Exploring Magnetic Alloys: Properties and Innovations
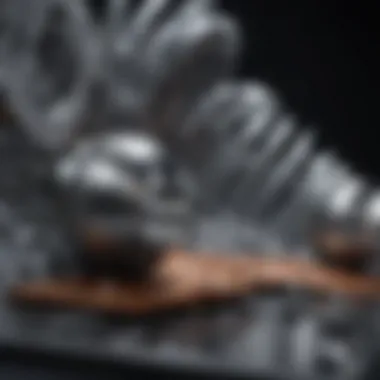
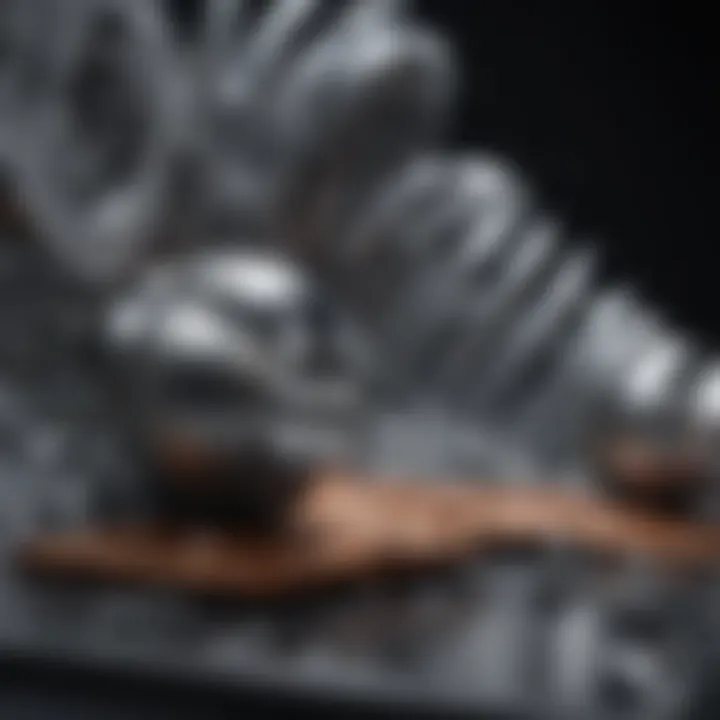
Intro
Magnetic alloys, comprising a unique blend of metals, exhibit properties that can be harnessed for various practical applications. A thorough understanding of these materials is essential, especially as industries constantly seek to innovate and optimize their processes. From electric motors to data storage systems, these alloys play a critical role in multiple sectors.
One might wonder why magnetic alloys are so fascinating or crucial. The answer lies within their distinct properties like coercivity, magnetization, and permeability. These characteristics are not just numbers or specs on a material datasheet; they have implications that can influence technology and our everyday lives in significant ways.
Interestingly, beyond their magnetic properties, the manufacturing processes and techniques also represent a rich field ripe for discussion. This exploration can guide informed decisions in both research and practical applications.
In this guide, we're not just aiming to scratch the surface. Instead, we will dissect the complexities, observe current trends, and predict future developments related to magnetic alloys.
Research Overview
Understanding the core nature of magnetic alloys begins with exploring the methodologies that researchers employ to study them. The following subsections will illuminate the research landscape surrounding these materials, delineating approaches that yield insights into their properties and applications.
Methodological Approaches
In the realm of studying magnetic alloys, a variety of methodological approaches are utilized. For instance:
- Characterization Techniques: Techniques such as X-ray diffraction and scanning electron microscopy help in understanding microstructural details.
- Magnetic Measurements: Techniques like vibrating sample magnetometry reveal crucial information about magnetization behaviors.
- Modeling and Simulation: Computational methods provide predictive insights into the behavior of these alloys under varying conditions.
This multi-faceted approach allows researchers to derive a more holistic understanding of the materials. New combinations of elements can lead to unexpected behaviors, emphasizing the importance of these varied techniques in uncovering information that may otherwise remain hidden.
Significance and Implications
The implications of research into magnetic alloys stretch far and wide. Understanding their properties directly influences technological innovation. For instance, the development of more efficient magnetic materials can lead to improvements in energy efficiency, advancements in electronics, and even progress in medical technologies. As industries grapple with the challenges of sustainability, innovations in magnetic alloy composition and application can contribute significantly to greener technologies.
"In the realm of technology, the quest for efficiency and effectiveness often hinges on understanding materials at a fundamental level."
In summary, magnetic alloys are not merely materials; their properties and the research surrounding them dictate the pace of innovation across many fields. The need for continual exploration and development in this area remains paramount, as does the understanding of its broader impacts.
