Exploring Electric Car Battery Composition and Innovation
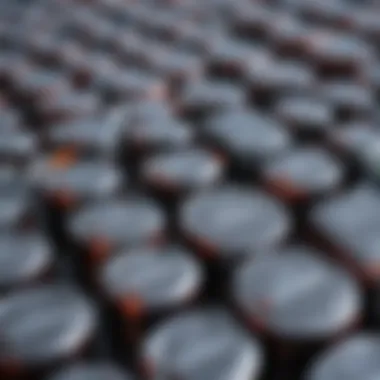
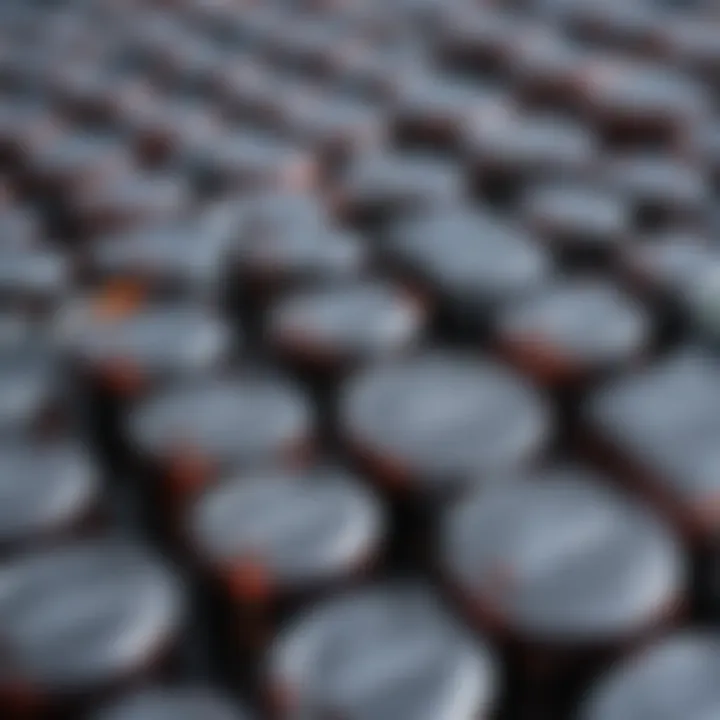
Intro
Electric car batteries form the heart of electric vehichles, powering everything from the mundane errands to cross-country odysseys. Yet, few fully grasp the complex makeup of these batteries or how their components work in harmony to deliver both efficiency and performance. This article aims to demystify the key aspects of electric car battery composition, tracing the materials, technologies, and innovations that drive the industry forward.
Understanding these elements is not just an academic exercise; it carries significant implications for the future of sustainable mobility. As electric vehicles (EVs) gain traction globally, the need for a deeper comprehension of their power sources becomes apparent. The trajectory of energy storage technology is evolving rapidly, and a grounding in these topics can illuminate how we approach energy consumption, sustainability, and technological advancement in our daily lives.
Central to this discourse is the acknowledgment of the balance required in the design of these batteriesâtuning for performance, safety, and environmental considerations often presents a complex challenge.
"Batteries are the fuel tanks of electric cars, but they are significantly more complex and fascinating than just that. Understanding their anatomy and operation is essential in todayâs EV-centric world."
Next, letâs dive into the Research Overview segment, where foundational methodologies and the significance of the subject will be explored.
Prelude to Electric Car Batteries
Electric car batteries are like the beating heart of an electric vehicle, vital for its operation and performance. Understanding their composition is crucial, not just for those in the automotive industry but also for anyone interested in the future of sustainable transportation. With the growing momentum towards green energy, knowing how these batteries function and what they are made of can provide insights into their efficiency, longevity, and environmental impact.
As electric cars become more prominent, so does the importance of comprehending the technology that powers them. Itâs not merely a matter of convenience; the makeup of the battery can influence factors such as range, charging time, and safety. Letâs dive into why these components carry significant weight in the realm of modern vehicles.
Significance in Modern Transportation
Electric car batteries represent a revolutionary shift in how we perceive travel. They reduce our reliance on fossil fuels, contributing to lower greenhouse gas emissions. This shift is not just a fad; it's a fundamental change in the paradigm of personal and commercial transportation. In a time where climate change looms large, electric vehicles are seen as a beacon of hope.
