Exploring the Human IgG Sequence and Its Significance
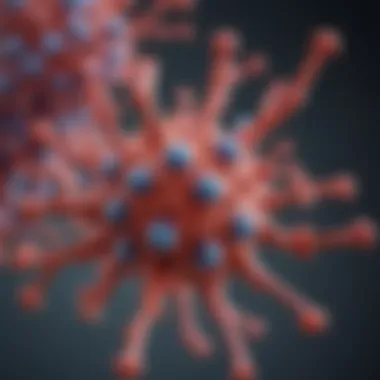
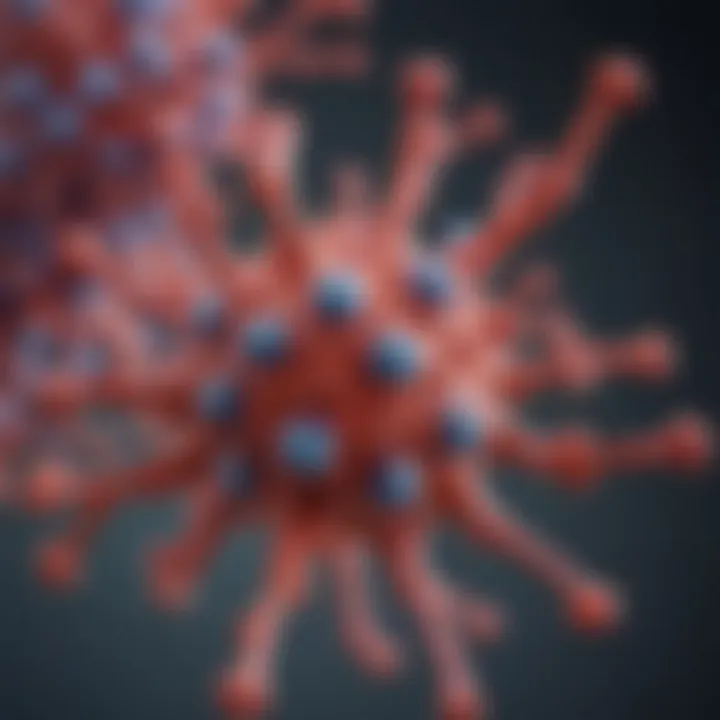
Intro
Human Immunoglobulin G (IgG) is a crucial player in the immune system, acting as the body's first line of defense against pathogens. This article takes a closer look at the structure and function of the IgG sequence, exploring how its intricate design contributes to immunological functions, including neutralization of toxins and pathogens.
From evolution to clinical applications, the conversation will flow through various aspects of IgG, addressing why understanding its nuances is not only valuable for researchers but also essential for healthcare professionals and students.
You might wonder about the importance of IgG subclasses, their unique roles in immune responses, or how sequence variations can impact therapeutic outcomes. These elements play a significant part in both health and disease management, making it vital to dive into the depths of human IgG to appreciate its full significance.
Research Overview
Methodological Approaches
To study IgG effectively, researchers employ diverse methods, ranging from genetic sequencing to structural biology techniques. Techniques such as mass spectrometry and x-ray crystallography are particularly useful in examining the conformations and interactions of IgG molecules.
- Sequencing - High-throughput sequencing can reveal variations in IgG genes, leading to insights about population-level immune characteristics.
- Structural Analysis - Advanced imaging techniques allow for detailed visualization of IgG's molecular architecture, revealing how its structure relates to function.
This confluence of methodologies fosters a better understanding of how IgG operates within the human body and its potential applications in medicine.
Significance and Implications
The implications of researching IgG are far-reaching. Understanding the sequence can:
- Improve vaccine design.
- Enhance monoclonal antibody therapies.
- Inform personalized medicine approaches.
Essentially, a deeper insight into IgG can pave the way for innovative treatment modalities for various diseases, including autoimmune disorders and infections.
Current Trends in Science
Innovative Techniques and Tools
In today's scientific landscape, the advent of bioinformatics has transformed the way researchers analyze IgG sequences. Tools that allow for rapid comparisons of IgG alleles across populations can uncover hidden trends and vulnerabilities within immune responses. Techniques such as CRISPR-Cas9 are revolutionizing IgG studies, enabling precise modifications that can lead to breakthroughs in immunotherapy.
Interdisciplinary Connections
The study of IgG does not exist in a vacuum. There is a growing recognition of the interplay between immunology and other disciplines like:
- Genomics - Understanding genetic influences on IgG production.
- Pharmacology - Examining how IgG handles drugs and therapies.
- Pathology - Investigating IgG roles in various diseases.
Such interdisciplinary collaboration enriches the research environment and leads to multifaceted approaches to health and disease management.
"The sequence of human IgG is not just a string of amino acids; it is a vibrant narrative of the immune response that has developed over millennia."
Overall, this article aims to provide a meaningful look into the Human IgG sequence, its significance, and its implications in health and disease. Whether you're a student diving into immunology or a seasoned researcher seeking fresh insights, the intricate details of IgG merit exploration.
Prolusion to Human IgG
The ensuing sections shall dig into the intricacies surrounding the human Immunoglobulin G (IgG) sequence, a key player in our immune system that works behind the scenes to preserve our health. Understanding human IgG is not just for the biology buffs; it has real-world implications in clinical settings, vaccine development, and therapeutic interventions. This introduction sets the stage by illuminating the building blocks of IgG and its pivotal role in our defense mechanisms, serving as an essential baseline for the elaborate details to come.