Current Trends in Science
The scientific landscape surrounding magnetic alloys is dynamically evolving, often influenced by advancements in technology and cross-disciplinary dialogues. Here, we will review some current trends shaping the future of magnetic alloys research.
Innovative Techniques and Tools
The incorporation of new techniques in the study of magnetic alloys is transforming the research landscape. Emerging tools, such as:
- High-throughput material synthesis: Allows for rapid testing of combinations and properties.
- Cryogenic magnetic measurement techniques: Enable more precise determination of magnetic properties at low temperatures.
- Advanced computational methods: Simulating the electronic structure and magnetic properties at the atomic level sheds light on previously unexamined behaviors.
These advancements not only provide valuable insights but also accelerate the pace of discovery, making the field more dynamic than ever.
Interdisciplinary Connections
As magnetic alloys become increasingly crucial in various sectors, their study has begun to bridge different disciplines, merging physics, chemistry, and engineering. This interdisciplinary approach draws on insights from different fields, fostering innovation and creating new avenues for research.
Understanding how magnetic properties interact with other material characteristics could lead to breakthroughs in devices that rely on magnetic performance, such as energy storage systems, automotive components, and medical imaging technologies.
By navigating these connections, researchers and industry professionals can capitalize on the synergies found at the intersection of disciplines, propelling both scientific and practical advancements further.
Prologue to Magnetic Alloys
In an increasingly technological world, magnetic alloys play a significant role in driving innovation across various fields. These materials are more than mere combinations of metals; they are complex systems exhibiting unique properties that have direct implications on industries from electronics to medical devices. Understanding magnetic alloys goes beyond classroom memorization; it requires a grasp of how each alloy's properties can either enhance or constrain their application.
Magnetic alloys are specially formulated mixtures of two or more metals that display magnetic properties. This description barely scratches the surface, though. The breadth of their application warrants an examination of what defines them and how they evolved.
Defining Magnetic Alloys
Magnetic alloys are primarily used for their magnetic properties, which are derived from their elemental composition and the microstructural arrangement of these materials. They can be categorized based on their magnetic characteristics, like ferromagnetic or paramagnetic. Ferromagnetic alloys, such as various iron alloys, retain their magnetization after an external magnetic field is removed. This makes them vital for applications needing permanent magnets, like motors and loudspeakers. On the other hand, paramagnetic materials, though showing magnetism when subjected to an external field, lose this property once the field is gone. This distinction is critical when considering the application of these materials in technology.
From the everyday refrigerator magnet to complex magnetic sensors in automobiles, the versatility of magnetic alloys is a testament to their importance. Each application leverages different properties of these alloys, making understanding their composition and behavior essential for material scientists and engineers alike.
Historical Context
The journey of magnetic alloys is as fascinating as their scientific properties. Early discoveries in magnetism can be traced back to ancient civilizations that noted their effects but lacked an understanding of the underlying principles. The invention of the compass during the Han dynasty was probably one of the first instances where magnetic properties were harnessed for practical use.
Fast forward to the 19th century when scientists like Michael Faraday began to explore magnetism more scientifically. His work paved the way for the systematic exploration of magnetic materials. The first permanent magnet steels emerged around this time, with the first commercial applications appearing in electrical machines by the early 20th century.
The modern era has seen a remarkable evolution in understanding magnetic alloys. Post-World War II, the technological revolution led to heightened interest in electronic components, which in turn propelled research into alloys that could perform in increasingly demanding environments. As the demand for miniaturization and enhanced performance grows, so too does the global interest in advanced magnetic alloys.
"The history of magnetic alloys is a narrative of curiosity, innovation, and necessity, embodying the spirit of scientific discovery that drives technological advancements today."
Today, the continuous development of new types of magnetic alloys challenges traditional ideas and opens new pathways for research and application. From its rudimentary beginnings to the sophisticated material science of today, the history and definition of magnetic alloys reveal the intersection of historical necessity and futuristic innovation.
Understanding Magnetic Properties
Understanding the magnetic properties of alloys is like peeling an onion; it reveals layers of complexity that are integral to their industrial application. Magnetic properties define how materials respond to magnetic fields, influencing their performance in a variety of settings. For researchers and professionals alike, grasping these properties is vital as it dictates the suitability of a particular material for specific tasks. This section focuses on three prominent types of magnetism—ferromagnetism, paramagnetism, and diamagnetism.