Moreover, as technology advances, the performance of electric vehicles improves. Consider how batteries develop over time: each iteration comes with better energy density and charging capacity. An understanding of battery composition can help consumers appreciate these enhancements.
"The future of transportation rests on the intersection of innovation and sustainability, and electric car batteries are at the forefront of this evolution."
Overview of Electric Vehicle Types
To grasp the composition of electric car batteries, it's essential to recognize the different types of electric vehicles and how each type aligns with battery technologies. Hereâs a brief overview:
- Battery Electric Vehicles (BEVs): These cars rely solely on batteries for power. They do not have any internal combustion engine (ICE) and include popular models like the Tesla Model 3 and the Nissan Leaf. The focus is often on maximizing battery capacity and efficiency.
- Plug-in Hybrid Electric Vehicles (PHEVs): These vehicles combine a traditional ICE with a battery. They can run on electricity alone for shorter journeys or switch to gasoline for longer trips. The batteryâs role here is to support the electric driving range without fully replacing the gasoline engine.
- Hybrid Electric Vehicles (HEVs): Unlike PHEVs, HEVs canât be plugged in to charge. They utilize a smaller battery that is recharged through regenerative braking and the gasoline engine. Their battery composition is less critical as they operate using both electric and fuel power.
Each of these vehicle types interacts differently with their respective batteries. By understanding these nuances, we can better appreciate the complexities of battery design choices and their implications for the future of electric mobility.
Key Components of Electric Car Batteries
Electric car batteries play a pivotal role in the functionality and performance of electric vehicles. Understanding their key components not only illuminates the intricate relationships within the battery's construction but also offers insights into how advancements in technology can enhance overall vehicle efficiency and safety. Each component, from the anode and cathode materials to the separator and electrolyte composition, plays a crucial role in the battery's performance. As trends in sustainability and demand for electric vehicles grow, the examination of these elements becomes ever more significant.
Anode Materials
Graphite Usage
Graphite has been the mainstay in the anode materials for lithium-ion batteries, predominantly due to its ideal characteristics. It possesses a layered structure that facilitates lithium-ion intercalation, a critical process that enables the battery to charge and discharge effectively. Its influence on energy density cannot be overstated; it allows for a compact battery design without compromising performance. Additionally, graphite is extensively available and relatively inexpensive, making it a widely adopted choice for manufacturers.
This aspect of graphite usage comes with its set of unique features. For instance, despite its benefits, graphite anodes do suffer from issues related to structural integrity over numerous charge cycles. This leads to capacity fade, which can hinder the overall lifespan of the battery. Thus, while graphite remains beneficial for many applications, ongoing research into alternatives continues to gain traction.
Emerging Alternatives
Emerging alternatives to conventional graphite materials are gaining attention in the electric vehicle sphere. Silicon, for example, has been touted for its exceptional capacity for lithium storage, theoretically doubling the energy density compared to traditional graphite. The high abundance of silicon also contributes to its attractiveness. However, the significant volumetric expansion that silicon undergoes during lithiation poses a serious challenge.
The characteristics of these alternatives make them noteworthy. Although they present enticing advantages, such as increased energy density, their stability and long-term performance are still under scrutiny. This paints a complex picture; while the future of battery technology could benefit from these materials, overcoming the inherent challenges is paramount for widespread adoption.
Cathode Materials
Lithium Cobalt Oxide
Lithium cobalt oxide has been a popular choice among cathode materials, particularly in consumer electronics and electric vehicles. Its high energy density means that it can store a significant amount of energy in a compact form, which is essential for enhancing the driving range of electric vehicles. Moreover, it operates well under a variety of conditions, maintaining stability and performance.
However, the drawbacks cannot be ignored. Lithium cobalt oxide is also more expensive compared to some alternatives, and its thermal stability comes into question. A notable critique of its application is the ethical implications of cobalt mining, often associated with poor labor practices in certain regions. As the market evolves, these considerations are driving a shift toward alternative cathode materials.