Overview of Immunoglobulins
Immunoglobulins, commonly known as antibodies, are specialized proteins produced by plasma cells to combat pathogens. Think of them as your body's surveillance system, equipped with the ability to identify and neutralize foreign invaders, such as bacteria and viruses. Here's a summary of some critical aspects:
- Diverse Functions: Each antibody type has unique roles; while IgG handles the heavy lifting in most immune responses, others like IgM and IgE tailor responses to specific threats.
- Functional Diversity: Antibodies can neutralize toxins, opsonize pathogens for phagocytosis, and activate other immune system components—an orchestra of defense strategies.
- Biological Significance: The presence of antibodies is often a marker of an immune response. For instance, high levels of IgG might indicate past infections or vaccinations.
To understand human IgG deeply is to grasp the several nuances of immune responses that occur throughout our lifetime.
What is IgG?
IgG, or Immunoglobulin G, occupies a prominent place among the immunoglobulin classes. It is the most abundant antibody in serum, accounting for about 75-80% of total immunoglobulins. Some crucial points about IgG include:
- Structure: IgG is characterized by its distinct Y-shape structure composed of two heavy chains and two light chains, setting it apart from other antibody classes. The architecture of IgG allows it to effectively bind to antigens, which is essential for their neutralizing functions.
- Subclasses: IgG is further divided into four subclasses: IgG1, IgG2, IgG3, and IgG4. Each plays a different role in immune responses, adapting to various pathogenic contexts.
- Responses: It's not just a numbers game; IgG responds specifically to antigens, enabling long-term immunity after an infection or vaccination. This adaptability makes it a focus for many therapeutic applications.
Understanding IgG requires delving into its multifunctional aspects and appreciating how it weaves into the larger fabric of the immune system, a narrative that unfolds throughout this article.
Structure of Human IgG
Understanding the structure of Human IgG is paramount in appreciating its diverse functions in the immune system. The uniqueness of IgG structure plays a significant role in how it interacts with antigens, pathogens, and other immune components. This section aims to dissect the intricate makeup of IgG and highlight how these features contribute to its overall efficacy in immune responses.
Basic Structural Components
At its core, Immunoglobulin G consists of four polypeptide chains: two heavy chains and two light chains. Each of these chains is linked together by disulfide bonds, creating a Y-shaped molecule that is vital for its function. The heavy chains are longer, while the light chains are shorter, and they give rise to distinct regions termed Fab (antigen-binding fragment) and Fc (crystallizable fragment).
- The Fab regions are crucial for binding to specific antigens, enabling the immune system to recognize and neutralize pathogens. This specificity arises during the development of B cells, influenced by somatic hypermutation, which refines the affinity of antibodies to their respective antigens.
- The Fc region, on the other hand, is significant for recruiting other components of the immune system, like complement proteins and effector cells. Its structure facilitates opsonization and immune signaling, each critical for effective immune engagement.
These structural features not only define the functionality of IgG but also pave the way for its subclasses to exhibit distinct roles in immune responses.
Fab and Fc Regions
Delving deeper, the differentiation between the Fab and Fc regions unveils a wealth of information about how IgG operates. The Fab regions contain the antigen-binding sites, which are each comprised of a combination of variable and constant regions.
- Within the variable regions, unique amino acid sequences enable the diverse binding capabilities of IgG. Consequently, each IgG can target an array of pathogens with specific binding strengths. This diversity is akin to having a locked toolbox—only the right key can turn the lock.
- The Fc region serves as a beacon for immune system activity. It interacts with Fc receptors on various immune cells, facilitating processes such as antibody-dependent cellular cytotoxicity and phagocytosis. The molecular architecture of the Fc region, especially its glycosylation patterns, can also influence the binding affinity to these receptors, thereby altering the efficiency of the immune response.
This functional duality is remarkable; the ability to both neutralize pathogens directly and coordinate further immune action underscores the versatility of IgG.
Glycosylation Patterns
The glycosylation of IgG is another key aspect that cannot be overlooked. These carbohydrate moieties attached to the Fc region play a critical role in modulating the stability and function of IgG.
- The presence and type of glycan structures can influence IgG’s interactions with Fc receptors, ultimately modulating biological responses such as antibody-dependent cellular cytotoxicity.
- Alterations in glycosylation patterns may also reflect pathological states. For instance, changes in glycosylation can occur in various diseases, offering a unique biological marker that can be used in diagnostic applications.
Overall, comprehending the structural intricacies of Human IgG reveals why this antibody is an indispensable component of the immune arsenal, laying the foundation for its many roles ranging from pathogen neutralization to therapeutic applications.