Types of Magnetism
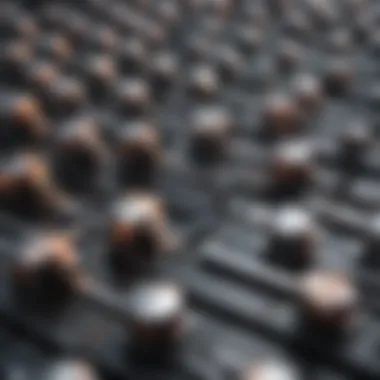
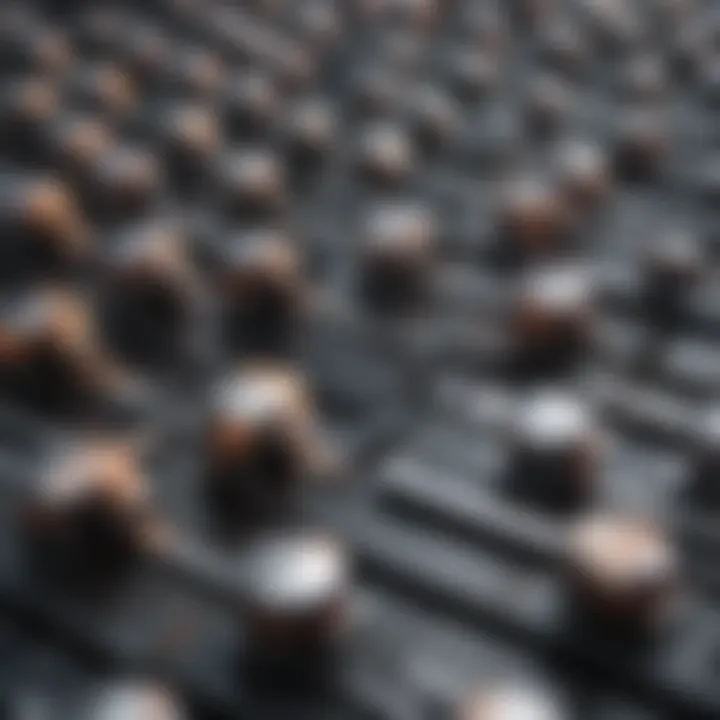
Ferromagnetism
Ferromagnetism is a fascinating characteristic prominent in materials like iron, cobalt, and nickel. This property arises from the alignment of magnetic moments of atoms in a specific, cooperative manner. One key feature of ferromagnetism is its ability to retain magnetization even in the absence of an external magnetic field. This permanent magnet behavior makes ferromagnetic materials quite useful, especially in applications like electric motors, sensors, and transformers.
The uniqueness of ferromagnetism also lies in its temperature-dependence. Below a certain temperature, known as the Curie temperature, the spontaneous alignment of magnetic moments occurs, allowing the material to become magnetized. However, if exposed to high temperatures, the alignment can break within the material, resulting in a loss of magnetism.
Advantages:
- Permanent Magnetization: This property is critical for applications that require a steady magnetic field.
- High Magnetic Susceptibility: Ferromagnetic materials respond strongly to external magnetic fields.
Disadvantages:
- Temperature Sensitivity: Their functionality can decrease significantly at elevated temperatures.
Paramagnetism
Moving on to paramagnetism, this property can be observed in materials that exhibit a weak attraction to magnetic fields. Unlike ferromagnetic materials, paramagnetic substances magnetize only in the presence of an external field. A distinctive characteristic of paramagnetism is its linear response to magnetic fields; when the field is removed, the material loses its magnetization quickly.
One of the fascinating aspects of paramagnetic materials is that they often contain unpaired electrons in their atomic structure. This means that while they do not form permanent magnets, they can still be influenced by external magnetic fields, albeit feebly. Common applications include certain medical imaging techniques and magnetic resonance imaging (MRI).
Advantages:
- Flexibility in Application: Paramagnetic materials are often used in unique environmental conditions without the risk of permanent magnetization.
Disadvantages:
- Weak Magnetization: Their effect is not significant, limiting their application in many heavy-duty scenarios.
Diamagnetism
Diamagnetism represents yet another unique type of magnetism, found in most materials to some degree. This phenomenon occurs when materials exhibit a very weak, negative response to external magnetic fields, effectively repelling them. The key characteristic of diamagnetism is its inherent nature—every material has some level of diamagnetic behavior, but only under specific conditions do these effects become noticeable.
Diamagnetic materials tend to have no unpaired electrons, and through this structure, they induce opposing magnetic fields in the presence of an external magnetic field. A notable example of a diamagnetic material is bismuth. The intriguing aspect of diamagnetic materials is their ability to levitate under strong enough magnetic fields, which has led to various experimental applications in physics.
Advantages:
- Non-Destructive: Unlike other magnetic materials, diamagnetic materials can be useful when precision is needed without residual magnetization.
Disadvantages:
- Limited Performance: The weakness of diamagnetism restricts its functional applications to specialized areas.