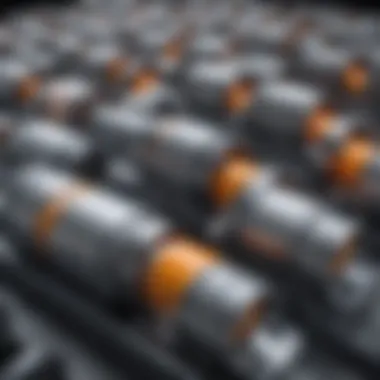
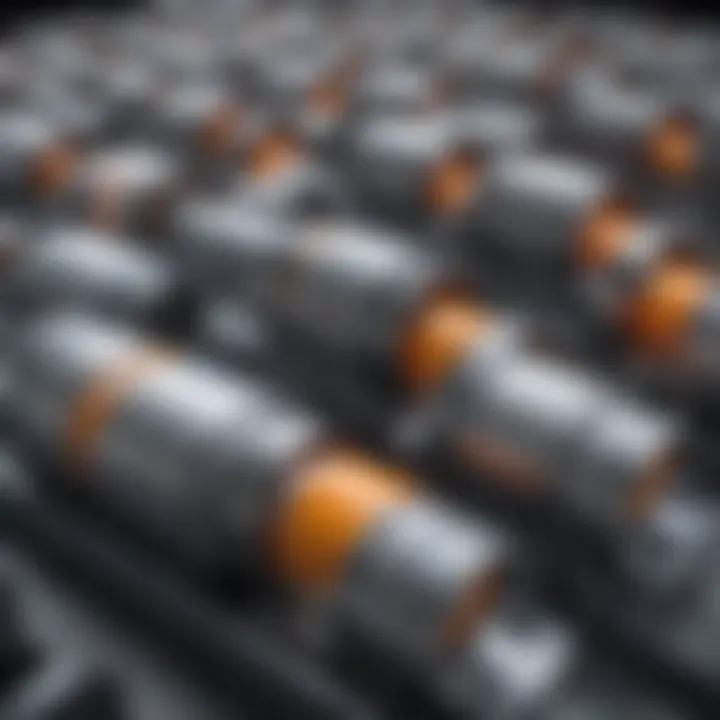
Lithium Iron Phosphate
Lithium iron phosphate offers a contrasting profile to lithium cobalt oxide. With a focus on safety, this material provides remarkable thermal stability and a long life cycle, making it an appealing choice for electric vehicle manufacturers looking for reliable performance.
One major benefit is its resilience against overheating, which enhances safety aspects during operation. However, the trade-off is a lower energy density. This means vehicles utilizing lithium iron phosphate may not achieve the same driving range as those using more energy-dense materials. Thus, while lithium iron phosphate may not be the most compact or energy-efficient option, its emphasis on safety and longevity holds significant merit within the growing electric vehicle market.
Electrolyte Composition
Liquid vs. Solid Electrolytes
The debate between liquid and solid electrolytes is an ongoing topic in battery technology. Liquid electrolytes, commonly found in traditional lithium-ion batteries, allow for fast ion movement and are well understood in terms of their performance. This feature makes them a practical choice in most existing electric vehicle applications.
On the other hand, solid electrolytes are gaining traction in the quest for improved safety and energy density. They eliminate some of the flammable risks associated with liquid electrolytes, potentially leading to safer battery designs. However, the challenge is in achieving adequate ionic conductivity without sacrificing performance. The interplay of these electrolyte types presents both benefits and drawbacks, each playing a significant role in future battery development.
Conductivity and Stability
The conductivity and stability of electrolytes deeply influence the overall efficiency of electric car batteries. Effective ionic conductivity is essential for maintaining performance during high demand, as it directly affects the charge and discharge rates.
Additionally, stability under various operating conditions ensures a reliable lifespan for the battery. Traditional liquid electrolytes often face challenges related to degradation over time, while solid electrolytes, though safer, require more research for scalability and integration into current battery technologies. This balance of conductivity and stability is crucial in achieving reliable and efficient electric car batteries.
Separator Functionality
Material Selection
The separator's material selection is another critical aspect of electric car batteries. Typically made from porous materials, the separator plays a vital role in preventing electrical short-circuits while allowing lithium ions to move freely between anode and cathode. The choice of materials can directly impact the battery's efficiency and safety.
With advancements in materials technology, the development of separators using innovative polymers is pushing the envelope in terms of performance. Yet, if those materials are not sufficiently robust or if they degrade, it could lead to safety hazards. Therefore, careful consideration is given to the properties of separator materials within battery design.
