Exploring Human Total RNA: Structure, Function, Applications
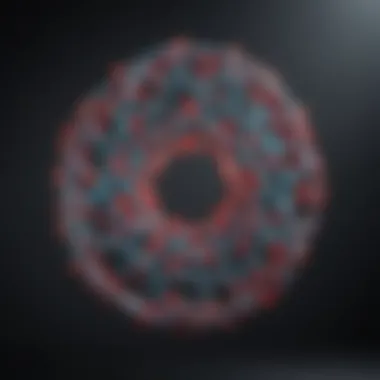
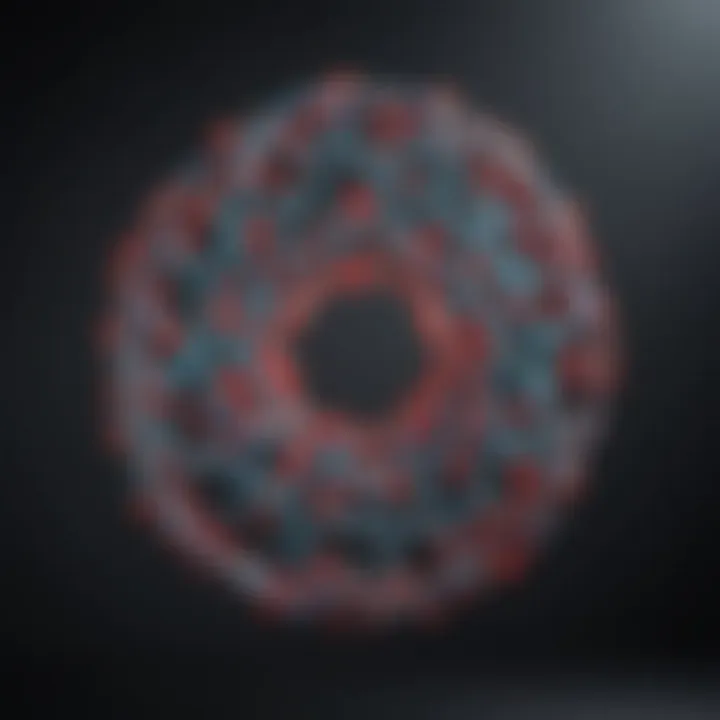
Intro
Human total RNA plays a fundamental role in the functions and processes of cells. Understanding its structure, types, and the roles it performs is crucial for various scientific fields, especially genetics and biotechnology. Total RNA includes messenger RNA (mRNA), ribosomal RNA (rRNA), and transfer RNA (tRNA), among others. Each type has distinct functions, such as coding for proteins and aiding in protein synthesis.
The extraction of total RNA is a significant procedure in molecular biology research. Quality assessment of RNA is essential, as it affects subsequent experiments and analyses. Advancements in RNA research continue to reveal its importance in diagnostics and therapeutics, leading to exciting possibilities in medical applications. This article aims to explore various facets of human total RNA, its applications, and the ongoing advancements in understanding its roles.
Research Overview
Methodological Approaches
Research on human total RNA relies on several methodologies to extract and analyze RNA samples. Different extraction techniques are pivotal in ensuring that high-quality RNA is obtained. Common methods include phenol-chloroform extraction, column-based purification, and more recent advancements involving magnetic bead technology. Each approach has its advantages and limitations in terms of yield, purity, and time efficiency.
Once extracted, RNA quality assessment tools like spectrophotometry and gel electrophoresis help ascertain RNA integrity. These methods are crucial for researchers to ensure that their analyses will produce reliable results. The choice of extraction and assessment methods impacts the overall conclusions that can be drawn from RNA research.
Significance and Implications
The significance of understanding human total RNA cannot be overstated. It serves various functions essential for cellular operations and gene regulation. Moreover, insights into RNA composition can lead to breakthroughs in disease mechanisms and therapies. For instance, studying mRNA in the context of cancer can help in identifying potential biomarkers and therapeutic targets.
"Understanding RNA is not merely an academic exercise; it has real-world implications for health and disease."
The implications of total RNA research extend into diagnostics. RNA biomarkers can provide important information about diseases, helping in early detection and targeted therapies. Furthermore, RNA therapeutics, such as RNA interference and CRISPR technologies, are at the forefront of innovative treatment approaches.
Current Trends in Science
Innovative Techniques and Tools
Recent advancements in sequencing technologies, such as RNA sequencing (RNA-seq), have revolutionized the analysis of total RNA. RNA-seq enables a comprehensive view of the transcriptome, revealing both known and novel RNA molecules. This technique enhances the ability to study gene expression patterns, alternative splicing, and non-coding RNAs, thus broadening our understanding of RNA's functional repertoire.
Additionally, advancements in bioinformatics tools enable more sophisticated analyses of RNA. These tools facilitate the processing and interpretation of vast datasets generated by RNA sequencing.