Measuring Magnetic Properties
Measuring magnetic properties is vital for understanding and optimizing the performance of magnetic alloys. Techniques such as vibrating sample magnetometry, superconducting quantum interference devices, or Hall effect measurements are routinely employed to assess how materials react to external magnetic fields. Some factors, like temperature and composition, can significantly affect these properties, and thus precise measurement methods are crucial. Without knowing how to gauge the magnetic behavior accurately, it would be challenging to innovate or improve upon existing materials in any meaningful way.
Composition and Microstructure of Magnetic Alloys
When it comes to magnetic alloys, the composition and microstructure are the two sides of the same coin. These aspects not only influence the material's magnetic properties but also its mechanical characteristics. A finely engineered composition lead to unique traits that make certain alloys particularly useful in various applications. Let’s take a closer look at the elements and structural aspects that contribute to the efficacy of these materials.
Common Elements in Magnetic Alloys
Iron
Iron is often the backbone of many magnetic alloys. It’s not just abundant in nature, but its magnetic properties are exceptional, especially in terms of ferromagnetism. One key characteristic of iron is its ability to enhance magnetic permeability when alloyed. This makes it a beneficial choice, particularly for electric motors and transformers.
However, iron has certain pitfalls. It is susceptible to oxidation, which can alter its magnetic properties over time. To mitigate this, alloying with other elements is common, helping to protect against corrosion while enhancing performance in devices. The unique feature of iron lies in its significant saturation magnetization, which is an advantage in high-efficiency applications.
Cobalt
Moving on to cobalt, this element plays a crucial role in improving the thermal stability of magnetic alloys. A key characteristic of cobalt is its high Curie temperature, which allows for effective magnetization at elevated temperatures. This makes cobalt an essential component in applications where heat is a factor, such as in aerospace technologies.
The unique feature of cobalt is its ability to create hard magnetic materials. However, cobalt's scarcity and cost can limit its usage in large-scale applications, presenting a downside. When paired with the right materials, cobalt offers a powerful combination of durability and magnetic strength, pushing the boundaries of innovation.
Nickel
Lastly, we can't overlook nickel. This element is well-known for its role in creating soft magnetic alloys. Nickel’s capacity for high saturation magnetization and ductility makes it a popular choice in applications requiring easy magnetization. This characteristic allows for efficient energy transfer in devices like inductors and transformers.
One unique aspect of nickel is its contribution to improving corrosion resistance, thanks to its passivation properties. But, it does have its drawbacks; nickel can exhibit a decrease in magnetic properties at very high temperatures, which must be taken into consideration during alloy design.
Microstructural Analysis
Microstructural analysis is another critical element in understanding the performance of magnetic alloys. The arrangement of particles, grain size, and phase distribution all play pivotal roles. These microstructural nuances can make or break the functionality of a magnetic material.
Grain Size and Shape
Grain size and shape contribute significantly to the alloy’s magnetic characteristics. Finer grains generally lead to superior magnetic performance because they provide a larger surface area for magnetic domain interactions. A key characteristic of smaller grains is that they reduce the energy barriers for magnetization, making it easier for the material to switch its magnetic orientation.
This leads to a unique feature of fine-grained materials: enhanced permeability and coercivity, which are crucial in applications like magnetic sensors. However, there is a trade-off; if grains are too small, they may become more susceptible to external disturbances, impacting stability.
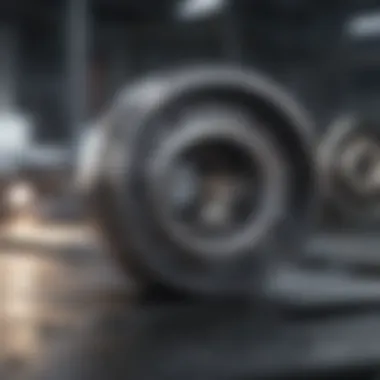
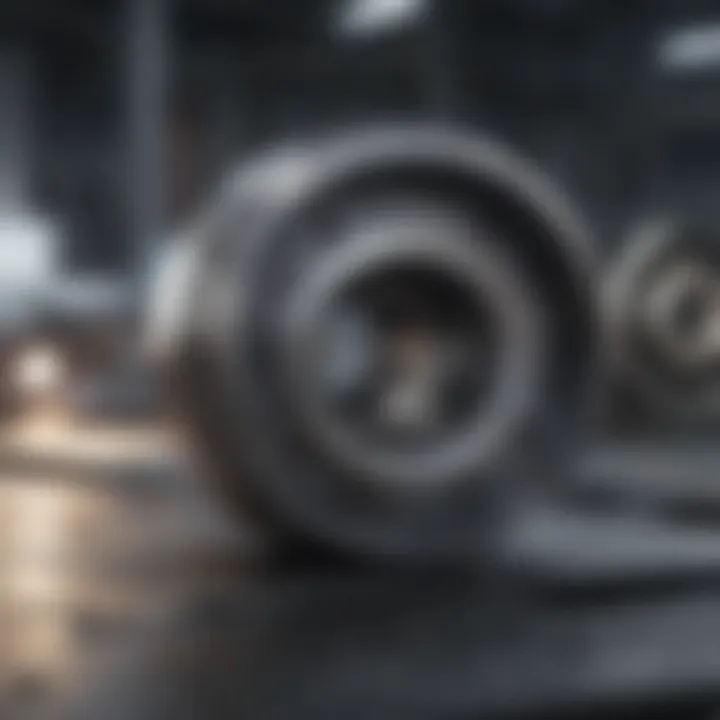
Phase Distribution
Another essential factor in microstructural analysis is phase distribution. A varied microstructure, displaying multiple phases, can lead to diverse magnetic properties, allowing for tailored material behavior. The key characteristic of phase separation is that it can enhance the overall mechanical strength while maintaining magnetic performance.