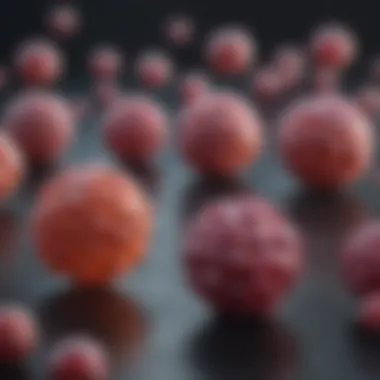
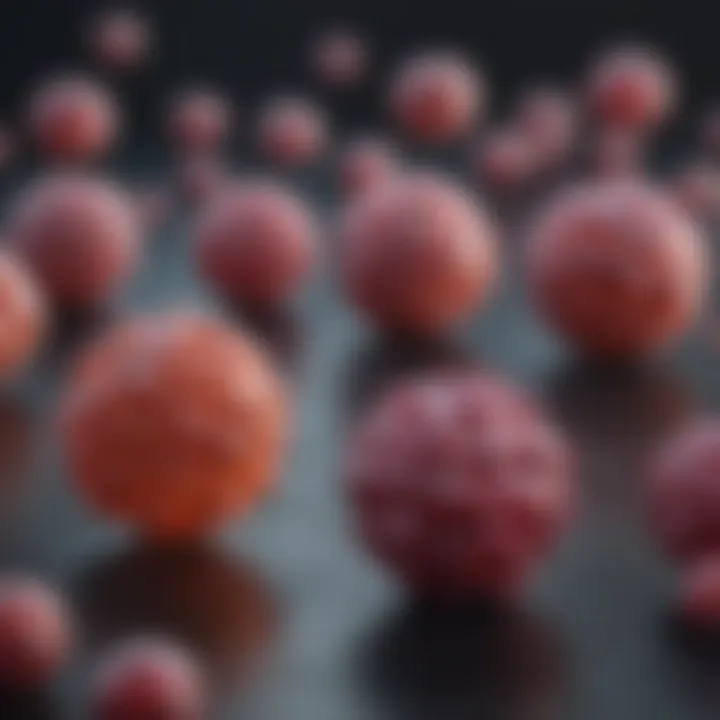
"The structure of IgG is not merely a molecular curiosity; it is the blueprint for one of the immune system's most critical players."
This detailed insight into the structure reinforces our understanding of IgG’s functionality, and sets the stage for further exploration of its roles in human health and disease.
Functional Roles of IgG
The functions of Immunoglobulin G (IgG) are intricate and multifaceted, making it a cornerstone of the human immune response. IgG is not merely a passive player; it is actively engaged in combating pathogens, which is fundamental to maintaining health. Delving deeper into the roles IgG plays reveals a layer of complexity that is critical to understand, especially for those studying immunology.
Neutralization of Pathogens
One of the primary roles of IgG is the neutralization of pathogens, including bacteria and viruses. This is achieved through several mechanisms. When a pathogen enters the body, IgG antibodies can bind to specific antigens on the surface of these invaders. This binding effectively blocks the pathogen's ability to attach to host cells, preventing infection.
Moreover, IgG can neutralize toxins released by pathogens. Toxins can wreak havoc on bodily functions, and the timely action of IgG can thwart these hazardous effects. For instance, during a bacterial infection, specific IgG molecules might directly bind to harmful toxins, rendering them inactive.
In addition to direct neutralization, IgG can also mark pathogens for destruction through a process known as opsonization, which leads us to the next crucial function of IgG.
Activation of Complement Cascade
The second significant role of IgG involves the activation of the complement cascade, a series of proteins that play a vital part in the immune response. When IgG binds to antigens on the surfaces of pathogens, it creates a complex that can trigger the complement system.
The complement system consists of numerous proteins that, once activated, can lead to the lysis (bursting) of pathogens, opsonization for easier elimination, and promotion of inflammation to recruit additional immune cells. This cascade amplifies the immune response, making it more robust than it otherwise would be. The synergy between IgG and the complement system exemplifies how the body orchestrates a coordinated defense against infections.
Here are some key points about the complement activation:
- Pathway Initiation: IgG activates the classical pathway due to its ability to bind with antigen, thus initiating the complement cascade.
- Opsonization: The activated complement components enhance the efficiency of phagocytosis by coating the pathogens, making them more recognizable to immune cells.
- Inflammatory Response: Complement proteins stimulate the release of inflammatory mediators that attract immune cells to the site of infection.
Opsonization Mechanism
Opsonization is a vital process where IgG plays a leading role by enhancing the ability of phagocytes, such as macrophages and neutrophils, to engulf and destroy pathogens. When IgG binds to a pathogen, it tags it for destruction, acting like a neon sign indicating, "Here I am!" for the immune system.
This mechanism performs a dual function: it not only marks the pathogen but also facilitates its recognition by phagocytic cells. These immune cells possess receptors that specifically bind to the Fc region of IgG. This interaction triggers a cascade of intracellular signals that culminate in the phagocytosis of the opsonized pathogen.
To emphasize the significance of this process, consider the following points:
- Enhanced Efficiency: Opsonized pathogens are engulfed more readily by phagocytes compared to uncoated pathogens.
- Phagocyte Activation: The binding of IgG to its receptor tends to activate the phagocyte, boosting its microbial killing capabilities.
- Memory System: Opsonization also aids in developing immunological memory, paving the way for more expedient responses in future infections by the same pathogen.
"Through various mechanisms, IgG fulfills its mission as a critical agent of immune protection, showcasing the body's intricate ability to defend against diseases."
In summary, the functional roles of IgG are indispensable to the immune system. By neutralizing pathogens, activating complement, and enhancing opsonization, IgG provides robust protection, showcasing the dynamic nature of our immune defense.
Human IgG Subclasses
Understanding the subclasses of Immunoglobulin G (IgG) plays a crucial role in appreciating the immune response in humans. Each subclass has its own unique characteristics that contribute to its function in the immune system. This section will explore these subclasses in detail, shedding light on their distinct roles and how they collectively enhance our immune defense.
Overview of Subclasses
Human IgG consists of four subclasses: IgG1, IgG2, IgG3, and IgG4. All these subclasses share a common structure but differ in their amino acid sequences, which leads to variations in their biological activities.
