognition and signaling processes.
Understanding these components is essential. The interdependent relationship between these elements ensures that the membrane can perform its functions effectively.
Membrane Dynamics and Fluidity
The dynamic nature of the cell membrane is fundamental in its operation. Membrane fluidity is influenced by temperature, lipid composition, and the presence of cholesterol. At higher temperatures, membranes become more fluid, while lower temperatures can make them more rigid. This fluidity is essential for the movement of proteins and lipids within the membrane, enabling processes like endocytosis and cell signaling.
- Factors affecting fluidity:
- Temperature variations.
- Types of fatty acids in phospholipids.
- Cholesterol presence.
The adaptability of the membrane’s fluidity enables the cell to pivot effectively in response to environmental changes. Such flexibility is crucial for maintaining homeostasis, a key aspect of cellular functionality.
Role of Membranes in Cellular Communication
Membranes are pivotal in the realm of cellular communication. They contain receptors that recognize and bind to signaling molecules, triggering responses inside the cell. This interaction is known as signal transduction. Membrane fluidity allows receptors to move and cluster, optimizing their interactions with signaling molecules.
"Membrane proteins facilitate the binding of signaling molecules, leading to critical changes in cell function."
Moreover, the cell membrane's structure allows it to selectively permit substances to enter or exit. This selective permeability is crucial for maintaining the internal environment of the cell. Additionally, the recognition decals on carbohydrates aid in cell-to-cell communication and immune responses.
In summary, the composition, dynamics, and communication capabilities of cell membranes are vital topics that enrich our understanding of cellular functionality. Each element contributes not only to the structural integrity but also to the essential processes that sustain life.
Organelles and Their Functions
Understanding organelles is crucial for grasping cellular functionality. Organelles serve distinct roles within cells, with each contributing to the overall maintenance and operation of cellular processes. This section will elaborate on five fundamental organelles, detailing their individual functions and importance in cellular activity. Recognizing this functionality aids in comprehending how cells adapt and respond to both internal and external environments.
Nucleus: The Control Center
The nucleus is often described as the control center of the cell. It houses the cell's genetic material, DNA, which contains the instructions for protein synthesis essential for cellular function.
- Functions of the Nucleus:
- It regulates gene expression, influencing cell function and behavior.
- The nucleus is responsible for the replication of DNA during cell division.
The presence of a nuclear envelope helps protect the genetic material while allowing communication with the cytoplasm through nuclear pores. This communication is vital for maintaining the biology of the cell. The nucleus also contains nucleolus, where ribosomal RNA is synthesized, emphasizing its role in protein production.
Mitochondria: Energy Producers
Mitochondria are often referred to as the powerhouse of the cell. Their primary function is to generate adenosine triphosphate (ATP), the cell's energy currency.
- Importance of Mitochondria:
- They convert nutrients from food into usable energy through aerobic respiration.
- Mitochondria have their own DNA, which suggests an evolutionary symbiotic origin.
The energy produced is critical for numerous cellular processes, including metabolism, cell division, and maintenance of homeostasis. Understanding mitochondrial function also links to various medical conditions, highlighting their significance beyond basic biology.
Endoplasmic Reticulum: Synthesis and Transport
The endoplasmic reticulum (ER) is a network of membranes involved in protein and lipid synthesis. It is classified into two types: rough ER and smooth ER.
- Functions of the Rough ER:
- Functions of the Smooth ER:
- Studded with ribosomes, it synthesizes proteins destined for secretion or membrane insertion.
- Lacks ribosomes and is involved in lipid synthesis and detoxification processes.
The ER serves as a transportation system within the cell, moving synthesized proteins and lipids to the Golgi apparatus for further processing or distribution. This function is integral for maintaining cellular integrity and efficiency.
Golgi Apparatus: Modifying and Packaging
The Golgi apparatus is often likened to a post-office for the cell. Its primary function is to modify, sort, and package proteins and lipids for secretion or use within the cell.
- Key Functions of the Golgi Apparatus:
- It modifies proteins created in the rough ER by adding carbohydrate groups, producing glycoproteins.
- Packages these molecules into vesicles for transport to their destinations.