Role in Safety
The separator's role in safety cannot be overlooked. A well-designed separator ensures that the anode and cathode do not come in direct contact, which can be a recipe for disaster. Instances of thermal runaway highlight the importance of a reliable separator. By providing a barrier, it serves as a crucial line of defense against short-circuiting and overheating.
The continuous evolution of battery technology will undoubtedly shape the future landscape of electric mobility. Understanding the composition of these batteries isn't merely academic; it is vital for progressing toward a sustainable transportation future.
Battery Types Utilized in Electric Vehicles
Battery types play a crucial role in the performance, range, and overall efficacy of electric vehicles (EVs). Choosing the right battery not only affects how well a vehicle drives but also impacts the longevity and sustainability of its energy source. As the electric vehicle market continues to expand, understanding these types can help consumers, engineers, and researchers make informed decisions.
Lithium-Ion Batteries
Advantages and Disadvantages
Lithium-ion batteries, or simply Li-ion batteries, stand out for various reasons. Their high energy density is one of the main aspects that makes them attractive. They can store significant amounts of energy without taking up much space, which is vital for the compact design of electric vehicles.
However, itâs not all roses with Li-ion tech. They can be costly and have a limited lifespan, often requiring replacement after a certain number of charging cycles. This can become quite burdensome, especially for budget-conscious consumers. Despite these downsides, their ability to deliver robust performance makes them the go-to choice for most EV manufacturers today.
Applications in EVs
Li-ion batteries find homes in almost all modern electric vehicles, powering everything from compact cars to larger SUV models. Their versatile application is due in part to their substantial energy capacity and reliability. Car makers have become accustomed to this technology, which emphasizes its importance and deep integration in the industry.
That said, there are drawbacks associated with their use. The manufacturing process of lithium has its own environmental implications, raising questions about sustainability. When placed alongside the rising competition from other battery types, itâs clear that while they dominate the current landscape, advanced alternatives are always on the horizon.
Solid-State Batteries
Potential Benefits
Solid-state batteries are presenting an optimistic prospect. They replace the liquid electrolyte commonly found in traditional batteries with a solid electrolyte, leading to increased safety and energy density. This makes them lightweight and potentially extends the range of EVs significantly.
One interesting feature of solid-state batteries is their robust thermal stability. This reduces the risk of overheating, a critical factor in ensuring vehicle safety. Many see this as a game-changer, reinvigorating interest in battery technology and making manufacturers eager to invest.
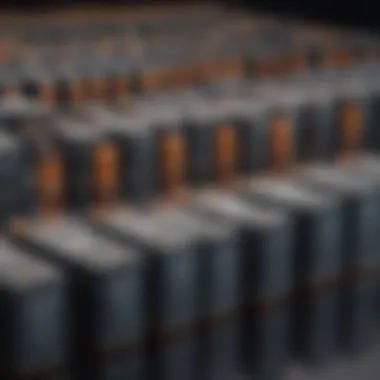
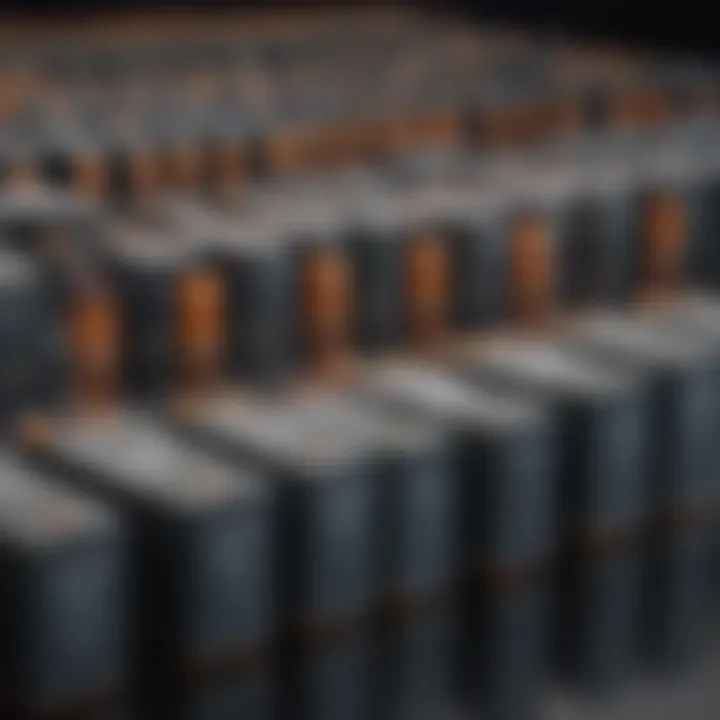
Current Development Challenges
While solid-state batteries hold promise, theyâre not without their hurdles. The production cost remains high, keeping their widespread adoption at bay. Scaling up production facilities involves significant investment and technological innovation.
