Exploring Pyridine: Molecular Structure and Significance
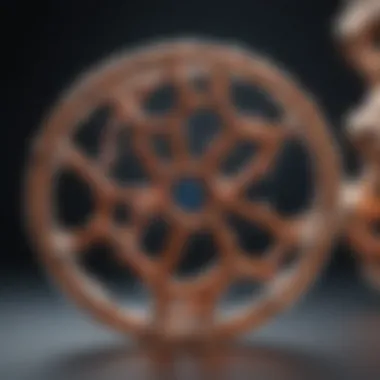
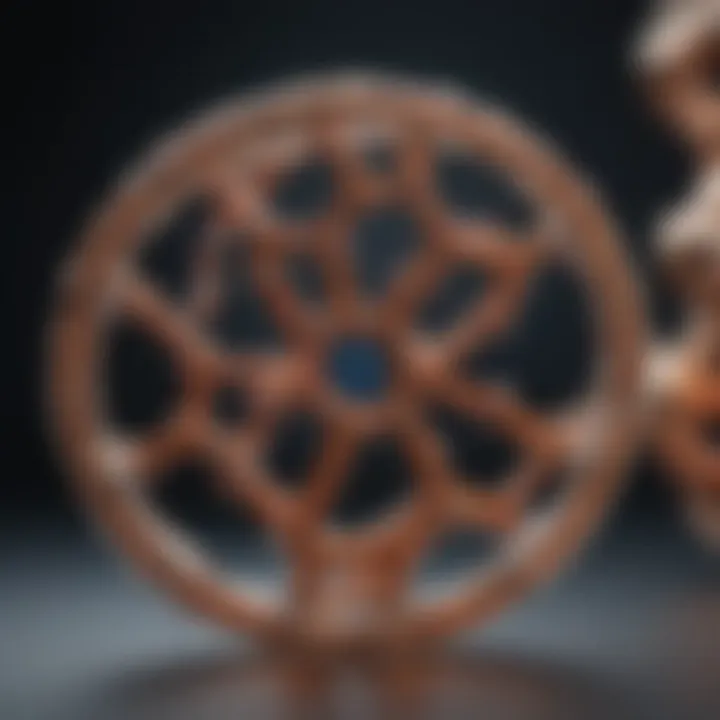
Intro
Pyridine, chemically represented as C5N, is an aromatic heterocyclic organic compound. This simple nitrogen-containing ring has implications across various scientific disciplines. Understanding its molecular structure is essential for grasping its chemical behavior and relevance in industrial applications and biological systems.
Research Overview
Methodological Approaches
In examining pyridine, a mix of theoretical and experimental methods is employed. Researchers often utilize spectroscopy, chromatography, and computational chemistry to analyze its properties. Spectroscopic techniques like NMR (Nuclear Magnetic Resonance) and IR (Infrared Spectroscopy) enable scientists to gain insight into the molecular structure and dynamics of pyridine.
Additionally, computational methods such as density functional theory (DFT) allow for an in-depth analysis of electronic structures. This approach helps in understanding the energy levels and reactivity of pyridine molecules.
Significance and Implications
The significance of C5N extends beyond simple chemical curiosity. Pyridine is a key solvent and reagent in numerous chemical reactions. Its role as a precursor in the synthesis of various organic compounds demonstrates its versatility. In pharmaceuticals, pyridine derivatives are crucial for developing new drugs, highlighting its importance in the medicinal chemistry field.
Pyridine’s toxicological profile also warrants attention. While it serves many beneficial functions, its effects on human health and the environment necessitate cautious handling and regulation. This complex nature punctuates the need for detailed analysis and understanding of its properties.
Current Trends in Science
Innovative Techniques and Tools
Recent advancements in analytical methods are reshaping how pyridine is studied. The introduction of high-resolution mass spectrometry is enhancing the ability to detect and quantify pyridine and its derivatives in various samples. This technology provides greater sensitivity and specificity, enabling researchers to explore trace amounts in complex matrices.