Interdisciplinary Connections
Total RNA research intersects with multiple disciplines, including genomics, proteomics, and systems biology. By integrating data from different fields, researchers can develop a more holistic view of biological processes. The connection between RNA and other molecular components enriches the understanding of cellular dynamics and can lead to collaborative efforts aimed at addressing complex biological questions.
Prolusion to Human Total RNA
Understanding human total RNA is essential for various fields, from molecular biology to clinical diagnostics. Total RNA encompasses all types of RNA within a cell, which play pivotal roles in the biological processes that sustain life. This section introduces the significance of total RNA, pointing out its intricate involvement in gene expression and regulation.
Moreover, this overview will address why systematically studying total RNA provides insight into cellular function, disease mechanisms, and potential therapeutic targets. Total RNA serves as both a marker of cellular health and a participant in cellular processes, making it a valuable area of research.
Definition and Importance of Total RNA
Total RNA refers to the complete set of RNA molecules present in a cell at a given moment. This includes messenger RNA (mRNA), transfer RNA (tRNA), ribosomal RNA (rRNA), and non-coding RNAs. Each type of RNA has unique roles but collectively, they function in various cellular activities such as protein synthesis, regulation of gene expression, and maintenance of cellular integrity.
The importance of total RNA cannot be overstated. It not only reflects the gene expression profile of a cell but also plays a significant role in the regulation and adaptation of cellular responses to environmental changes. Furthermore, abnormalities in RNA expression patterns can be linked to diseases, highlighting the necessity of total RNA analysis in clinical settings.
Overview of RNA Types
mRNA
Messenger RNA is crucial for the transfer of genetic information from DNA to the protein synthesis machinery in a cell. mRNA acts as a template during translation, guiding the assembly of amino acids into proteins. Its key characteristic is the ability to carry specific genetic instructions tailored for each gene. This dynamic functionality makes mRNA a popular focus in research, especially in the context of gene expression studies. However, mRNA can be quite unstable, which presents challenges in its analysis and reliability in some settings.
tRNA
Transfer RNA is integral to the translation process, linking codons in mRNA to the corresponding amino acids during protein synthesis. tRNA's unique cloverleaf structure enables it to carry amino acids to the ribosome effectively. This RNA type is essential for accurate protein formation, ensuring that the correct sequence is established. A notable advantage of tRNA is its high specificity for particular amino acids, but it can also be constrained in its availability, impacting translation efficiency under certain conditions.
rRNA
Ribosomal RNA is the primary component of ribosomes, the cellular machines that synthesize proteins. rRNA facilitates the interaction between tRNA and mRNA, playing a structural and catalytic role in protein synthesis. Its abundance in the cell and essential nature in ribosome function illustrates why it is vital for life. A consideration with rRNA is its stability, which can make it a useful biomarker in assessing cellular health, but it is often overlooked in favor of more directly related RNAs like mRNA.
Non-coding RNAs
Non-coding RNAs, a diverse group of RNA molecules that do not translate into proteins, are gaining attention for their regulatory roles in gene expression and cellular processes. They are classified into various categories, including small interfering RNAs, long non-coding RNAs, and microRNAs. These RNAs are essential for maintaining cellular processes and preventing gene overexpression or silencing when needed. Their complexity presents both a unique opportunity for novel therapeutic strategies and challenges in deciphering their precise functions and interactions within the cell.
Structure of Human Total RNA
The structure of total RNA in humans plays a crucial role in its functionality. Understanding RNA structure helps in grasping how it participates in various biological processes. Total RNA encompasses different types of RNA, each contributing uniquely to cellular functions. Analyzing its structure not only aids in comprehension of gene expression, but also in the discovery of potential therapeutic and diagnostic avenues.
Key Components of RNA
The functional roles of total RNA are deeply interconnected with its structural components. Three primary elements define RNA structure: nucleotide composition, secondary structure, and post-transcriptional modifications. Each element provides insights into the overall significance of RNA in human health and disease.
Nucleotide composition
Nucleotide composition is fundamental to the structure of RNA. It primarily consists of four nucleotides: adenine, guanine, cytosine, and uracil. This composition ensures that RNA can perform a variety of tasks within cells. The unique feature of uracil, instead of thymine which is found in DNA, gives RNA distinct properties. The presence of these nucleotides facilitates the formation of RNA's diverse structures. This characteristic makes nucleotide composition essential in RNA's functionality. However, variations can affect overall stability and reactivity of RNA.
Secondary structure
The secondary structure of RNA refers to the way nucleotides pair up to form double-stranded regions, loops, and bulges. This folding is crucial because it dictates RNA's functionality. The unique feature of intramolecular base pairing allows for complex shapes. This property is beneficial as it enhances stability and informs interactions with proteins. However, intricate folding can lead to misfolding problems, which may result in dysfunctional RNA. Understanding these structures can provide insights into how RNA performs specific roles in cellular mechanisms.