The unique feature of phase distribution lies in its ability to fine-tune the specific attributes of magnetic alloys. This can sometimes improve resistance to demagnetization during operation. However, managing phase balance can be tricky; too much variation might lead to unpredictable behavior in magnetic applications, thus requiring careful consideration during the alloy development process.
"The microstructure of a magnetic alloy is like its fingerprint; unique to the processes it undergoes and critical to its performance."
Manufacturing Processes of Magnetic Alloys
The manufacturing processes of magnetic alloys play a critical role in determining their overall performance and functionality. How these alloys are produced can significantly influence their magnetic properties and usability across various applications. By understanding the intricacies of these manufacturing techniques, researchers and engineers can optimize the characteristics of magnetic alloys to meet specific needs in industries such as electronics, automotive, and medical devices.
Melting and Casting Techniques
Melting and casting techniques are foundational processes in creating magnetic alloys. The key aspect here is the melting point of the metals involved; for example, iron, cobalt, and nickel, known for their magnetic properties, must be heated to high temperatures to allow for proper mixing and formation of desired phases.
In a typical melting process, the raw materials are heated in a furnace until they reach a molten state. It is essential to control the temperature within specific limits to prevent unwanted reactions that may degrade the alloy's magnetic properties. For instance, if iron is overheated, it can lead to oxidation, which detrimentally affects its magnetic performance.
Once the alloy is melted, it is poured into molds to shape it into desired forms. This casting can be done in several ways, including:
- Sand Casting: Utilizes a sand mold; it's often used for simple shapes and larger parts.
- Die Casting: Involves high-pressure pouring of molten alloy into reusable steel molds, suitable for mass production with consistent, precise shapes.
- Investment Casting: Provides higher accuracy and smooth finishes, often used for components requiring tight tolerances.
Each of these methods has its own set of advantages and challenges. Properly executed casting can result in improved microstructures, ensuring that the produced alloys retain their magnetic properties even under varying operational conditions.
Powder Metallurgy
Powder metallurgy is another manufacturing technique gaining traction in the creation of magnetic alloys, especially when fine control over composition and microstructure is desired. This method starts with metals being ground into fine powders, often mixed with additives to enhance properties such as strength and corrosion resistance.
The key steps in powder metallurgy include:
- Blending: Metals are mixed in very precise proportions to achieve a homogeneous mixture that exhibits consistent magnetic properties.
- Compaction: The powdered metal is then compressed into a desired shape under high pressure. This phase ensures that the particles bond together effectively, a crucial step for achieving the required density and strength.
- Sintering: Following compaction, the shaped powder is heated just below its melting temperature. This process allows the particles to bond together further, refining the microstructure and enhancing magnetic performance.
A notable advantage of powder metallurgy is that it can produce near-net shape components, minimizing waste and allowing for the creation of complex geometries that might be difficult or impossible to achieve with traditional melting and casting methods. Furthermore, sintered parts tend to have superior mechanical properties and better magnetic performance due to their refined microstructures.
"By leveraging advanced powder metallurgy, manufacturers can achieve unique magnetic properties tailored to specific application requirements, paving the way for future innovations in this field."
In summary, the manufacturing processes of magnetic alloys are essential for defining their properties and applications. With techniques like melting and casting and powder metallurgy, the evolution of these processes holds the key to advancing magnetic materials in various high-tech fields. Understanding these processes further enables the development of innovative magnetic alloys, crucial for meeting contemporary challenges in technology.