Interdisciplinary Connections
Pyridine’s relevance is not confined to chemistry alone. Its applications touch various fields such as materials science, biology, and environmental science. For instance, in green chemistry, pyridine is studied for its potential to aid in sustainable practices. Its properties are connected to the development of eco-friendly solvents and catalytic processes that minimize waste and hazardous byproducts.
Pyridine serves as a bridge between different scientific disciplines, illustrating the interconnectedness of research and application in modern science.
In summary, the exploration of C5N encompasses a profound understanding of its molecular structure, chemical characteristics, and multifaceted applications. Awareness of its toxicity and regulatory aspects further rounds out its profile in both research and industry.
Preface to C5N
Understanding C5N, commonly known as pyridine, is essential for various fields of chemistry, biology, and industry. This compound, with a simple molecular formula, holds intricate characteristics that play a crucial role in many chemical processes. Its structure contributes to its unique properties and applications, which resonate across different scientific domains.
Definition and Overview
C5N is an organic compound classified as an aromatic heterocycle due to the nitrogen atom present in its ring structure. The presence of nitrogen within the six-membered ring of carbon gives pyridine distinct reactions and interactions compared to other hydrocarbons. The molecular formula indicates that it consists of five carbon atoms, five hydrogen atoms, and one nitrogen atom. This structure allows pyridine to engage in a variety of chemical reactions. The molecule exhibits basic properties, making it a suitable ligand in coordination chemistry and a building block in synthesizing numerous derivatives. Its molecular geometry is planar, which is typical for aromatic compounds, thus affecting its reactivity and interactions.
Historical Context
Pyridine was first discovered in 1849 by the chemist August Wilhelm von Hofmann. He isolated it from coal tar, which is a byproduct of the carbonization of coal. Its name derives from the Latin word "pyr", meaning fire, reflecting its strong association with combustion processes. The compound started garnering attention due to its potential applications in various fields, including agriculture and pharmaceuticals. Over the decades, pyridine has been utilized to synthesize compounds such as herbicides, insecticides, and antiseptics. Its enduring relevance in both academic research and industrial applications positions it as a significant subject in the study of organic chemistry.
Chemical Structure of C5N
The chemical structure of C5N, or pyridine, is central to understanding its properties, reactivity, and applications across various fields. Pyridine is an aromatic heterocyclic compound characterized by its distinct molecular framework. Knowledge of its structure is vital for students, researchers, and professionals involved in chemistry and related disciplines.
Molecular Geometry
Pyridine has a molecular geometry shaped by a six-membered ring containing five carbon atoms and one nitrogen atom. The nitrogen atom's presence distinguishes pyridine from other aromatic compounds. In pyridine, the arrangement of atoms leads to a planar structure, with bond angles close to 120 degrees. This geometry is crucial for its stability and reactivity.
The structure can be visualized as:
In addition to its planar conformation, the lone pair of electrons on the nitrogen does not participate in the aromatic resonance, so it remains in an sp2 hybridized orbital. This arrangement affects how pyridine interacts with other molecules. The presence of nitrogen contributes to the compound's polarity and solubility in polar solvents.