This unique challenge reflects broader issues within battery technologyâstriking a balance between advancements and practicality remains tough. As companies tackle these obstacles, the spotlight shines on the benefits that could follow if these issues are resolved.
Nickel-Metal Hydride Batteries
Historical Context
Nickel-metal hydride batteries, or NiMH batteries, were once the standard for electric and hybrid vehicles. Their relatively simple chemistry and robust design allowed them to gain traction, especially in early EV models. While they donât boast the same energy density as lithium-ion batteries, they overcame challenges in durability and safety.
Interestingly, these batteries are often credited with making electric vehicles more mainstream, paving the way for todayâs innovations. Their history has left a significant mark on the evolution of EV technology and how we perceive battery options today.
Current Relevance
Though primarily supplanted by lithium-ion technology, NiMH batteries still have a role in the automotive market, particularly in hybrid vehicles. They are less expensive and provide reasonable performance for certain applications.
This relevance highlights their importance as transitional technology. As car manufacturers work on refining battery tech, NiMH remains a practical choice for specific segments, especially in markets where cost efficiency is vital. Their resilience in the face of newer technology underscores their contribution to sustaining the growing electric vehicle market.
Manufacturing Processes
Understanding the manufacturing processes of electric car batteries is crucial for several reasons. First, these processes directly influence the performance, lifespan, and safety of the batteries that power electric vehicles. A well-constructed battery not only enhances the driving experience but also contributes to the overall sustainability goals of the automotive industry. Manufacturers face numerous challenges here, including cost efficiency, technology integration, and minimizing environmental impact while meeting consumer demand.
Cell Production Techniques
Assembly Line Overview
When it comes to producing electric car batteries, the assembly line approach is quite the game changer. This method paves the way for streamlined production. In essence, the assembly line breaks down the battery-making process into simple, repetitive tasks that can be performed by workers or machines. One significant characteristic of this model is its scalability. As demand for electric vehicles rises, manufacturers can quickly ramp up production without missing a beat. Moreover, the structured workflow minimizes errors and increases overall quality, which is paramount for battery safety.
However, one must consider the downside too: relying heavily on assembly lines often leads to less flexibility in adapting to new battery technologies or designs. Additionally, if any hiccup occurs in one part of the line, it can cause a domino effect that stalls production entirely.
Quality Control Measures
Quality control measures play an integral role in ensuring that each battery cell produced meets rigidity and performance standards. The unique aspect of quality control is its ability to encompass multiple testing stages throughout the production process. By integrating tests at various pointsâsuch as pre-production inspections and post-assembly evaluationsâmanufacturers can catch defects early. This proactive approach is not just a good practice but a necessity in an industry where battery failures can lead to grave consequences.
One notable feature of quality control is the implementation of advanced analytics. Many manufacturers use data-driven methodologies to monitors performance metrics and predict potential failures. This helps ensure a reliable product reaches consumers. However, such systems require significant investments in technology and training, which can be a concern for smaller production facilities.
Impact of Recycling
The impact of recycling on electric car battery manufacturing cannot be overstated. With the increasing concerns about resource depletion and environmental sustainability, recycling has become a focal point for many manufacturers. By reclaiming valuable materials such as lithium, cobalt, and nickel, the industry can reduce reliance on mined resources and mitigate toxic waste problems.
Processes and Methods
Recycling processes for electric car batteries generally involve several critical stages. The most common methods include pyrometallurgical and hydrometallurgical recycling. In pyrometallurgy, discarded batteries are subjected to high temperatures, allowing for the recovery of metal alloys. This process is advantageous because it can handle various battery types. However, the downside is that it can consume large amounts of energy.
On the other hand, hydrometallurgy uses aqueous solutions to leach out metals while being less energy-intensive. It also has lower emissions which makes it a sensible option in today's environmentally conscious market. Yet, efficiency and completion rates can be a concern with these methods, sometimes resulting in valuable materials being left behind.