Post-transcriptional modifications
Post-transcriptional modifications include a variety of alterations that occur after RNA is synthesized. These modifications, such as methylation or pseudouridylation, play a significant role in regulating RNA stability, localization, and translation efficiency. The unique feature of these modifications is their ability to impact RNA function in multiple ways. Importantly, they can enhance the resilience of RNA against degradation. On the downside, excessive or inappropriate modifications can hinder RNA's functionality, leading to cellular dysregulation.
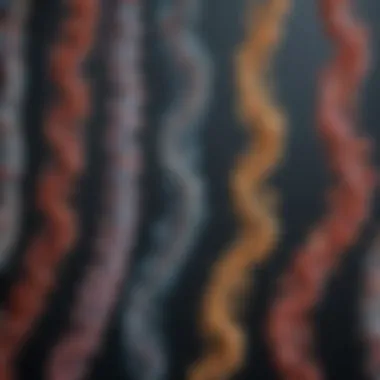
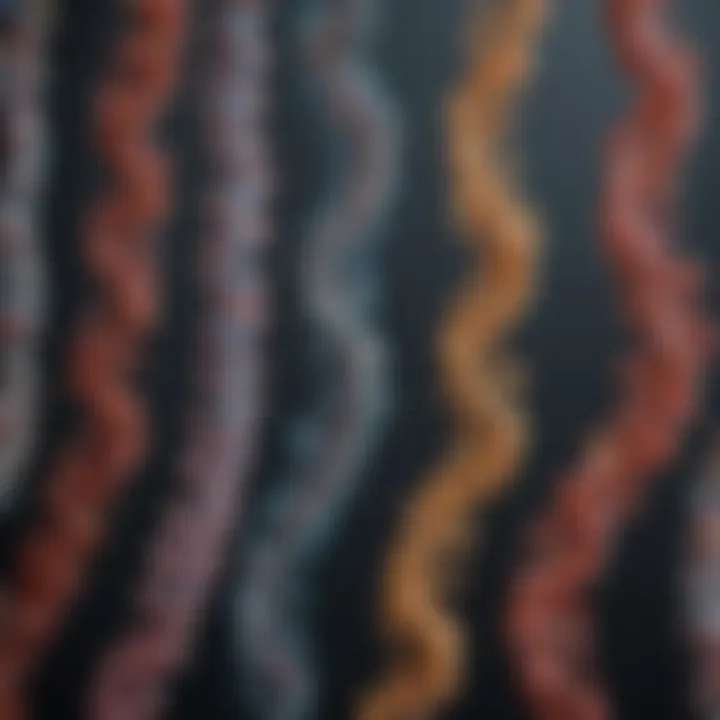
"Understanding the structure of total RNA deepens insights into its diverse functionalities and impacts on cellular dynamics."
The exploration of these three critical components reveals their intertwined nature in shaping the roles of RNA in human cells. Understanding these elements not only fosters appreciation for RNA's complexity but also paves the way for innovative research and applications.
Functions of Total RNA in Human Cells
The functions of total RNA within human cells are fundamental to understanding biological processes. Each type of RNA plays a specific role that ensures cellular activities run smoothly. Total RNA is critical for gene expression, cellular signaling, and regulatory processes. It connects genetic information stored in DNA with the functional requirements of the cell.
Role in Gene Expression
Transcription process
The transcription process is essential for gene expression. In this phase, messenger RNA (mRNA) is synthesized from a DNA template. This method is notable because it accurately reflects the genetic code while allowing for modification. The key characteristic of transcription is that it generates an RNA strand complementary to the DNA sequence. This makes transcription a central focus in studies related to human total RNA.
Transcription is beneficial as it provides a direct mechanism for cells to express genes as needed. Unique features of this process include its transcription factors that regulate efficiency and the potential for various RNA editing. However, the transcription process can face challenges, such as mutations that lead to dysfunctional mRNA. This can result in disease and other implications in RNA research.
Translation mechanisms
Following transcription is the translation mechanism, where the ribosome decodes the mRNA sequence into a specific protein. Translation is critical because it is where the genetic code translates into functional proteins essential for cellular operation. The main characteristic here is the ternary complex formation involving mRNA, ribosomal subunits, and tRNA.
Translation represents a crucial aspect of cellular machinery. Its unique feature lies in how it utilizes transfer RNA (tRNA) to bring amino acids to the ribosome. This contributes to effective protein synthesis. However, challenges can arise during translation, such as premature termination or frameshifts, which can lead to dysfunctional proteins.
Involvement in Cellular Processes
Cellular signaling
Cellular signaling is another vital function performed by total RNA. It encompasses the communication pathways that inform cells about their environment. The role of RNA in signaling is often overlooked, yet it is integral for maintaining homeostasis. One key characteristic of cellular signaling is how RNA molecules can function as signaling molecules themselves. They can modulate various cellular functions through interaction with other biomolecules.
This aspect is beneficial as it enhances understanding of dynamic cellular responses. Moreover, understanding RNA in signaling could lead to targeted therapeutic interventions. Its unique features can include the versatility of RNA molecules in switching between different signaling pathways. However, there are disadvantages, such as potential miscommunication between cells leading to diseases.