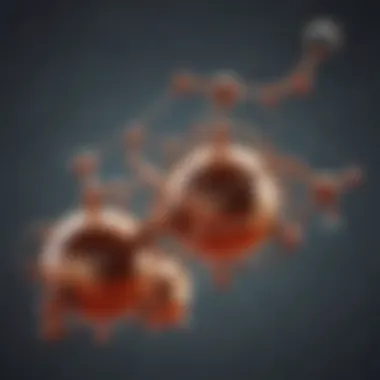
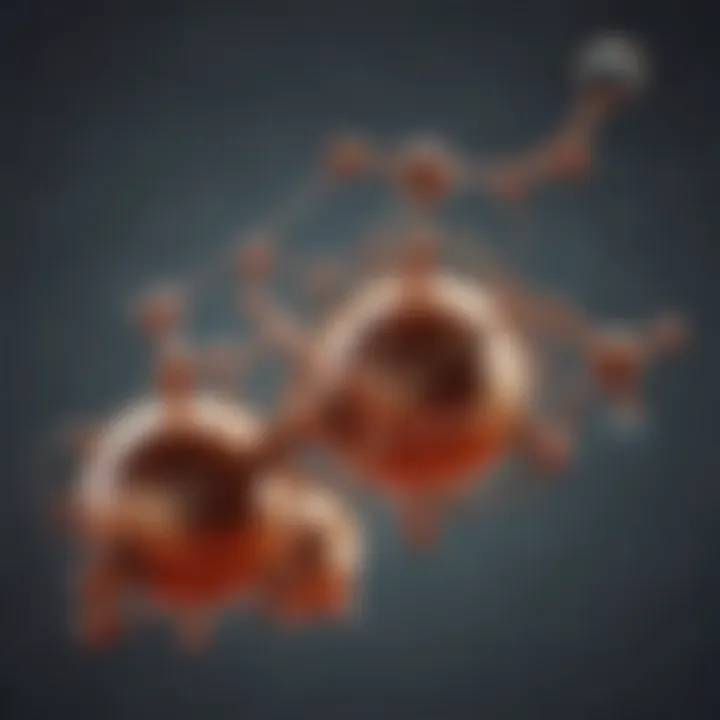
Bonding Characteristics
Pyridine's bonding characteristics are defined by its hybridization and the nature of the bonds present in its structure. The carbon atoms form sigma bonds through sp2 hybridization, allowing for π-bonding that contributes to the compound's aromaticity. The resonance within the ring stabilizes its structure. Furthermore, the nitrogen participates in the formation of both sigma bonds with carbon and maintains a lone pair of electrons.
These aspects lead to several key features:
- Electronegativity: Nitrogen's higher electronegativity compared to carbon creates a dipole moment in the molecular structure. This impacts pyridine's interactions with other chemical species.
- Reactivity: Due to the lone pair on nitrogen, pyridine can act as a weak base. Its bonding structure allows for protonation reactions, making it reactive in acidic environments.
- Differentiation in nucleophilicity: The basic nature of pyridine is lower than that of aliphatic amines due to the aromatic stabilization from the pi system, a pertinent factor in many chemical reactions.
Understanding these bonding characteristics is crucial for interpreting pyridine's performance in various chemical reactions and its behavior in biological systems. In summary, the molecular structure of C5N provides invaluable insight into its chemical behavior and potential applications.
Physical Properties of Pyridine
Understanding the physical properties of C5N, also known as pyridine, is crucial for its applications in various fields. These properties not only define its behavior in chemical reactions but also determine its usability across industries such as pharmaceuticals, agriculture, and materials science. Physical characteristics like melting point, boiling point, solubility, and density provide insights into how pyridine can be effectively handled and utilized.
Melting and Boiling Points
Pyridine has a melting point of about -42 °C and a boiling point near 115 °C. These values indicate that pyridine is a liquid at room temperature, making it easy to incorporate into many chemical processes. The relatively low melting point suggests that it remains in liquid form under standard environmental conditions. The boiling point, however, is significant for processes that require heating, such as distillation and reactions conducted under reflux.
The boiling point also plays a role in determining the safety measures needed when working with pyridine, as it indicates the temperature at which it can vaporize to form potentially hazardous fumes.
"Pyridine's boiling point necessitates careful handling and proper safety protocols during laboratory or industrial use."
Solubility and Density
Pyridine is soluble in water, ethanol, and ether, which is an essential characteristic for its use in chemical synthesis and reactions. Its solubility in water facilitates various applications, including its role as a solvent and a precursor in synthetic chemistry. The density of pyridine is about 0.981 g/cm³ at 20 °C, slightly lighter than water. This aspect is also important when considering the separation processes or interactions with other substances in mixtures.
Pyridine's ability to dissolve a range of organic compounds allows it to act as an effective solvent in various laboratory settings. Its versatility enhances its role in reaction mediums, making it a valuable compound in chemical manufacturing.