Environmental Implications
The implications of recycling electric car batteries extend beyond the immediate recovery of materials. Recycling practices significantly impact the environment by helping to minimize landfill waste and reducing the carbon footprint of battery production. The recycling process also ensures that harmful chemicals are disposed of responsibly, preventing them from leaching into the environment.
A striking feature of enhanced recycling programs is their contribution to a more circular economy. By closing the loop on battery materials, manufacturers can reduce their environmental degradation and resource depletion particularly relevant in light of rapid growth in electric vehicle adoption. Nonetheless, the challenge remains in scaling up these practices to meet the burgeoning demands of the electric vehicle market, all while keeping costs manageable.
"Recycling electric car batteries can pave the way for a sustainable future by turning waste into valuable resources."
In sum, manufacturing processes encompass a plethora of factors that are pivotal for electric car batteries, from cell production techniques to recycling methods. These elements interplay to determine the efficacy, safety, and longevity of the electric vehicles we drive today.
Future Trends in Battery Technology
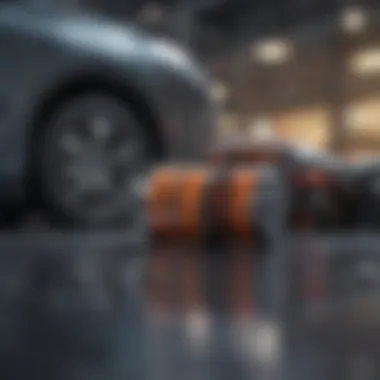
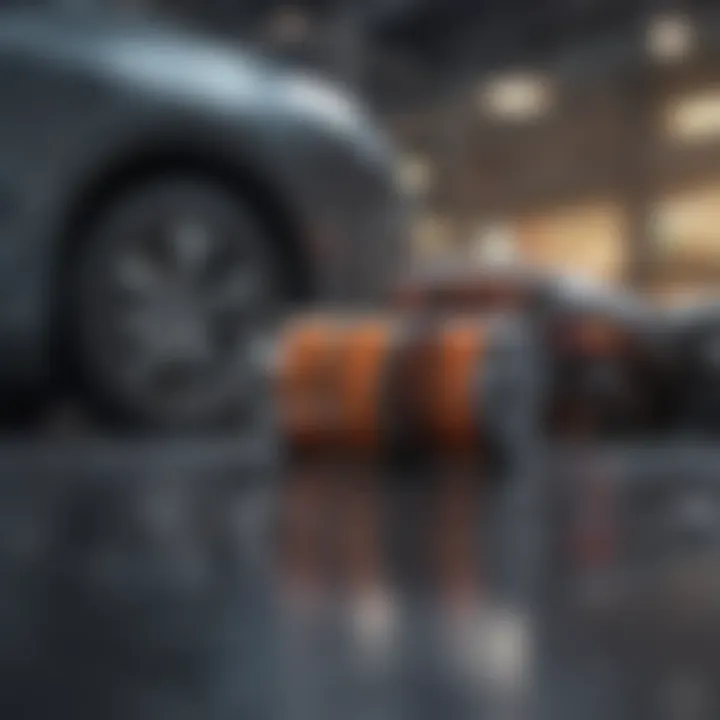
The exploration of future trends in battery technology is imperative for understanding the next wave of innovation in electric vehicles. As the world moves toward a greener and more sustainable future, advancements in battery technology stand at the crossroads of necessity and innovation. The quest for batteries that hold more energy, charge faster, and last longer has become a focal point in developing electric vehicles. Not to mention, these strides have implications not just for performance but also for the overall environmental impact of electric car usage.
Advancements in Energy Density
Research Innovations
Research innovations in energy density are all about pushing the limits of what batteries can achieve. In simple terms, energy density measures how much energy a battery can store for a given weight or volume. When you think of the ideal electric car battery, keeping weight low while storing maximum energy is like finding the holy grail. Recent breakthroughs involve materials such as silicon in the anode rather than traditional graphite.
The inclusion of silicon has shown tremendous potentialâsilicon can hold up to ten times more lithium ions than graphite. Not to mention, improvements in lithium-sulfur technology are another avenue researchers are exploring. The key characteristic of these innovations is their ability to potentially double or triple the energy capacity of batteries.
However, these advancements donât come without a drawback; issues surrounding the cycling stability of these materials need sorting out. If researchers can resolve those, itâll mark a turning point in battery technology.