Regulation of gene expression
The regulation of gene expression is another significant aspect of total RNA's function. This involves controlling the timing and rate of gene expression, which is crucial for development and response to internal and external stimuli. One notable characteristic is the role of small non-coding RNAs, which can directly influence gene expression post-transcriptionally.
These regulatory mechanisms enhance the adaptability of organisms to their environments. The unique aspect of this regulation involves feedback loops that can alter gene expression based on cellular conditions. Certain disadvantages include the complexity of regulatory networks, making them challenging to study and interpret, often leading to inconsistencies in data results.
"Understanding RNA functions is not only about basic science but also about unlocking new avenues for therapeutic intervention in diseases."
Broadly, the interplay of these functions of total RNA illustrates its fundamental role in biological processes. Key assessments of total RNA involvement in cellular functions enhance insights into human health and disease.
Methods for Total RNA Extraction
The extraction of total RNA is a critical step in various molecular biology applications. Understanding different methods for total RNA extraction enables researchers to obtain high-quality RNA, which is essential for downstream applications like gene expression analysis, sequencing, and more. With proper techniques, researchers can ensure the integrity of their samples, which influences the accuracy and reliability of their results.
Common Extraction Techniques
Phenol-chloroform extraction
Phenol-chloroform extraction is one of the oldest and most widely used methods for RNA extraction. This technique relies on the phase separation mechanism, where phenol and chloroform are used to separate nucleic acids from proteins and other contaminants. The key characteristic of this method is its effectiveness in isolating high-purity RNA from a variety of biological samples.
One of the main advantages of phenol-chloroform extraction is its high yield and purity of RNA. However, it can be hazardous due to the toxicity of both phenol and chloroform. Careful handling and proper safety measures are essential when using this method. Additionally, this technique may require more labor and time compared to newer extraction methods, which might limit its appeal in high-throughput scenarios.
Column-based methods
Column-based methods have grown in popularity for RNA extraction. This technique involves using a silica column that specifically binds RNA. The process is relatively quick and straightforward, which is why it has become a common choice among researchers. The key characteristic of column-based methods is their ability to effectively purify RNA while removing contaminants in a single step.
Column-based methods offer several advantages: they minimize the number of steps, reduce handling time, and are less hazardous than phenol-chloroform extraction. However, they may sometimes yield lower quantities of RNA compared to traditional techniques, making them less suitable for specific applications where high RNA yield is critical.
Magnetic bead separation
Magnetic bead separation is another contemporary method used for RNA extraction. In this technique, magnetic beads coated with oligo(dT) are used to capture poly(A) RNA from cellular samples. The unique feature of magnetic bead separation is its versatility, allowing researchers to isolate RNA from various sources, including difficult samples.
This method is beneficial for its rapid processing and high specificity for mRNA, making it a preferred choice, especially in studies focusing on gene expression. However, it may not be as effective for total RNA extraction from non-polyadenylated transcripts, such as rRNA and non-coding RNAs. Therefore, consideration of the intended application is necessary when choosing this extraction method.
Considerations for Sample Integrity
Maintaining the integrity of RNA samples is crucial for reliable analysis. Several factors can impact RNA quality during handling and storage. Researchers must pay attention to these considerations to ensure optimal results.
Sample handling
Proper sample handling is an essential aspect of RNA extraction. This includes factors like the temperature during extraction and the use of appropriate reagents. A key characteristic of good sample handling is minimizing the time between sample collection and RNA extraction, as RNA is highly sensitive to degradation.
Efficient sample handling can preserve RNA integrity and enhance the quality of downstream applications. However, the challenge lies in ensuring that extraction protocols are followed strictly, as any deviation can lead to sample loss or contamination, ultimately affecting the outcome of the research.
Contamination prevention
Contamination prevention is critical in total RNA extraction processes. The presence of contaminants, whether from reagents, equipment, or the environment, can compromise RNA purity and reliability. The key characteristic of effective contamination prevention involves using certified RNase-free tools and reagents throughout the extraction process.
One unique feature of this practice is the use of reagents that are specifically validated for RNA work. While successful contamination prevention contributes significantly to the overall quality of RNA samples, it requires diligence and attention to detail. Failure to adhere to these measures can result in inconsistent results, potentially leading to misleading conclusions in research studies.
Ensuring RNA integrity through proper extraction and handling practices allows researchers to obtain accurate and reproducible data.
Quality Assessment of Total RNA
Quality assessment of total RNA is a crucial step in molecular biology and genetics. It ensures that the extracted RNA meets the required standards for downstream applications such as sequencing and gene expression analysis. High-quality RNA is essential to obtain reliable results in experiments. Poor quality RNA can lead to inaccurate conclusions and wasted resources.