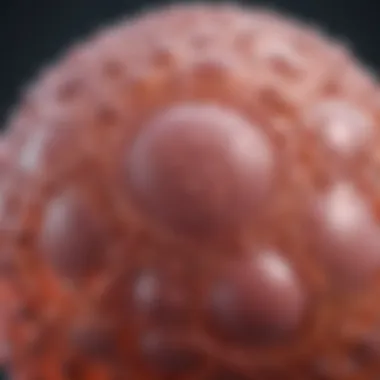
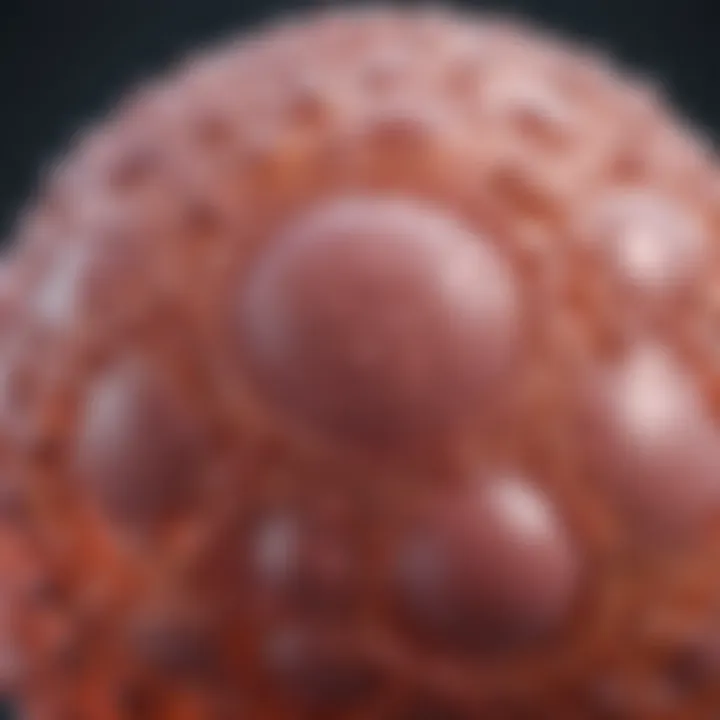
This organelle plays a pivotal role in cellular organization and the processing of molecules necessary for various functions, impacting overall cellular dynamics.
Lysosomes and Peroxisomes: Waste Management
Lysosomes and peroxisomes are organelles that manage cellular waste and maintain cellular health. Lysosomes contain enzymes for breaking down waste materials, while peroxisomes house enzymes for detoxifying harmful substances.
- Functions of Lysosomes:
- Functions of Peroxisomes:
- They digest macromolecules, cellular debris, and foreign pathogens.
- They metabolize fatty acids and detoxify hydrogen peroxide, a reactive metabolic byproduct.
Proper functioning of these organelles is essential for preventing the accumulation of toxic substances and ensuring cellular health.
"The organization of organelles within cells reflects their specialized functions, underscoring the complexity of cellular life."
Each organelle contributes to the cell’s ability to function and adapt within its environment. A thorough understanding of these components provides valuable insights into cellular biology, health, and disease.
Cytoskeleton: Structural Support and Motility
The cytoskeleton plays a vital role in maintaining the structural integrity and motility of cells. It is a complex network of protein filaments and tubules that provides shape to the cell, facilitates movement, and assists in cellular organization. Understanding the various components of the cytoskeleton and its functions offers insights into the essential processes that sustain cellular life and enable intercellular communication.
Components of the Cytoskeleton
The cytoskeleton is primarily composed of three types of protein structures:
- Microfilaments: These are the thinnest filaments of the cytoskeleton, made up of actin protein. They are primarily involved in muscle contraction, cell movement, and maintaining cell shape.
- Intermediate Filaments: These filaments are of intermediate size and provide mechanical support to cells. Different types of proteins form these filaments, including keratin, which is important in epithelial cells.
- Microtubules: These are the thickest components, composed of tubulin. Microtubules play crucial roles in cell division, intracellular transport, and forming the structure of cilia and flagella.
Together, these components orchestrate a dynamic structural framework that adapts to the cell's changing needs.