Commercialization Prospects
Commercialization prospects play a crucial role in determining which technologies will reach consumers. For these innovations to benefit everyday users, extensive testing, scaling production, and ensuring affordability are essential. Companies must consider both cost and practicality when evaluating new technology for market readiness.
Many industry giants are investing heavily in battery research, recognizing this is the tipping point to capture the electric vehicle market. The unique feature here is that while current options may be limited, leading firms are slated to potentially bring these higher density batteries into broader production, which could lower overall costs. However, as with any new technology, the challenge lies in maintaining a balance between innovation speed and quality assurance.
Integration of Renewable Resources
Integrating renewable resources into battery technology offers paths to not only improve the performance of electric vehicles but also align with global sustainability goals. This process encompasses methods of charging and overall energy production.
Solar-Powered Charging Solutions
Solar-powered charging solutions are becoming increasingly popular and are an essential part of future trends. By coupling electric vehicles with solar technology, we can harness the sunâs energy to recharge batteries. This integration reduces dependence on grid electricity and offers a cleaner charging source.
The critical characteristic is the ability to transform sunlight into electric charge effectively. This innovation is not just beneficial; itâs smart from an environmental angle. Transitioning to this kind of charging solution could empower consumers to charge vehicles with zero emissions. Of course, one must also consider the common concern of energy storage for nighttime use. Yet, by combining advanced battery technologies, this challenge can be addressed.
Life Cycle Assessment
Life cycle assessment (LCA) examines the environmental impacts of a battery throughout its existenceâfrom production to disposal. Understanding these factors is crucial when considering sustainable practices in battery technology. An LCA provides insights into the actual footprint of battery production and usage, helping to identify areas for improvement.
What makes LCA significant is its comprehensive view of sustainability. It highlights both the energy inputs required for batteries and the end-of-life disposal options available. This understanding can lead to better designs and processes that minimize environmental harm. However, the barriers to implementing findings from LCA can also be substantial, as changing established practices often meets with resistance.
By focusing on these trends, the industry not only enhances electric vehicle technology but also ensures that future advancements are sustainable and viable for the planet. In this rapidly evolving field, every step toward improvement brings the world closer to a greener future.
Culmination
In this article, weâve navigated the intricate landscape of electric car batteries, examining their composition and numerous facets that define their operation and impact. The significance of understanding electric car batteries cannot be overstated, as these components play a crucial role in shaping the future of transportation and sustainability.
Key Elements of the Conclusion
- The exploration of anode and cathode materials highlights the essential chemistry involved in energy storage.
- Understanding battery types illuminates not just their present applications but also potential advancements.
- A deeper knowledge of manufacturing processes and recycling methods is vital for addressing environmental concerns regarding battery waste.
Recognizing these factors leads to a more informed approach to developing better, safer, and more efficient batteries, ensuring that both current and future technologies align with sustainability goals.
"Advancements in battery technology represent not just a step forward in electric mobility but a leap towards a more resilient and connected world."
A comprehensive grasp of these components enriches discussions around the electric vehicle industry, leading to calculated decisions that not only foster innovation but also consider ethical implications.
Summary of Key Points
To sum it all up, here are the core takeaways:
- Electric car batteries consist of critical components such as anodes, cathodes, electrolytes and separators that each serve unique functions in energy storage and transfer.
- Different battery types, including lithium-ion and solid-state batteries, offer various benefits while presenting unique challenges.
- Manufacturing and recycling processes are integral not only to battery performance but also to their environmental impact, emphasizing the need for responsible practices.
Looking Ahead
As we venture into the future, the analysis of trends within battery technology will play a pivotal role. Innovations are excitingly on the horizon:
- Research into energy density enhancements could lead to longer-lasting batteries, reducing the frequency of recharges and ultimately benefiting consumers.
- The integration of renewable energy sources into charging solutions emphasizes a shift towards sustainable practices.
- Regulatory frameworks and governmental policies will likely influence the direction of battery technology development, making it essential for stakeholders to stay informed of changes in industry standards.
The road ahead offers vast potential for innovation and growth, shaping not just how we drive, but how we view our relationship with energy and the environment.
As electric car batteries continue to evolve, their contributions will undoubtedly fuel advancements across various sectors, rendering them not just components, but key players in the movement towards a sustainable future.