Applications of Magnetic Alloys
The application of magnetic alloys spans a wide array of industries, showcasing their versatility and pivotal role in modern technology. Understanding the specific applications of these materials illuminates not only their functional importance but also their contribution to efficiency and innovation in various fields. Each application harnesses the unique properties of magnetic alloys, tailoring them to meet specific needs while addressing challenges inherent to the domains they operate within.
Electronics and Telecommunications
In the electronics sector, magnetic alloys are indispensable. They are widely used in magnetic cores of transformers, inductors, and various electronic components to facilitate efficient energy transfer and minimize losses. For instance, iron-nickel alloys, like permalloy, are pivotal in the manufacture of low-loss transformer cores.
Moreover, with the advancement of wireless technologies, components like inductors in RF applications require materials that exhibit stability under varying frequencies. Magnetic alloys support this requirement by providing low coercivity and high permeability, ensuring that signals remain strong and clear without degrading.
- Key Benefits:
- Enhanced energy efficiency.
- Reliability in high-frequency applications.
The telecommunications industry also benefits significantly from the use of magnetic alloys in devices such as antennas and connectors. The careful selection of alloys can lead to improved signal strengths and transmission efficiencies, ultimately enhancing the quality of communication.
Medical Devices
In the medical field, magnetic alloys are crucial for devices such as MRI machines and other diagnostic tools. The alloy composition must be carefully balanced to ensure that imaging is as clear and accurate as possible. Alloys like gadolinium-based compounds are particularly valued for their properties that enhance magnetic resonance imaging capabilities.
Furthermore, magnetic alloys can be found in implantable devices. The demands are steep, often requiring materials to be non-magnetic while maintaining biocompatibility. Innovations in alloy designs aim to develop materials that not only meet these criteria but also improve patient safety and comfort during procedures.
- Considerations:
- Biocompatibility and patient safety.
- Tailored alloy properties to suit specific medical applications.
Automotive and Aerospace Industries
The automotive and aerospace sectors leverage magnetic alloys for applications that range from electric motors in hybrid and electric vehicles to the complex structures found in aircraft.
Magnetic alloys contribute to motor efficiency and performance. For instance, the use of silicon steel in electric motors optimizes energy usage, supporting the growing trend of eco-friendly transportation.
In aerospace, weight reduction is key, and the specific strength of certain alloys makes them ideal for parts that must withstand extreme conditions while remaining lightweight. Notably, developments in high-temperature superconductors present opportunities for even greater efficiency in these applications.
- Benefits of Magnetic Alloys in Automotive and Aerospace:
- Improved performance in electric and hybrid technologies.
- Weight reduction in critical components, supporting fuel efficiency.
"The evolution of magnetic alloys is a testament to human ingenuity, merging science and engineering to create solutions for the challenges faced by diverse industries."
In summary, the applications of magnetic alloys are characterized by their specific contributions to performance, safety, and efficiency across sectors. From the communication systems that connect us to the medical instruments that diagnose and treat, magnetic alloys remain at the forefront of technological advancements, continually evolving to meet increasingly complex demands.
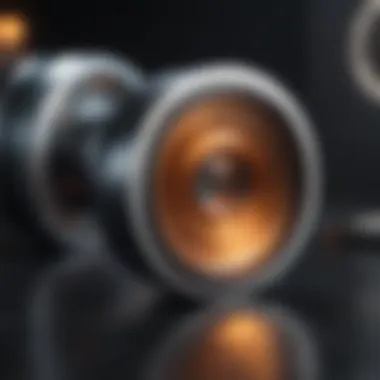
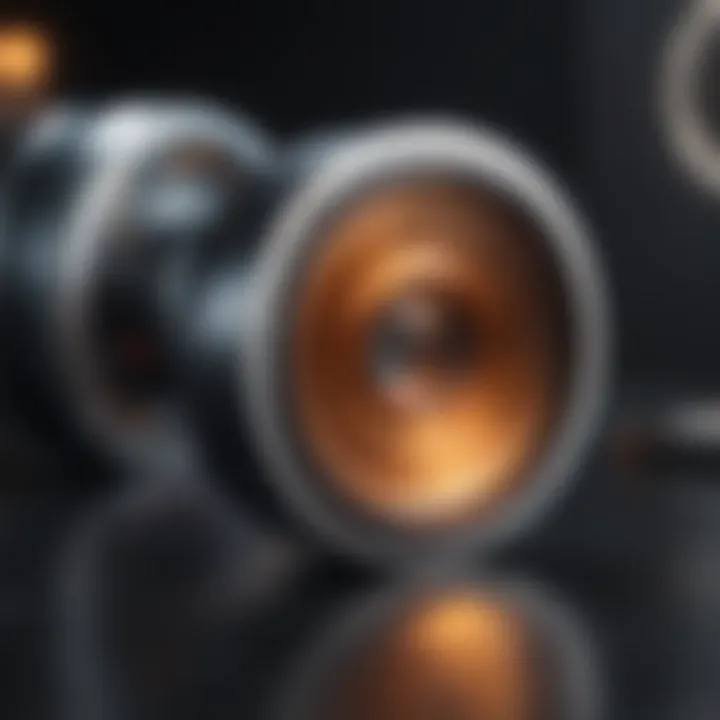
Recent Innovations in Magnetic Alloys
The landscape of magnetic alloys is constantly evolving, driven by both scientific advancement and industry application. Innovations in this field not only enhance the performance of existing materials but also open doors to new technologies that leverage the unique characteristics of these alloys. Understanding recent innovations helps us appreciate the ongoing research efforts, allowing for better utilization across diverse sectors like electronics, medicine, and aerospace.