Role in Cell Shape and Movement
The cytoskeleton grants cells their shape and flexibility. It reacts to internal and external stimuli, allowing the cell to change its form when needed. For instance, amoeboid movement, utilized by cells like macrophages, depends heavily on the rearrangement of the cytoskeleton.
Moreover, the cytoskeleton is integral to the process of cell division, particularly during mitosis and meiosis. Microtubules form the mitotic spindle, which is essential for the equal distribution of chromosomes into daughter cells. Without a functioning cytoskeleton, these processes would falter, ultimately affecting cell survival and function.
"The cytoskeleton is not just a passive structure; it is a dynamic support system that orchestrates various cellular activities."
The interplay between the cytoskeleton and cellular components is essential for effective communication within the cell and with its environment. For example, the cytoskeleton influences the arrangement of organelles, facilitating efficient metabolic functions.
In summary, the cytoskeleton is more than structural support. It embodies the complexity of cellular motility and organization, allowing cells to adapt and thrive in diverse environments.
Cellular Metabolism
Cellular metabolism is fundamental to all living organisms. It encompasses the biochemical reactions that occur within cells, allowing them to maintain life and operate efficiently. By understanding cellular metabolism, we can comprehend how cells gather energy, synthesize vital biomolecules, and manage waste. This is critical for educational and scientific communities, offering insights that underpin advancements in medical fields and biotechnology.
Metabolism is generally divided into two categories: anabolism and catabolism. Anabolism involves building large molecules from smaller units, requiring energy. Conversely, catabolism breaks down complex molecules into simpler ones, releasing energy. The balance between these processes is essential for homeostasis and overall cellular health.
Overview of Metabolic Pathways
Metabolic pathways are sequences of enzymatic reactions that transform substrates into end products. These pathways can be linear, branched, or cyclic. Understanding these pathways allows scientists to pinpoint where issues may arise, resulting in metabolic disorders.
Key pathways include:
- Glycolysis: The breakdown of glucose for energy.
- Krebs Cycle: Produces energy through the oxidation of acetyl-CoA.
- Electron Transport Chain: Generates ATP utilizing electrons from reduced molecules.
Each of these pathways is tightly regulated by enzymes, ensuring that the cell's metabolic demands are met. Disruptions in these pathways can lead to significant cellular dysfunction.
Energy Conversion Processes
Energy conversion is a pivotal aspect of cellular metabolism. Cells convert nutrients into usable energy, primarily in the form of adenosine triphosphate (ATP). This energy currency powers numerous biological processes, including muscle contraction, nerve impulse transmission, and cellular growth.
There are several methods of energy conversion in cells:
- Aerobic Respiration: Involves oxygen and results in maximum ATP production from glucose.
- Anaerobic Respiration: Occurs without oxygen, generating less ATP and producing byproducts like lactic acid.
- Photosynthesis: Utilized by plants to convert light energy into chemical energy stored in glucose.
Each energy conversion process is vital, serving specific cellular requirements and conditions. Understanding these mechanisms highlights the adaptability of cells and their need for efficient metabolic pathways.
"The intricate processes of cellular metabolism are not merely academic; they are crucial for advancements in treatments and therapies."
By studying metabolic pathways and energy conversion, we not only gain a clearer picture of cellular functionality but also obtain tools for addressing metabolic diseases and improving biotechnological applications.
For more details, you can visit Wikipedia on Cellular Metabolism or check additional resources at Britannica for deeper insights into the subject.
Cell Communication and Signaling
In the realm of cellular biology, communication and signaling stand as pivotal processes that dictate how cells interact and respond to their environment. This topic is critical because it provides insight into how cells convey information, allowing them to coordinate their actions effectively. Cell signaling is fundamental for maintaining homeostasis, facilitating development, and orchestrating immune responses. Without efficient communication, the very framework of multicellular life would be disrupted.
Mechanisms of Cell Signaling
Cell signaling involves a complex network of pathways that translate external signals into cellular responses. These mechanisms can be broadly classified into several types:
- Autocrine signaling: In this type, a cell releases signaling molecules that bind to receptors on its own surface, influencing its own behavior.
- Paracrine signaling: Here, signals act on neighboring cells, facilitating localized communication between cells in the same area.
- Endocrine signaling: This form utilizes hormones released into the bloodstream, targeting distant cells throughout the body.