- IgG1 is the most abundant, making up about 70-75% of serum IgG. Its ability to bind effectively to Fc receptors on immune cells enables it to trigger potent immune responses.
- IgG2 primarily responds to polysaccharide antigens, particularly those found on the surface of bacteria. This subclass is particularly crucial for defending against encapsulated bacteria.
- IgG3 is unique in its structure, featuring a long hinge region that imparts flexibility. This special structure allows IgG3 to form stronger immune complexes, effectively neutralizing pathogens.
- IgG4 stands out due to its unique properties. It can engage in Fab-arm exchange, where half of the antibody can swap with another Fab-arm, leading to a reduced capacity to activate complement. This subclass plays a significant role in passive immunity.
IgG1: The Dominant Subclass
As the dominant subclass, IgG1 is pivotal in immune protection. Its high affinity for various antigens makes it particularly effective in neutralizing viruses and bacteria. IgG1’s ability to fix complement enhances its capability to opsonize pathogens, tagging them for destruction by phagocytes.
This subclass provides robust immunity against a vast array of pathogens and is often the focus in vaccine development due to its efficiency in mounting a strong immune response. Moreover, its engagement with receptors like Fc gamma R1 allows for the activation of effector functions, consolidating its status as the frontline antibody in responses against infections.
IgG2: Response to Carbohydrate Antigens
IgG2's role is largely tied to the response against polysaccharide antigens found in bacterial capsules. The subclass excels in responding to antigens that have molecular structures that are more complex than simple proteins.
This subclass is vital in the body’s defense against encapsulated pathogens such as Streptococcus pneumoniae and Haemophilus influenzae. Interestingly, IgG2 is slower to be produced in response to a new infection compared to IgG1, but once produced, it provides substantial and long-lasting immunity against such bacteria.
IgG3: Role in Immune Complexes
The unique structural attributes of IgG3 contribute to its significant role in producing immune complexes. The longer hinge region found in IgG3 allows for greater flexibility, which enhances the antibody's ability to cross-link antigens, forming stable complexes with pathogens.
These immune complexes activate complement effectively, leading to inflammation that recruits immune cells to the site of infection. However, the expansive immune response driven by IgG3 means that it must be regulated carefully to prevent excessive inflammation that could damage healthy tissue.
IgG4: Unique Properties and Passive Immunity
IgG4 is an interesting player in the IgG family, particularly noted for its ability to perform Fab-arm exchange. This means that it can form bispecific antibodies, ultimately displaying reduced adaptive immunogenicity when compared to the other subclasses.
This characteristic helps reduce inflammation, allowing IgG4 to play a counterbalancing role especially in chronic allergic responses or in immune tolerance.
In cases of passive immunity, IgG4 can be transferred from mother to fetus, providing crucial protection, especially in early life. However, its role is complex, as while it can neutralize certain antigens, it does so with less effectiveness in activating complement than its counterparts.
The understanding of IgG subclasses is crucial to developing targeted therapeutics and vaccines, revealing their individual roles can inform personalized medicine approaches.
By dissecting the unique aspects of each subclass, we not only gain insights into their individual functionalities but also how they cooperate to mount an effective immune response.
Genetic Basis of Human IgG Sequences
Understanding the genetic foundation of human IgG sequences is akin to unlocking a door into the immune system's inner workings. It provides vital insights not only into how IgG molecules are produced but also into how variations can affect immune responses. At the core, IgGs are generated by a series of complex genetic processes that highlight the intricate funtioning of our immune system.
This section will delve into two critical aspects: the genomic organization of immunoglobulin (Ig) genes and the processes of somatic hypermutation and affinity maturation. Each of these contributors plays a pivotal role in the robust and dynamic nature of IgG responses. The implications of understanding these processes are vast. From therapeutic interventions to vaccine efficacy, the genetic aspect of IgG sequences bears relevance across multiple fields, striking chords with students, researchers, and healthcare professionals alike.
Genomic Organization of Ig Genes
The genomic structure of immunoglobulin genes is a fine-tuned orchestration of multiple components. Located on chromosomes 14, 2, and 22, these genes are meticulously arranged to facilitate the diverse antibody repertoire an individual can generate.
Key components include:
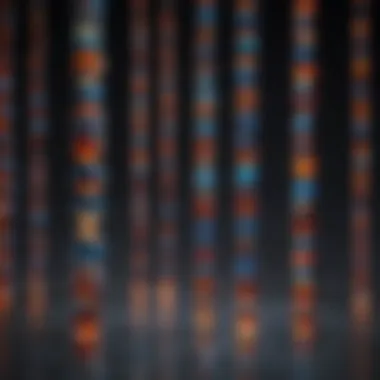
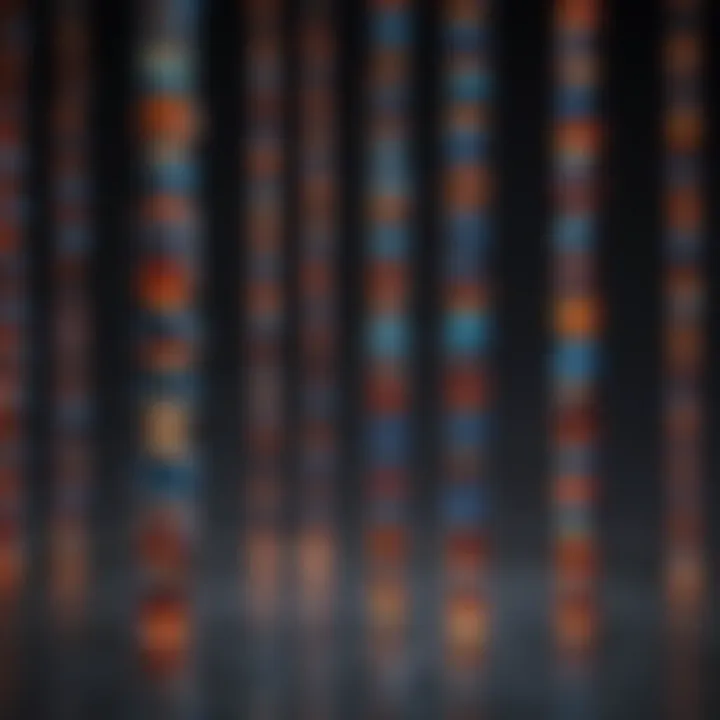
- Variable (V) segments: These are responsible for the diverse recognition of antigens. Genes in the V segment undergo recombination to create variable regions in the antibodies.