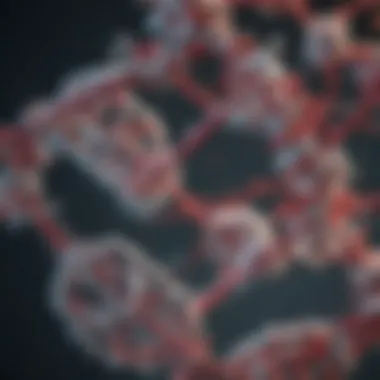
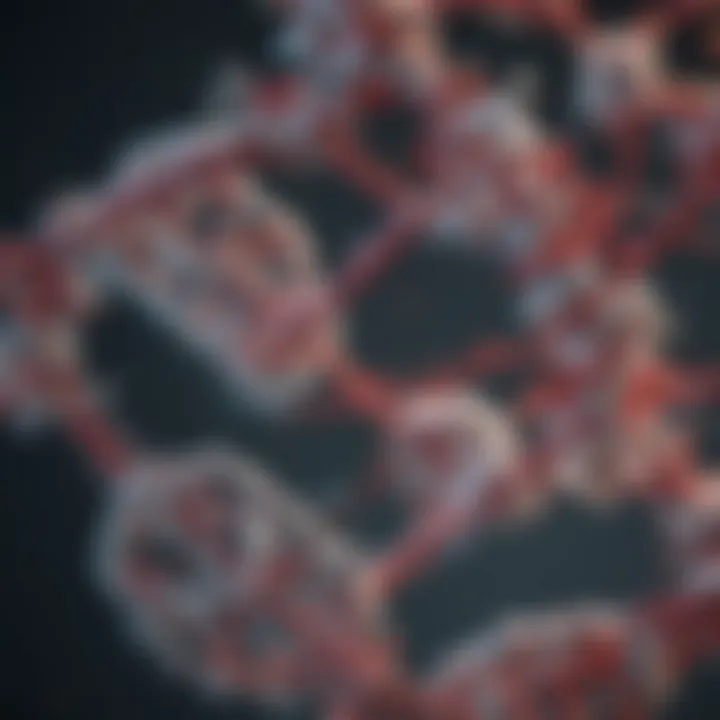
The significance of quality assessment lies in its ability to reveal the integrity and concentration of RNA samples. The evaluation techniques employed to assess RNA quality not only determine its usability but also help in troubleshooting issues related to sample degradation or contamination. Thus, understanding the best practices and methods in RNA quality assessment can enhance research outcomes across various scientific disciplines.
Evaluation Techniques
Spectrophotometry
Spectrophotometry is widely used to measure RNA concentration and purity. This technique relies on the absorbance of light at specific wavelengths to quantify RNA in a sample. One of the main benefits of spectrophotometry is its simplicity and speed. It is a non-destructive method that provides immediate results, making it a popular choice among researchers.
The unique feature of spectrophotometry is its capability to assess both concentration and purity. By measuring absorbance at 260 nm, researchers can determine the RNA concentration, while the ratio of absorbance at 260 nm to 280 nm indicates protein contamination.
However, there are limitations. Spectrophotometry cannot differentiate between different RNA types, such as mRNA and rRNA. Moreover, it can be affected by contaminants like phenol, which may lead to misleading results.
Agarose Gel Electrophoresis
Agarose gel electrophoresis is another critical technique for RNA quality assessment. This method allows visualization of RNA by separating the molecules according to size. The key characteristic of this technique is its ability to provide a qualitative assessment of RNA integrity.
Researchers can identify the presence of intact RNA bands or any degradation. The distinctive feature of agarose gel electrophoresis lies in its ability to reveal the size distribution of RNA molecules.
While it is a powerful method for detecting degraded RNA, agarose gel electrophoresis may not provide precise quantitative data. It is also time-consuming and requires interpretation of the gel images, which can sometimes lead to subjective conclusions.
Bioanalyzer Systems
Bioanalyzer systems are state-of-the-art tools designed to analyze RNA quality quickly and accurately. These systems use microfluidic technology for RNA analysis, providing high-throughput assessments. One significant advantage of bioanalyzer systems is their ability to deliver detailed profiles of RNA, including size, concentration, and integrity metrics.
The distinguishing feature is that they typically provide an RNA integrity number (RIN), which quantifies RNA quality on a scale from 1 to 10. A higher RIN indicates better sample quality.
Despite being highly effective, bioanalyzer systems can be costly and require specialized training to operate. Additionally, they may not be available in all laboratories, limiting accessibility for some researchers.
Interpreting Quality Metrics
Understanding how to interpret quality metrics is essential for accurate RNA analysis. This section discusses two key metrics: the RNA integrity number (RIN) and concentration assessment.
RNA Integrity Number (RIN)
The RNA integrity number, or RIN, is a valuable metric for assessing RNA quality. It provides a standardized score that reflects the level of RNA degradation. A higher RIN generally indicates intact RNA, suitable for experimental use.
RIN is beneficial because it simplifies RNA quality evaluations and supports reproducibility in results. One unique aspect of RIN is that it combines information from multiple bands in the gel, offering a comprehensive overview of sample integrity.
However, relying solely on RIN could overlook other important aspects of RNA quality. Hence, it is essential to consider RIN alongside other evaluation methods for a balanced assessment.