- Direct signaling: In some cases, cells communicate through direct contact, where membrane-bound signals interact with receptors on adjacent cells.
These mechanisms highlight the versatility of cell communication, ensuring that cells respond appropriately to various stimuli. Furthermore, signal transduction often involves a cascade of biochemical events, where the initial signal is amplified, leading to a significant cellular response. Through these processes, cells can modify their growth, metabolism, or activity based on internal and external cues.
Importance of Signal Transduction
Signal transduction plays a vital role in the overall functionality of a cell. The significance of this process can be seen in several areas:
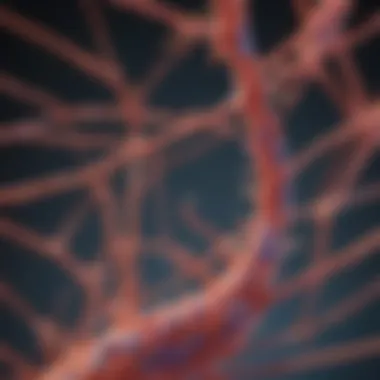
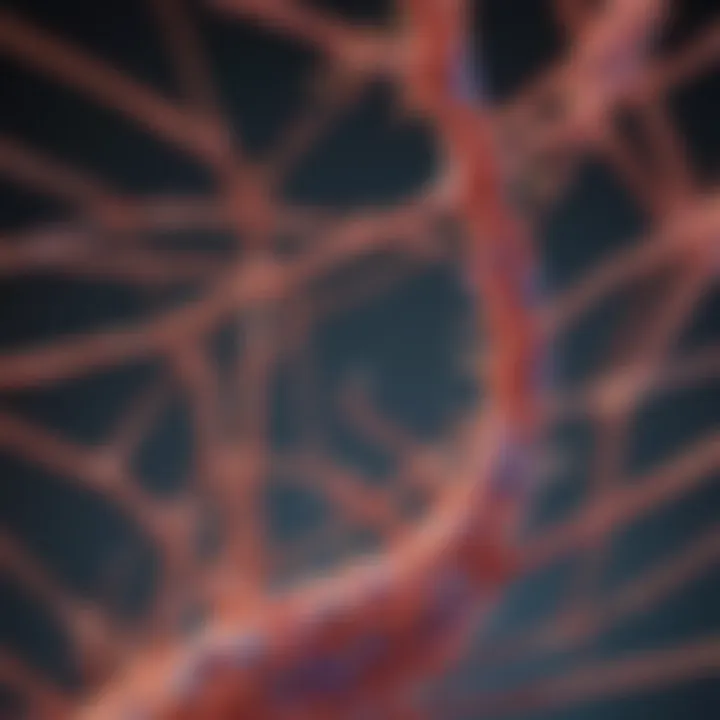
- Cellular Response: It converts signals into a coherent set of actions within the cell, such as gene expression, enzyme activity, or cell division. If signaling is flawed, it can lead to diseases such as cancer.
- Coordination of Functions: In multicellular organisms, efficient signal transduction ensures that different cell types can work together harmoniously. This coordination is crucial for processes like immune responses and tissue repair.
- Adaptation to Stimuli: Cells can adjust their behavior based on environmental signals. This adaptability is essential for survival, allowing cells to respond to stress or changes in nutrient availability.
The mechanisms of cell communication are not just processes; they represent the backbone of biological activity, enabling cells to react and adapt as necessary.
Understanding cell communication and signaling is fundamental for advancing fields like medicine and biotechnology. By dissecting these pathways, researchers can identify potential targets for new therapies, leading to innovative cures for various diseases.
Cell Cycle and Division
The cell cycle is fundamental to the biological functioning of all living organisms. It is the series of phases that a cell goes through as it grows and divides. Understanding the cell cycle provides insight into how cells replicate and maintain genetic integrity, which is crucial for the development, maintenance, and reproduction of both unicellular and multicellular organisms. Moreover, abnormalities in the cell cycle can lead to various diseases, including cancer. This section focuses on the intricate details of the cell cycle and the mechanisms of cell division, offering a clear perspective on why these processes matter in cellular biology.