- Diversity (D) segments: Particularly important in heavy-chain antibodies, these segments increase the combinatorial diversity.
- Joining (J) segments: These segments link V and D segments, contributing further to antibody diversity.
- Constant (C) regions: These determine the class of the antibody — as seen in IgM, IgA, IgG, etc.
The splicing and joining of these segments through a process called V(D)J recombination provide the foundation for generating unique antibodies capable of recognizing a vast array of antigens. This genetic framework is not only significant for individual immune responses but also evolves over generations, adding a layer of adaptability to our immune system.
Somatic Hypermutation and Affinity Maturation
Moving beyond the basics, somatic hypermutation is where the fine-tuning truly occurs. This process happens after B cells encounter an antigen and begins to proliferate. As these cells multiply, mutations occur at a high rate specifically within the variable region of the Ig genes. Each mutation attempts to enhance the affinity of the antibody for its specific antigen.
This process is complemented by affinity maturation, which goes through a selection mechanism. In essence, B cells producing antibodies with higher affinities are more likely to survive and proliferate, while those with lower affinities undergo apoptosis. This mechanism is crucial for refined immune responses, ensuring that the antibodies produced are not just numerous but also highly effective against pathogens.
"Somatic hypermutation not only increases the affinity of an antibody for its target but also establishes a robust adaptive response essential for long-term immunity."
To wrap it all up, the genetic underpinnings of IgG sequences not only emphasize the complex nature of immune responses but also present opportunities for therapeutic and clinical applications. As we peel back the layers of IgG genetic frameworks, we gain both foundational insights and innovative potential for applications in healthcare.
Variability and Evolution of IgG Sequences
Understanding the variability and evolution of Immunoglobulin G (IgG) sequences is essential for interpreting the immune response and its adaptability over time. IgG demonstrates a remarkable degree of diversity, resulting from factors such as somatic hypermutation and gene rearrangements. These processes allow the immune system to fine-tune its antibody responses to effectively confront an ever-evolving array of pathogens. This section explores the evolutionary origins of IgG along with the impact of pathogen pressure on its variation.
Evolutionary Origins of IgG
The journey of IgG begins with the broader class of immunoglobulins present in early vertebrates. Approximately 500 million years ago, the common ancestors of jawed vertebrates evolved a more sophisticated immune system, leading to the emergence of the adaptive immune response. Researchers believe that this evolution was triggered by the need to combat increasingly complex and diverse pathogens.
A vital aspect of this evolutionary journey is the gene rearrangement mechanism known as V(D)J recombination. This process enables the generation of a vast repertoire of antibodies from a limited number of immunoglobulin gene segments. In simpler terms, it's like mixing and matching pieces of a puzzle to create countless unique pictures – each one representing a slightly different antibody.
Key points about the evolution of IgG:
- Inception of the Adaptive Immune System: The adaptive system relies on IgG for a specific and strong response to foreign invaders.
- Gene Rearrangement: Mechanisms like V(D)J recombination create diversity in antibodies.
- Survivability: The ability to produce a wide range of immunoglobulins increases the chances of survival against various pathogens.
Impact of Pathogen Pressure on Variation
Pathogen pressure plays a significant role in shaping the variability of IgG sequences. In essence, as pathogens evolve, the immune system must adapt to recognize and neutralize new threats. This ongoing struggle between pathogens and host immunity leads to a dynamic process of selection, where certain IgG variants are favored over others.
When faced with specific pathogens, particularly those that frequently change their surface components—like the influenza virus or HIV—IgG sequences must adapt rapidly. This adaptability isn’t just important; it’s a matter of life and death for the organism. The phenomenon can be likened to a game of chess, where both the attacker and defender are constantly strategizing and countering moves.
In examining this dynamic:
- Natural Selection: IgG variants that can effectively bind to a varying pathogen will have a distinct survival advantage.
- Somatic Hypermutation: This process further enhances the affinity of antibodies towards specific antigens, improving the likelihood of effectively neutralizing pathogens.
- Diverse Pathogen Landscape: The presence of different pathogens in an environment contributes to the variation in IgG, effectively shaping the antibody landscape.
"The evolutionary arms race between pathogens and host immune systems is a fascinating dance of survival, driving the remarkable diversity we see in IgG today."