> "Recent innovations in magnetic alloys are reshaping industries by offering new options that can significantly improve efficiency and functionality."
Advanced Alloy Design Techniques
In recent years, researchers have made remarkable strides in the design of magnetic alloys, aimed at achieving specific magnetic properties tailored to particular applications. For instance, the advent of computational models has transformed how we approach material design. Techniques such as first-principles calculations and machine learning allow scientists to simulate and predict the behavior of alloys before they even reach the lab. This not only saves precious time but also minimizes costly trial and error.
Additionally, advanced techniques like additive manufacturing have come into play. This method allows for the creation of complex geometries that were previously unachievable with traditional manufacturing processes. The flexibility in design can lead to magnetic alloys that are customized for precise applications, such as miniaturized electronic components or bespoke medical devices that enhance patient care.
Moreover, researchers are exploring the benefits of introducing non-standard elements into the alloy mix. By adding elements like rare earth metals, certain properties such as temperature stability and saturation magnetization can be significantly enhanced. For example, alloys that incorporate terbium or dysprosium have shown promising improvements in high-performance magnets, providing a competitive edge in various technological applications.
Nanostructured Magnetic Alloys
The exploration of nanostructured magnetic alloys has opened new avenues for enhancing magnetic performance and achieving unique properties that bulk materials cannot match. At the nanoscale, the interplay between magnetic domains and their environment becomes complex, leading to significantly greater magnetic coercivity and stability. These characteristics are particularly beneficial in applications where precision and reliability are paramount.
Researchers have made leaps in the synthesis of metallic nanoparticles, utilizing methods like sol-gel processes and chemical vapor deposition. This enables fine control of size and shape, subsequently affecting the magnetic properties of the overall alloy. For instance, iron oxide nanoparticles have gained attention in the biomedical field for their potential use in targeted drug delivery and magnetic resonance imaging.
Another exciting area of inquiry involves the development of multilayered or composite magnetic structures where layers of different alloys are strategically combined. This layering can induce unique magnetic behaviors such as exchange bias and giant magnetoresistance, which are desirable in data storage applications.
As we look towards the future, the intersection of nanotechnology and magnetic alloys holds great promise. With continued investment in research and development, it is likely that we will see even more groundbreaking applications and enhancements across myriad fields.
Challenges in Magnetic Alloy Research
The field of magnetic alloy research is intricate, presenting a myriad of challenges that researchers must navigate. While these materials hold immense potential in various technological domains, understanding and overcoming these hurdles is crucial for maximizing their utility. Factors like performance limitations and sustainability concerns are front and center in ongoing studies and developments.
Performance Limitations
Magnetic alloys are not a one-size-fits-all solution. Their performance can be highly variable, influenced by composition, microstructure, and processing methods. For instance, the magnetic properties may degrade under certain conditions. High temperatures or extreme magnetic fields can lead to irreversible changes in magnetization. Moreover, there is often a delicate balance between desired properties like corrosion resistance and magnetic performance. For example, while adding elements such as aluminum can improve strength, it may come at the expense of some magnetic efficiency.
Researchers also grapple with the anisotropic behavior of magnetic alloys, where the magnetic properties differ depending on the direction of measurement. This complexity can hinder their ability to be utilized in applications where uniform magnetic properties are critical. Addressing these limitations requires innovative alloy design and precise manufacturing techniques to enhance properties.
Overall, improving the magnetic performance under various operational scenarios remains a significant focus in the field.
Environmental and Sustainability Issues
As the world pivots towards sustainable practices, the impact of magnetic alloy production on the environment cannot be ignored. The extraction and processing of metals for these alloys often lead to considerable energy consumption and emissions of hazardous pollutants. The mining practices for elements like nickel and cobalt raise questions about sustainability and ethical sourcing, leading to potential backlash from eco-conscious consumers and industries alike.