Concentration Assessment
Concentration assessment is a fundamental part of RNA quality evaluation. Accurate measurement of RNA concentration is critical for experimental design; it ensures that sufficient quantities of RNA are available for analysis.
One of the advantages of concentration assessment is its straightforward approach. Techniques like spectrophotometry provide instant feedback about RNA levels. The limitation here is that concentration alone does not guarantee the quality of RNA. A high concentration of RNA could still be degraded, which is why it is essential to combine concentration data with physical integrity observations.
Applications of Total RNA Analysis
The analysis of total RNA plays a crucial role in various fields of biomedical research and clinical applications. This section explores the multifaceted nature of RNA analysis and its implications in diagnostics and therapeutics. Understanding RNA's applications helps illuminate the potential it holds for disease understanding and treatment development. By focusing on diagnostic implications and therapeutic considerations, we can appreciate RNA's growing relevance in modern biomedicine.
Diagnostic Implications
Biomarkers for disease
Biomarkers are measurable indicators that reflect the presence or severity of a disease. In the context of human total RNA, specific RNA molecules can serve as effective biomarkers. These RNA biomarkers can indicate the onset of various diseases such as cancer, neurological disorders, and infectious diseases.
The ability to distinguish between healthy and diseased states through RNA profiling is a key characteristic of RNA biomarkers. They offer a sensitive mechanism for early detection, which is often crucial for effective treatment. Their non-invasive nature, especially when obtained from blood or other body fluids, makes them an attractive option for clinical settings.
Unique features of RNA biomarkers involve their capacity to reflect underlying biological processes. For example, changes in circulating microRNA levels have been linked to tumor progression. However, despite their potential, challenges such as variability among patients and the need for standardized protocols exist. This complexity underscores the importance of careful validation before applying RNA biomarkers in clinical practice.
RNA sequencing in clinical settings
RNA sequencing (RNA-seq) has emerged as a powerful tool for understanding gene expression patterns in various diseases. This technology enables comprehensive evaluation of RNA species, providing detailed insights into transcriptional changes that occur in disease states. The broad scope of RNA-seq allows for the identification of novel transcripts, mutations, and alternative splicing events, enhancing our understanding of disease mechanisms.
The distinctive capability of RNA sequencing lies in its ability to generate high-throughput data from millions of RNA molecules simultaneously. This feature contributes to its popularity among researchers seeking to unveil complexities of gene expression. Furthermore, its application in precision medicine can guide tailored therapies based on individual patient profiles.
Nevertheless, RNA sequencing also has its limitations. The process involves intricate data analysis and interpretation, demanding sophisticated bioinformatics expertise. Moreover, the cost associated with this technology can be significant, which poses challenges in resource-limited settings.
Therapeutic Considerations
RNA-based therapies
RNA-based therapies encompass a wide range of treatments that aim to modulate gene expression or function using RNA molecules. These include antisense oligonucleotides, small interfering RNA, and messenger RNA therapies. The potential of RNA-based therapies lies in their ability to target specific pathways involved in disease.
A primary characteristic of RNA-based therapies is their specificity. This allows for targeted interventions that can minimize off-target effects. Treatments like intron retention therapy have shown promise in genetic disorders by correcting splicing defects. This specificity makes such therapies a promising field in precision medicine.
The unique aspect of RNA-based therapies is their potential for rapid development. Unlike traditional protein-based therapies, RNA molecules can be synthesized relatively quickly. However, challenges include achieving sufficient delivery to target tissues and ensuring stability in biological environments. Balancing efficacy and safety remains an ongoing research focus.
Gene editing technologies
Gene editing technologies, particularly CRISPR-Cas systems, have transformed the landscape of genetic research and therapy. These methods enable precise modifications of genomic DNA and are gaining traction in various therapeutic contexts. RNA also plays a critical role in guiding these editing processes, as it serves as a template for specific targeting.
A key characteristic of gene editing is its precision, allowing for specific alterations that correct genetic defects. This is especially beneficial for inherited disorders and certain cancers. The adaptability of CRISPR technology to various cell types and organisms contributes to its appeal among researchers.