Laboratory Methods for Analyzing IgG
The exploration of human Immunoglobulin G (IgG) necessitates meticulous laboratory methods for analysis. These methods provide essential insights into the IgG structure, function, and its variants. Understanding these techniques is paramount as it not only aids researchers in their studies but enhances clinical applications relating to immunity and disease.
Analyzing IgG has therapeutic implications, particularly in diagnosing autoimmune conditions or monitoring responses to vaccination. With the rise of personalized medicine, accurate IgG quantification and characterization becomes even more vital. Let us delve into three prominent laboratory methods employed in the analysis of IgG.
Enzyme-Linked Immunosorbent Assay (ELISA)
The Enzyme-Linked Immunosorbent Assay, popularly known as ELISA, stands out as a fundamental tool in immunology. This method is pivotal in quantifying specific IgG antibodies in clinical samples.
Key components of ELISA include:
- Antigen Coating: The target antigen is bound to a plate, ideally a 96-well format, allowing easy multiple sample analysis.
- Patient Sample Addition: Serum or plasma samples containing potential antibodies against the antigen are added.
- Detection: After the binding of IgG to the antigen, a secondary antibody linked to an enzyme is introduced. This enzyme catalyzes a colorimetric reaction that correlates with the amount of IgG present.
Benefits of using ELISA:
- High specificity and sensitivity make it an ideal choice for detecting low antibody levels.
- The method is relatively straightforward and can be tailored to various targets, from viral pathogens to autoimmune markers.
However, practitioners must consider factors like sample storage conditions, a calibration curve for quantitation, and the specificity of the antibodies used to avoid cross-reactivity and false positives.
Western Blotting Techniques
Western blotting is another impactful method for the analysis of IgG. Often deployed after electrophoresis, this technique helps in identifying specific proteins within a sample. When analyzing IgG, it allows for the visualization of these antibodies and assessing their molecular weight.
The steps usually involved in western blotting are:
- Sample Separation: Proteins are separated using gel electrophoresis based on size.
- Transfer: Proteins are then transferred onto a membrane to preserve their structure for further analysis.
- Detection: Using antibodies specific to the target IgG, a visual signal (usually a color change or luminescence) is generated—allowing identification and quantification.
Western blotting benefits from high specificity akin to ELISA, but it demands substantial procedural expertise and can be time-consuming. Furthermore, the requirement for specific antibodies may complicate the analysis if those antibodies are not readily available or reliable.
Mass Spectrometry in IgG Analysis
Mass spectrometry (MS) has emerged as a cutting-edge technique that provides extensive details about IgG molecules. It is particularly adept at delineating complex mixtures and characterizing the glycosylation patterns crucial for IgG function.
How mass spectrometry operates:
- Ionization: The IgG samples are ionized to generate charged particles that can be manipulated in an electric or magnetic field.
- Mass Analysis: The mass-to-charge ratio of these ions is measured, providing detailed information about the molecular weights and structures.
- Data Interpretation: Advanced software analyzes the resulting spectra to extract various characteristics of the antibodies, including their subclasses and post-translational modifications.
Mass spectrometry offers unique benefits:
- It can provide comprehensive data in terms of the structural and functional aspects of IgG.
- Additionally, it can help identify variants that have significant implications in disease progression and treatment responses.
Yet, the complexity of the technology and the cost involved may be a hurdle for many laboratories, limiting its routine application.
Overall, each of these laboratory methods—ELISA, Western blotting, and mass spectrometry—offers distinct advantages and limitations. Choosing the appropriate technique depends on the specific research or clinical need, as well as the available resources.
Clinical Significance of IgG Variants
The clinical relevance of IgG variants is a multifaceted topic that holds significant implications for diagnostics, treatment strategies, and the management of various health conditions. Understanding these variants not only helps in comprehending the immune response but also aids in unveiling possible pathways for therapeutic intervention, particularly in the realms of autoimmune diseases, infectious diseases, and vaccine efficacy.
Autoimmune Disorders Linked to IgG
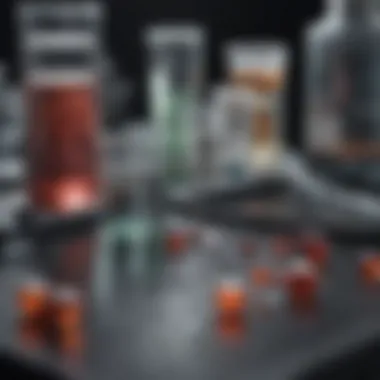
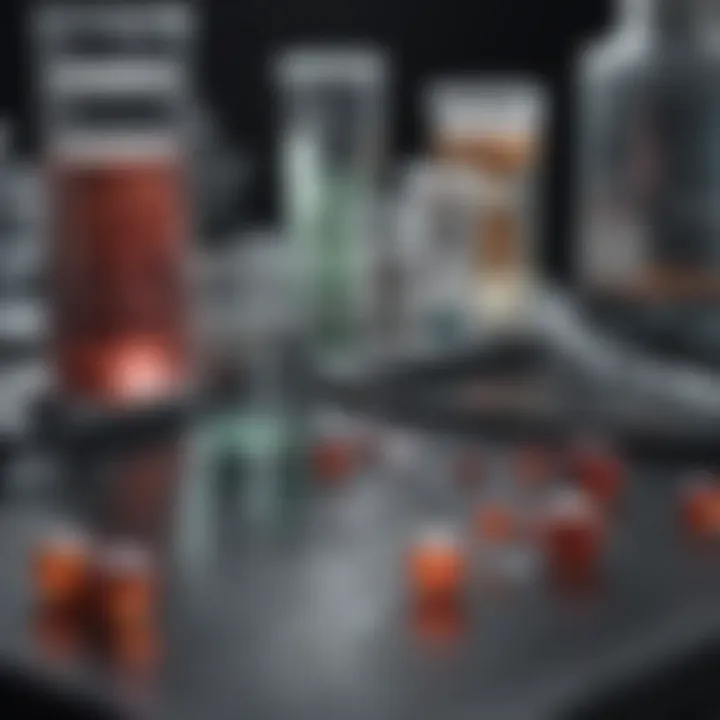
Autoimmune disorders often stem from a misdirected immune response, where the body's own antibodies mistakenly target healthy cells. IgG variants have been implicated in these disorders due to their diverse functionality and potential reactivity with self-antigens.