Moreover, the lifecycle management of magnetic alloys must be considered. Many alloys do not have clear recycling pathways, resulting in waste. Research into recyclable materials or alternatives that provide similar magnetic properties is gradually gaining traction. This focus on finding sustainable solutions is not just a checkbox; it represents a critical evolution in how industries are looking at materials. Integrating eco-friendly practices into the research pipeline is necessary for future developments in magnetic alloys.
"In the quest for advanced magnetic materials, we must not forget the impact of our methods on the planet."
Future Directions in Magnetic Alloy Research
The realm of magnetic alloys is evolving at a rapid pace, with advancements emerging that could redefine their applications and functionalities. As industries today actively pursue alternatives that enhance efficiency, sustainability, and performance, the significance of focusing on future directions in magnetic alloy research cannot be overstated. The evolution in this field is driven by the need to address current performance limitations while considering environmental concerns.
Emerging Trends
Recent years have showcased some remarkable trends in magnetic alloy research. One prominent direction is the development of eco-friendly materials. Traditional manufacturing processes can often be resource-intensive and environmentally damaging, leading to a demand for more sustainable practices. Researchers are now investigating ways to utilize recycled materials in the creation of new magnetic alloys. This practice not only reduces waste but also lessens the carbon footprint associated with ore extraction and processing.
Furthermore, researchers are diving deep into the area of high-temperature superconductors, which offer promising potential for energy-efficient applications. These superconductors can be paired with magnetic alloys, forming new hybrid systems that help overcome energy loss in electrical systems, thus paving the way for more efficient devices in areas like power distribution.
> "The synthesis and analysis of magnetic alloys today direct us not merely to innovate but to integrate sustainability within our technological fabric."
Interdisciplinary Approaches
Magnetic alloy research is beginning to adopt an interdisciplinary approach, melding insights from materials science, physics, and even engineering disciplines. By tapping into diverse fields, researchers can develop innovative solutions that encompass various perspectives, yielding alloys that are smarter and more adaptable.
For instance, collaboration with computational materials science has led to predictive models that can simulate the microstructural behavior of magnetic alloys before they are even synthesized. This not only accelerates the research and development phase but also minimizes costly experimental failures.
The exploration of biomimetic materials is another fascinating interdisciplinary overlap. Here, researchers draw inspiration from nature to craft alloys with enhanced properties—be it improved strength, flexibility, or magnetic performance. One example is the study of how certain biological systems manipulate magnetic fields, leading to potential new methodologies for alloy design.
The future of magnetic alloy research thus appears to be a vibrant tapestry woven from many threads—each one contributing to a deeper understanding and better material performance. The direction set by these trends and approaches will likely fuel innovations for decades to come.
Closure
The exploration of magnetic alloys in this article highlights their significance in modern technology and industrial applications. By dissecting various facets—from their inherent properties to manufacturing processes and their multitude of uses—we gain valuable insights into how these materials shape our world.
Summary of Key Insights
Magnetic alloys are not just a niche in metallurgy; they hold the key to advancements in numerous fields like electronics, medical devices, and aerospace sectors. Here are some pivotal points:
- Diverse Properties: Each type of magnetic alloy, whether ferromagnetic or paramagnetic, exhibits unique characteristics that make them suitable for different applications. These properties are determined by the alloying elements and their microstructural arrangement.
- Manufacturing Techniques: Processes like powder metallurgy and advanced casting methods dictate the performance of the final product. Innovations in fabrication techniques can lead to enhancements in properties like strength, flexibility, and resistance to external factors.
- Growing Applications: The demand for magnetic alloys spans various industries, from smartphones to MRI machines. This is tied to the evolving technological landscape where efficient magnetic materials play a crucial role.
"The future of magnetic alloys is not just about improving existing materials, but rather about pioneering new solutions to meet the challenges of tomorrow's technology."
Final Thoughts on Magnetic Alloys
Looking ahead, the future of magnetic alloys appears promising yet demanding. With rapid advancements in research and development, the focus is likely to shift toward sustainable practices and interdisciplinary approaches. This is essential, given the pressing environmental concerns and the need for efficient resource management in alloy production.
Moreover, the synergy between disciplines, such as materials science, physics, and engineering, could revolutionize the design of magnetic alloys. Innovation will not only enhance the performance and applications of these materials but also ensure that they are environmentally friendly.
As we move forward, continued research into the nuances of magnetic properties, and adaptive manufacturing techniques will be vital in harnessing the full potential of magnetic alloys. Ultimately, their importance is underscored by the advancements they can facilitate in technology, ultimately improving our daily lives.