Stages of the Cell Cycle
The cell cycle encompasses several distinct stages, each characterized by specific activities and processes. The major stages include:
- Interphase: This is the longest phase, making up the majority of the cell cycle. It is subdivided into three phases:
- M Phase (Mitosis): This phase includes several stages where the cell divides its copied DNA and cytoplasm to form two new daughter cells. The phases of mitosis include:
- G1 Phase (Gap 1): The cell grows and performs its normal functions. It checks for DNA damage before proceeding to DNA synthesis.
- S Phase (Synthesis): DNA replication occurs here. Each chromosome is duplicated to form sister chromatids, preparing the cell for division.
- G2 Phase (Gap 2): The cell continues to grow and produce proteins necessary for mitosis. The cell checks for DNA errors and prepares for mitotic division.
- Prophase: Chromatin condenses into visible chromosomes, and the mitotic spindle begins to form.
- Metaphase: Chromosomes align at the equatorial plane of the cell.
- Anaphase: Sister chromatids are pulled apart to opposite poles of the cell.
- Telophase: Nuclear membranes re-form around each set of chromosomes, which de-condense back to chromatin.
Mechanisms of Cell Division
Cell division is vital for growth, healing, and reproduction. There are two main types of cell division: mitosis and meiosis, each serving different functions in the organism.
- Mitosis: This type of division produces two genetically identical daughter cells. It is essential for somatic cells and facilitates growth and repair. The mechanism is tightly regulated by various proteins ensuring that errors do not occur during DNA replication and segregation.
- Meiosis: In contrast to mitosis, meiosis occurs in germ cells and results in four genetically diverse gametes. This process includes two rounds of division that halve the chromosome number, leading to genetic variation, which is vital for evolution and adaptation.
The regulation of these cell division processes is complex and includes checkpoints that monitor the integrity of DNA and the appropriate completion of preceding stages. Failure in these regulatory mechanisms can lead to uncontrolled cell proliferation, which is a hallmark of cancerous cells.
"The understanding of cell division not only elucidates fundamental biological processes but also enhances our ability to devise medical treatments for diseases rooted in cellular dysfunction."
Cellular Adaptation and Response
Cellular adaptation is a fundamental concept in biology that describes how cells adjust to changes in their environment. Understanding this topic is crucial as it reveals how cells maintain homeostasis and functionality under varying conditions. The adaptability of cells impacts their survival and performance, which in turn influences the overall health of an organism. Changes in nutrient availability, temperature, and stress can all trigger cellular responses that are vital for organismal efficiency.
Adaptations to Environmental Changes
Cells are continuously exposed to a range of environmental factors. Their ability to adapt is essential for survival. These adaptations can be physiological or structural. For instance, in low-oxygen conditions, cells may undergo hypoxia-inducible factor (HIF) activation, promoting pathways that enhance oxygen utilization. This is observed in muscle cells during intense exercise when they face temporary oxygen deprivation.
Another adaptation is observed in plant cells, which may develop thicker cuticles in response to high temperatures. These structural changes help in reducing water loss, safeguarding cellular functions.
The mechanisms behind these adaptations often involve:
- Gene expression changes: Specific genes are turned on or off in response to stimuli.
- Protein modifications: Enzymatic activities can alter based on environmental conditions.
- Morphological changes: Cells may alter shape or size to better suit their environment.
Ultimately, these adaptations play a crucial role in development, resilience, and long-term survival within diverse ecosystems.
Role of Stress Responses
Stress is a common challenge for cells, triggering a variety of responses that help maintain cellular integrity. Stress responses are crucial for mitigating damage and ensuring continuity of function. Cells utilize various pathways to respond to stress, which include oxidative stress, thermal stress, and mechanical stress.
One of the key mechanisms of stress response involves the activation of stress-activated protein kinases (SAPKs), which mediate cellular signaling pathways vital for coping with adverse conditions. For example, when cells experience oxidative stress, they may activate antioxidant defenses to neutralize reactive oxygen species.
The role of chaperone proteins is also significant during stress response, as they assist in proper protein folding and prevent aggregation. Notably, heat shock proteins exemplify this mechanism, promoting survival during thermal stress.
In summary, the ability of cells to adapt to their environment and manage stress is a critical component of their functionality. Understanding these processes enhances our knowledge of cellular biology and can inform biomedical applications in combating diseases and improving health outcomes.