Synthesis of Pyridine
The synthesis of pyridine is a vital area of study within chemistry due to its significant role in various industrial applications and biological functions. Understanding how pyridine can be synthesized effectively provides valuable insights into its utility and potential for innovation. There are two primary routes for synthesizing pyridine: natural occurrence and synthetic methods. Each method presents unique benefits, considerations, and implications for different fields of research and application.
Natural Occurrence
Pyridine naturally occurs in small amounts in several plants, particularly in the form of alkaloids. These compounds often play a critical role in plant defense mechanisms and contribute to their complex biochemistry. This organic molecule can also be found in certain animal tissues and products, such as fermented foods and cigarette smoke. The ability to extract pyridine from natural sources presents opportunities for sustainable practices in production but also has limitations, like low yield and variability in purity. Therefore, while the natural path to obtaining pyridine is fascinating, it is not typically the primary focus for large-scale synthesis.
Synthetic Methods
Chemical Reactions
Chemical reactions for synthesizing pyridine are crucial due to their cost-effectiveness and scalability. Among the most popular methodologies are the Hantzsch pyridine synthesis and the Bönnemann reaction. These reactions allow for the formation of pyridine from simpler, readily available chemical precursors. The key characteristic of these reactions is their ability to generate pyridine derivatives with varying functional groups, thus enhancing the compound's range of applications in pharmaceuticals and agrochemicals. The benefit of chemical reactions lies in their ability to produce large quantities efficiently. However, navigating the complex mechanisms and necessary conditions for reaction can be a challenge, requiring careful attention to optimize yields and purity.
The unique feature of these reactions is the potential for specific functionalization, making them attractive for tailored applications. The advantage of utilizing chemical reactions for pyridine synthesis is direct control over the synthesis parameters; however, improper handling or conditions can lead to undesired by-products, complicating purification processes.
Catalytic Processes
Catalytic processes also offer a significant path for pyridine synthesis. They involve the use of catalysts to accelerate reactions, typically leading to higher yields and more efficient energy use. Notable approaches include the use of transition metals to promote cyclization or dehydrogenation of precursors to form pyridine. These key characteristics make catalytic processes a popular choice in both research and industry, providing benefits like reduced reaction times and lower energy requirements compared to traditional methods.
The unique feature of catalytic processes is their ability to operate under milder conditions, which can minimize the risk of thermal degradation of sensitive compounds. While the advantages include efficiency and selectivity, concerns about catalyst recycling and contamination can limit their applicability in some scenarios. Thus, exploring both synthetic methods further enriches the body of knowledge surrounding pyridine and its synthesis, leading to potential breakthroughs in its applications.
Reactivity and Chemical Reactions
The field of reactivity and chemical reactions is essential to understanding pyridine (C5N) and its behaviors in various environments. Pyridine is known for its aromaticity, which influences its reactivity patterns significantly. This section highlights the beneficial aspects and considerations surrounding the reactivity of pyridine, emphasizing its role in both organic synthesis and practical applications.
Electrophilic Aromatic Substitution
Electrophilic aromatic substitution (EAS) is a fundamental reaction in organic chemistry that occurs with pyridine. The nitrogen atom in pyridine introduces unique properties due to its lone pair of electrons. Though pyridine is less reactive than benzene in EAS due to the electron-withdrawing effect of nitrogen, it can still participate in this type of reaction.