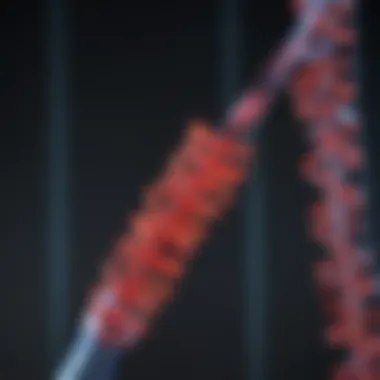
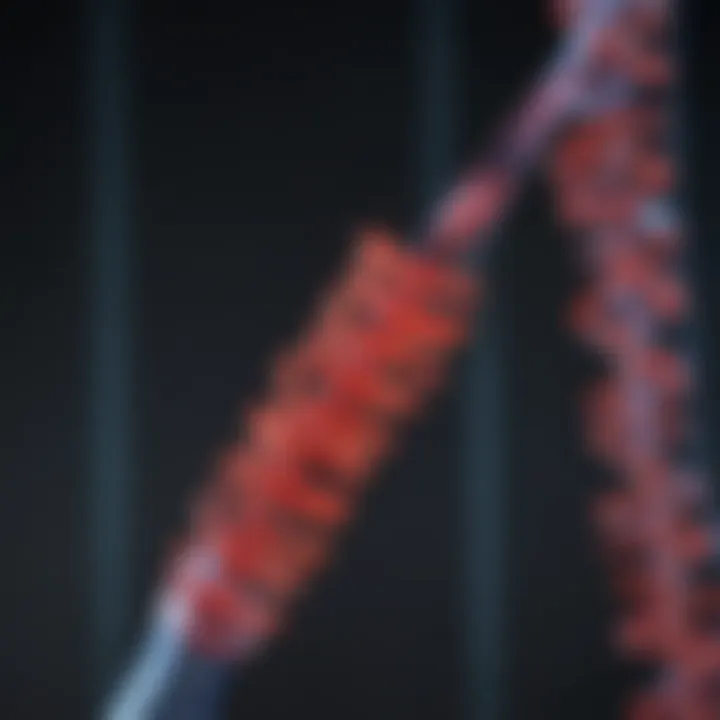
Nonetheless, the unique feature of gene editing technologies also presents significant ethical considerations. Off-target effects and the long-term consequences of genomic modifications are major concerns. Moreover, regulatory hurdles can slow down the translational application of these technologies to clinical settings. Thus, while gene editing holds great promise, it requires thorough examination for responsible and safe use.
In summary, total RNA analysis continues to evolve, shaping the landscape of diagnostics and therapeutics. The potential biomarker applications and innovations in RNA-based therapies herald a new era in personalized medicine, albeit with necessary caution and rigorous validation.
Challenges in Total RNA Research
Research on total RNA is a complex field that presents several challenges. Understanding these difficulties is important for improving methodologies, conducting reliable studies, and achieving accurate results. Many factors can complicate RNA research, but addressing these issues is essential for advancing our knowledge in molecular biology, diagnostics, and therapeutics.
Technical Limitations
Sample degradation
Sample degradation is a critical aspect of RNA research. It refers to the breakdown of RNA molecules, making them unusable for further analysis. This degradation can occur at multiple points, from sample collection to during storage and processing. This characteristic is vital as it affects the integrity of the total RNA. High-quality RNA is necessary for reliable experiments, especially those involving gene expression studies. One way to minimize sample degradation is to work quickly and use RNA stabilization solutions.
However, the unique feature of sample degradation is that it can vary significantly based on external conditions such as temperature and time. If RNA is exposed to high temperatures, enzymes known as RNases may break it down quickly. This can lead to disadvantages, such as misleading results in experiments. Therefore, understanding the conditions leading to degradation is essential in RNA research.
Variability in extraction efficiency
Variability in extraction efficiency is another issue in total RNA research. This refers to differences in the amount and quality of RNA that can be isolated from various biological samples. Factors like sample type, extraction method, and the experience of the technician can influence the efficiency of the extraction process. This characteristic indicates why it is crucial to optimize each step of the extraction protocol to achieve reliable results.
The unique feature of this variability is that it can affect reproducibility in experiments. An inconsistency in extracting RNA can lead to differing results in gene expression analysis. As a result, researchers may struggle to compare their findings with other studies. This variability becomes a significant challenge when trying to establish a standard method ensuring reliable outcomes in RNA analysis.
Interpretative Obstacles
Data complexity
Data complexity is a significant challenge in total RNA research. This complexity arises from the vast amount of information generated from techniques such as RNA sequencing and microarrays. A key characteristic of data complexity is the need for sophisticated computational tools to analyze and interpret the extensive datasets. Such complexity can limit the understanding of underlying biological processes. Thus, focusing efforts on developing effective bioinformatics solutions is vital to unravel the intricate RNA landscape.
One unique feature of data complexity is that it requires a multidisciplinary approach, combining biology, statistics, and computer science. This can be beneficial as various perspectives lead to more refined analysis methods and insights. However, the downside to data complexity is the steep learning curve for researchers who may not have strong computational skills.
Normalization issues in RNA analysis
Normalization issues in RNA analysis present another layer of complication. Normalization is the process of adjusting data from individual samples to allow for fair comparisons. Fluctuations in RNA input levels, amplification efficiencies, and other factors can result in biased data if not addressed. A key characteristic of normalization issues is that they can introduce significant variation in the interpretation of results.
The unique feature of these issues is that they can distort conclusions regarding gene expression levels. As a result, researchers may misinterpret findings if normalization is not adequately addressed. This highlights the necessity for consistent protocols during RNA analysis to ensure that results are accurate and comparable to other studies.