"Cellular adaptability is not just a survival tactic; it shapes the evolutionary trajectory of organisms by influencing how they can thrive in diverse conditions."
In the context of medicine, recognizing these adaptations has direct implications for treatment strategies, particularly in disease states where cellular responses may be compromised.
Applications of Cell Biology
The fusion of cell structure and function is essential in various biological and practical applications. The understanding of cellular mechanisms offers insights into how cells operate, adapt, and respond under different conditions. This, in turn, paves the way for innovative solutions in medicine and biotechnology. The applications of cell biology are vast, proving critical for future advancements in health and technology.
Cell-Based Therapies
Cell-based therapies have emerged as a pivotal aspect of modern medicine, targeting a range of diseases and conditions. These therapies utilize human cells to restore or improve function in damaged tissues. Such treatments are distinguished by their ability to harness the body’s own repair mechanisms. Here are some key points regarding cell-based therapies:
- Regenerative Medicine: By transplanting specialized cells, it is possible to regenerate tissues, such as in spinal cord injuries or heart disease.
- Stem Cell Research: Stem cells possess the unique ability to differentiate into any type of cell. This feature is central in treating degenerative diseases and injuries.
- Immune Modulation: Using cells to enhance or suppress immune responses can help treat cancers or autoimmune disorders effectively.
- Personalized Medicine: Tailoring treatments according to the patient’s own cells increases the likelihood of treatment success.
Ultimately, the advancements in cell-based therapies highlight the importance of understanding cellular structure and functionality, as well as their interactions within the human body.
Biotechnology and Genetic Engineering
Biotechnology stands at the intersection of biology and technology, and genetic engineering is a significant component within this field. By manipulating the genetic material of organisms, researchers can create products and treatments that were not originally possible. Here are essential aspects to consider:
- Genetic Modification: The introduction of new genes into an organism can lead to desired traits, such as pest resistance in crops.
- Gene Therapy: This involves correcting defective genes responsible for disease development. It holds great promise for genetic disorders, providing potential cures where none existed before.
- Synthetic Biology: Creating new biological parts or systems can enhance technologies in medicine and agriculture. It is a forward-looking approach that combines biology and engineering principles.
- Environmental Applications: Genetically modified organisms can be developed to clean up pollutants or improve agricultural sustainability.
The exploration of biotechnology and genetic engineering emphasizes the critical relationship between structure and function at the cellular level. This knowledge not only enriches scientific understanding but also enhances practical applications that benefit society as a whole.
"Understanding the intricacies of cell biology enables remarkable advancements in medicine and technology."
In summary, the study of cell structure and functionality directly influences applications in various fields, notably in medicine and biotechnology. Cell-based therapies and genetic engineering exemplify this connection, showcasing how in-depth knowledge of cell biology can be translated into effective solutions. Through this exploration, we appreciate the profound impact that cellular understanding has on addressing complex biological challenges.
Closure
The topic of cell structure and functionality is paramount in understanding the essence of biological processes. This article has highlighted how cellular architecture underpins all aspects of life. The intricate relationships between different components such as cell membranes, organelles, and the cytoskeleton directly correlate to their respective functions.
In exploring these relationships, it is clear that the design of a cell is not merely a random arrangement of parts. Instead, each component is specifically tailored to facilitate essential functions that sustain life. For example, the membranes regulate what enters and exits the cell, thus maintaining homeostasis. The mitochondria produce ATP, the energy currency necessary for various metabolic processes.
Furthermore, advancements in cell biology have significant implications in fields such as medicine and biotechnology. Cell-based therapies, for instance, leverage the understanding of cellular components to treat diseases. By manipulating cellular structures, researchers can devise targeted treatments that respond to individual patient needs. This highlights not only the theoretical understanding gained from cellular studies, but also practical benefits that can emerge from this knowledge.
"Understanding cell organization is crucial for innovations in health and technology."
In summary, the exploration of cell structure and functionality presents not just a theoretical framework but also practical insights applicable in real-world scenarios. The adaptations and interrelations observed at the cellular level are foundational to advances in science and medicine. As our comprehension of these complexities grows, so too does our potential to improve health outcomes and innovate in technology.