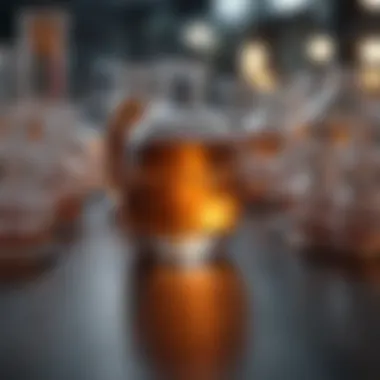
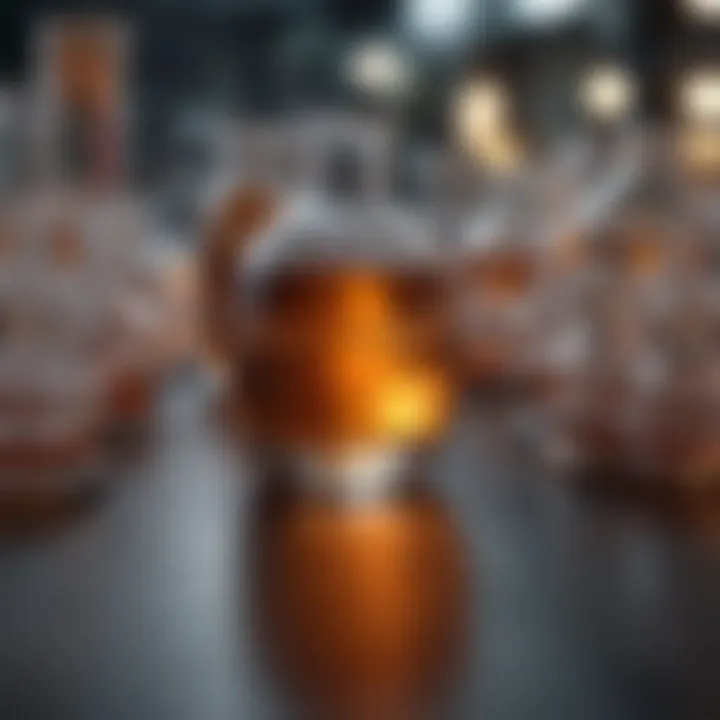
In EAS, an electrophile replaces a hydrogen atom in the aromatic ring, allowing for the formation of diverse products. For pyridine, the position of substitution tends to favor the 2- and 4- positions due to the resonance stabilization offered by the nitrogen lone pair, which is less available at the 3-position.
The common electrophiles used in EAS with pyridine include bromine and nitrating agents. The results often expand the utility of pyridine in creating complex chemical structures that serve as intermediates in various industrial applications. For example, the bromination of pyridine can yield valuable compounds used in agricultural chemicals and pharmaceuticals.
Nucleophilic Reactions
Nucleophilic reactions also play a significant role in the chemistry of pyridine. The basicity of pyridine allows it to act as a nucleophile in many reactions, where it can donate its lone pair of electrons to electrophilic groups.
In nucleophilic reactions, pyridine can react with halogens or carbonyl compounds. A notable reaction is the nucleophilic acyl substitution where pyridine acts as a base and nucleophile. This reaction is particularly significant in synthesizing pyridine derivatives that possess desirable biological activities.
Furthermore, the addition of nucleophiles can lead to the formation of pyridine N-oxides and other important derivatives that are critical in pharmaceutical development. The versatility of pyridine in nucleophilic reactions not only showcases its importance in synthesis but also its role in drug discovery and development.
In summary, both electrophilic aromatic substitution and nucleophilic reactions underscore the rich chemistry of pyridine. The ability to engage in these reactions broadens the scope of their applications in fields such as materials science, medicinal chemistry, and agricultural innovation.
"Pyridine's reactivity in electrophilic and nucleophilic reactions is a cornerstone of its application in the field of chemistry, driving advancements across several industries."
This understanding of reactivity sets the stage for future endeavors in pyridine research, paving the way for innovative applications and new synthetic strategies.
Applications of Pyridine
Pyridine, with the chemical formula C5N, serves as a versatile compound in various industries and fields. Its unique molecular structure allows it to participate in multiple chemical reactions, making it valuable in both industrial applications and pharmaceuticals. Understanding these applications is crucial, as it highlights pyridine's significance in modern science and technology.
Industrial Uses
In the realm of industry, pyridine is a key ingredient in the manufacturing of numerous products. One of its primary uses is as a solvent. It dissolves a wide range of compounds, making it essential in the production process of dyes and pesticides. Additionally, due to its ability to withstand high temperatures, pyridine plays a role in the synthesis of various polymers and resins.