- Rheumatoid Arthritis: In this condition, IgG antibodies are produced against various components of joint tissue. The presence of specific IgG variants can serve as biomarkers, indicating disease progression and treatment response.
- Systemic Lupus Erythematosus (SLE): SLE is characterized by the production of autoantibodies, including IgG, against nuclear antigens. Variants of IgG may influence the severity of the disease and guide personalized treatment approaches.
Individuals diagnosed with autoimmune conditions may benefit from monitoring IgG variant levels, as this could identify alternative therapeutic avenues and empower patients amidst their health challenges.
Infectious Diseases and IgG Response
IgG plays a crucial role in the body’s defense against pathogens. Variability in IgG sequences can impact the effectiveness of immune responses to infectious agents.
- Pathogen Recognition: Pathogens, such as bacteria, viruses, and fungi, may present unique surfaces that elicit specific IgG responses. Variants in IgG can create a diverse arsenal of antibodies, capable of recognizing and neutralizing these varying threats.
- Predictive Value in Infections: Certain IgG variants may predict susceptibility or resistance to infections. For example, individuals with specific IgG subclasses or alleles might respond differently to the same infection, influencing both the severity of the disease and the recovery time.
Much of the ongoing research aims to tailor treatments based on the IgG profiles of individuals with infectious diseases, presenting possibilities for more effective interventions and improved health outcomes.
IgG in Vaccine Response
Vaccination is a fundamental tool in preventing infectious diseases, and IgG responses are critical to vaccine efficacy. The presence of certain IgG variants following vaccination can indicate the strength and longevity of an immune response.
- Seroconversion: Successful vaccination typically leads to an increase in specific IgG levels, signifying an adequate immune response. Variability in IgG sequencing can influence how well an individual’s immune system responds post-vaccination.
- Diverse Efficacy: The response to vaccines is not uniform across the population, with some individuals exhibiting robust IgG responses, while others may not produce substantial levels. Identifying the IgG variants associated with strong vaccine responses can illuminate factors that enhance or limit vaccine effectiveness.
In sum, the clinical significance of IgG variants is profound and multifarious. By bridging the gap between immunology and clinical practice, these variants pave the way for personalized medicine, offering targeted solutions to improve health outcomes across various conditions.
"Understanding the significance of IgG variants is pivotal, not just for unraveling immune response complexities but also for tailoring medical strategies that align with individual patient needs."
The exploration of IgG variants presents an intriguing frontier in medical research and clinical application, ultimately contributing to a deeper understanding of health and disease.
Therapeutic Applications of IgG
The significance of IgG in therapeutic contexts cannot be overstated. This section marks a deep dive into the utilization of IgG in modern medicine. Immunoglobulin G serves not only as a sentinel in immune responses but is a linchpin in various therapeutic avenues. As advancements in biomedicine continue to come about, the therapeutic applications of IgG have paved the way for innovative treatments, offering new hope for patients around the globe.
Monoclonal Antibodies in Medicine
Monoclonal antibodies (mAbs) are tailored proteins derived from a single clone of B cells. These antibodies are a prime example of the sophisticated use of human IgG in therapy. By leveraging the body’s own immune mechanism, these mAbs can be designed to target specific antigens, whether that be cancer cells or pathogens.
Here’s why the importance of mAbs can’t be overlooked:
- Specificity: mAbs are engineered to bind precisely to their specific target. This specificity helps in reducing collateral damage to healthy cells, which is a common adverse effect in many traditional treatments.
- Versatility: They can be used in a variety of contexts, from oncology to autoimmune diseases. For instance, therapies targeting CD20 in certain lymphomas have shown success rates that leave many oncologists optimistic.
- Replaceable: With ongoing research, new mAbs can continually be developed to meet rising challenges posed by both existing and new diseases.
Moreover, the versatility of monoclonal antibodies is showcased in their application. They might be used alone or in combination with other treatments, augmenting the overall efficacy of therapeutic regimens.
Intravenous Immunoglobulin Therapy
This form of therapy involves administering pooled IgG antibodies through the veins, a method used for a wide array of conditions. It’s particularly beneficial for patients who have deficiencies in their immune systems or certain autoimmune disorders.
Key elements of intravenous immunoglobulin therapy include:
- Broad Spectrum Activity: By providing a multitude of antibodies, IVIG can help modulate immune responses and neutralize a broader range of pathogens at once.
- Reduction of Inflammation: This therapy has the unintended benefit of balancing aberrant immune activities, which is crucial in diseases characterized by excessive inflammatory responses, like Guillain-Barré syndrome.