Future Directions in Total RNA Studies
The field of total RNA research is continually evolving, presenting numerous avenues for exploration and innovation. Understanding these future directions is crucial as they promise to enhance our comprehension of human biology and improve clinical practices. Emerging technologies and interdisciplinary approaches are at the forefront, allowing researchers to address unanswered questions and develop novel applications in diagnostics and therapeutics.
Emerging Technologies
Single-cell RNA sequencing
Single-cell RNA sequencing represents a significant advancement in the analysis of RNA. Unlike traditional bulk RNA sequencing, which averages gene expression across a population of cells, single-cell RNA sequencing allows for the examination of gene expression at the individual cell level. This high resolution provides insights into cellular heterogeneity, which is particularly important in complex tissues like tumors.
The key characteristic of single-cell RNA sequencing is its ability to profile thousands of genes in hundreds or thousands of single cells simultaneously. This is especially beneficial for understanding how different cell types respond to environmental changes, diseases, or treatments.
One unique feature of this technology is its application in identifying rare cell populations that may drive disease progression, which traditional methods might overlook. However, there are some disadvantages. These include high costs, complex data analysis, and the need for sophisticated computational tools to interpret the data adequately.
CRISPR and its implications
CRISPR technology has revolutionized our understanding of genetics and RNA’s role in gene regulation. CRISPR allows for precise editing of genetic sequences, including RNA. This can lead to significant breakthroughs in therapeutic strategies for genetic disorders.
The critical characteristic of CRISPR is its precision and efficiency in targeting specific RNA sequences for modification or knockdown. Its popularity in research stems from its versatility and potential impact on personalized medicine approaches.
A unique aspect of CRISPR is its capacity to facilitate functional genomics studies by enabling the systematic evaluation of gene function. The advantages, such as reduced off-target effects when compared to earlier gene-editing techniques, are significant. However, challenges remain, including ethical considerations and the need for targeted delivery systems in clinical settings.
Interdisciplinary Approaches
Integrating bioinformatics
The integration of bioinformatics in RNA studies has become indispensable. It enables researchers to manage and analyze the vast amounts of data generated by RNA sequencing technologies. With the complexities involved in interpreting gene expression data, bioinformatics tools provide a framework for understanding biological significance.
Bioinformatics is particularly beneficial because it allows for the incorporation of various data types, including genomic, transcriptomic, and proteomic data. This multimodal approach can yield insights into cellular functions and disease mechanisms. However, one of the noteworthy challenges is the steep learning curve associated with bioinformatics tools.
Collaboration with clinical research
Collaboration between RNA researchers and clinical professionals enhances the translation of RNA research into real-world applications. This interaction can guide the development of effective diagnostics and therapeutics based on RNA analysis.
A notable characteristic of such collaborations is their potential to expedite the research process by ensuring that findings are relevant to patient care. By involving clinical perspectives, researchers can address pressing health concerns more effectively.
The unique feature of integrating clinical insights into RNA research is the potential for rapid feedback loops, where research informs clinical practice and vice versa. However, a challenge in such collaborations can be the differences in priorities and timelines between researchers and clinicians, which must be navigated thoughtfully to achieve mutual goals.
"The future of RNA research is not just in methods, but in how we blend disciplines to push the boundaries of our understanding."
Finale
The conclusion serves a significant role in synthesizing discussions presented throughout this article on human total RNA. It encapsulates the complex topics, highlighting crucial aspects of RNA's structure, functionality, and its applications in modern science.
Total RNA is fundamental for understanding cellular mechanisms, for it operates at the core of gene expression. The article intricately outlines the diversity of RNA types—messenger RNA (mRNA), transfer RNA (tRNA), ribosomal RNA (rRNA), and non-coding RNAs—each contributing uniquely to cellular processes. This diversity emphasizes the importance of total RNA in regulating biological functions.
The methodologies for RNA extraction and quality assessment are pivotal in research and clinical applications. By discussing techniques such as phenol-chloroform extraction and bioanalyzer systems, the article prepares students and professionals alike to navigate the practical aspects of RNA studies. This knowledge is vital because the quality of RNA can significantly influence experimental results and clinical diagnostics.
Furthermore, the potential for total RNA analysis extends into diagnostics and therapeutics. Identifying RNA biomarkers for various diseases and leveraging RNA-based therapies illustrate the tangible impacts of this research field.
In reflecting on the challenges faced in total RNA research, the reader gains an appreciation for the complexities inherent in RNA analyses. Addressing technical limitations and interpretative obstacles is essential for advancing this field.
The discussion on future directions reveals promising avenues for exploration. Emerging technologies like single-cell RNA sequencing and interdisciplinary approaches integrating bioinformatics will likely propel RNA research into new heights. This opens doors to innovative methodologies, promising more precise understanding and application of RNA in health and disease.
In summary, the conclusion of this article emphasizes the critical role of total RNA in both basic biology and applied biomedical research. It seeks not only to inform but also to encourage further exploration and innovation in the realms of RNA studies and their multitudinous applications. The implications of future discoveries are profound, reiterating the importance of continued investment in this vital area of science.