- Life-Saving Potential: In cases of severe immune deficiency, this therapy can provide immediate, albeit temporary, immunity. This is essential in protecting vulnerable patients against infections while additional measures are established.
In summary, the therapeutic applications of IgG, particularly through monoclonal antibodies and intravenous immunoglobulin therapy, exemplify the power of harnessing the body's own defenses to treat formidable diseases. This intersection of immunology, engineering, and medicine represents a significant leap forward in health care, with implications reaching beyond the horizons of traditional medicine.
"Immunoglobulins are not just antibodies; they are a lifeline for many in the face of severe immunological challenges."
The realm of IgG is indeed vast, and as research continues, we may uncover even more innovative strategies to optimize its therapeutic potential.
Future Perspectives in IgG Research
Exploring the future perspectives in IgG research is crucial to understanding how these immune proteins can be further leveraged in both clinical and laboratory settings. As research continuously evolves, new technologies, strategies, and methods are emerging that promise to enhance our comprehension of IgG sequences and their functional nuances. The implications span far beyond academic interest; they hold significant potential for improving health outcomes and personalizing medical interventions.
New Technologies for IgG Sequencing
Recent advancements in sequencing technologies have revolutionized the way we explore IgG sequences. High-throughput sequencing methods, including next-generation sequencing (NGS), allow researchers to delve deeper into the variability of IgG genes at a dizzyingly fast pace. These state-of-the-art technologies enable more comprehensive profiling of IgG against various diseases, which contributes to understanding the immune response much more holistically.
Some of the prominent technologies under the spotlight include:
- Single-cell sequencing: This allows the analysis of IgG sequences from individual immune cells, offering insights into clonal diversity and the evolutionary history of antibody responses.
- CRISPR-Cas9 based editing: Researchers are utilizing CRISPR technology not only for editing genes but also for fine-tuning IgG expression in experimental models.
- Bioinformatics tools: Sophisticated algorithms are being devised to analyze large datasets, helping in the identification of critical patterns and correlations in IgG sequences and their efficacy.
With these technologies, we can anticipate better insights into IgG variability and functionality, fostering enhanced strategies for vaccine design and therapeutic antibody production.
Personalized Medicine Opportunities
The recent exploration of personalized medicine hinges on the premise that treatment can be tailored to individual genetic make-up and immune profiles. In the context of IgG, this means that by understanding a patient’s unique IgG sequence, researchers can predict responses to infections and vaccines. For instance, when a patient presents with an autoimmune disorder, clinicians could analyze specific IgG variants and adjust treatment strategies based on their findings.
Moreover, personalized medicine could open doors for:
- Targeted immunotherapy: IgG therapeutics can be crafted to better match an individual’s immune system, improving efficacy and minimizing side effects.
- Vaccine optimization: Tailored vaccines could be developed to elicit the most effective IgG response based on an individual’s genetic predispositions.
- Predictive analytics: By associating specific IgG sequences with disease susceptibility or treatment responses, healthcare providers can adopt a preventive rather than reactive approach.
"Personalized medicine synergizes with recent findings in IgG research, not only transforming treatment paradigms but emphasizing the individuality of immune responses."
Epilogue
In wrapping up the exploration of the human IgG sequence, it’s vital to underscore its central role within the immune system. IgG is not simply a component; it is a cornerstone, providing a robust response to pathogens and ensuring the body's defense mechanisms operate effectively. The understanding of IgG extends beyond basic immunology; it carries implications for therapeutic strategies, vaccine development, and even personalized medicine.
Summary of Key Findings
A series of notable observations cut across the discussions concerning IgG, including:
- Structural Complexity: Human IgG is characterized by its unique structure, comprising variable and constant regions that facilitate its diverse functions against infections.
- Functional Diversity: Each subclass of IgG plays distinctive roles in the immune response. For instance, IgG1 leads in neutralizing pathogens, while IgG3 is instrumental in forming immune complexes.
- Genetic Variability: The genetic mechanisms, such as somatic hypermutation and class switching, underpin the adaptability of IgG responses to changing pathogenic challenges. This variability is not merely academic; it's a critical aspect of how the immune system learns and evolves.
- Clinical Implications: Emerging insights into IgG variations inform the understanding of autoimmune diseases, infections, and treatment methodologies, offering avenues for precise health interventions.
Overall, these findings highlight the multifaceted nature of IgG, positioning it as a crucial element in the interplay between health and disease.
Implications for Future Research
Looking ahead, the exploration of IgG presents numerous avenues for future research. Some of the key implications include:
- Advancements in Sequencing Technologies: As sequencing techniques continue to evolve, understanding the scopes of IgG genes could lead to novel therapies and targeted treatments, particularly in immune-mediated diseases.
- Personalized Medicine: With the growing trend toward tailored medical approaches, further investigation into individual IgG profiles might yield personalized strategies for vaccinations and therapies, enhancing efficacy and reducing adverse reactions.
- Pathogen Interaction Studies: Understanding how different pathogens affect IgG responses will be crucial for designing effective vaccines and therapeutics. This research can illuminate the affectivity of IgG subclasses in varying infections.
- Autoimmunity Research: Gaining a deeper insights into the correlation between IgG variants and autoimmune disorders could pave the way to better management and treatment options for affected individuals.
By embracing these research directions, scientists and medical professionals can move towards richer understandings of immune functions, laying the groundwork for next-generation immunotherapies.