- Production of Agrochemicals: Pyridine derivatives are used in the formulation of herbicides and insecticides, improving agricultural outcomes.
- Synthesis of Pharmaceuticals: It acts as a building block for numerous medications, enhancing their efficacy and stability.
- Manufacturing of Rubber: Pyridine contributes to the vulcanization process, improving the resilience of rubber products.
Pyridine also finds its application in electrochemistry. It serves as a ligand in coordination chemistry and aids in the design of electrochemical sensors. Its role in these industrial contexts cannot be understated, as it helps drive innovations and improvements in product formulation.
Role in Pharmaceuticals
Pharmaceutically, pyridine is more than just a chemical structrure; it is a crucial component in many life-saving drugs. Its properties enable it to interact favorably within biochemical pathways, offering therapeutic benefits. Pyridine derivatives have established their presence in various therapeutic areas, ranging from anti-inflammatory drugs to antibiotics.
- Synthesis of Nicotinic Acid: Pyridine is a precursor in the formation of nicotinic acid, which is vital in treating conditions such as hyperlipidemia and cardiovascular diseases.
- Antibiotics and Anti-Cancer Agents: Compounds derived from pyridine have demonstrated anti-bacterial and anti-cancer properties, proving their importance in medical treatments.
- Centrally Active Compounds: Some pyridine derivatives function as anesthetics or sedatives, affecting neurotransmitter systems within the body.
This dual role in both industrial applications and pharmaceuticals showcases pyridine's versatility. Its impact on research, development, and practical applications highlights its importance in chemical science, influencing both economic growth and advancements in health technology.
"Pyridine is a bridge between chemistry and applied sciences, facilitating progress in both drug development and industry."
Biological Implications of Pyridine
The significance of pyridine in biological contexts cannot be understated. Pyridine itself acts as a pivotal organic compound that influences diverse biochemical pathways and physiological processes. Being a nitrogen-containing heterocyclic compound, it features prominently in various biochemical applications, highlighting its importance not only as a molecular structure but also in its functional significance within living organisms.
Pyridine in Biochemistry
Pyridine serves as a fundamental building block in biochemistry. Its derivatives play crucial roles in numerous biological activities. For example, pyridine rings are integral to the structure of essential coenzymes such as NAD (Nicotinamide Adenine Dinucleotide) and NADP (Nicotinamide Adenine Dinucleotide Phosphate). These coenzymes are vital for cellular respiration and metabolism, facilitating redox reactions that are necessary for energy production.
Pyridine derivatives also act as precursors for various alkaloids. These compounds may exhibit significant biological activity, including enzyme inhibition, antioxidant action, and anti-inflammatory effects. Alkaloids derived from pyridine can be found in numerous plants and have been employed in traditional and modern medicine. Their biological activity often stems from the structural features present in the pyridine ring, which enhances molecular interactions with biological targets.
Additionally, studies show that pyridine derivatives are involved in neurotransmission. Compounds like nicotine, a pyridine alkaloid, demonstrate meaningful interactions with nicotinic acetylcholine receptors. This interaction has broader implications for understanding addiction, neurodegenerative diseases, and cognitive function.
Toxicological Aspects
While pyridine and its derivatives have beneficial applications in biology, concerns about their toxicity must be addressed. Pyridine itself is considered toxic upon inhalation or ingestion, causing irritation of the respiratory tract and central nervous system effects. Continuous or high-level exposure can lead to severe health implications.
A deeper understanding of the toxicological effects of pyridine is critical in assessing its usage in pharmaceuticals and other industrial applications. Toxicology studies focus on its metabolites, which may exhibit different toxicity levels compared to the parent compound. For instance, some pyridine derivatives have been shown to cause genotoxic effects in laboratory settings. This necessitates careful monitoring and regulation when pyridine is employed in synthesis and manufacturing processes.

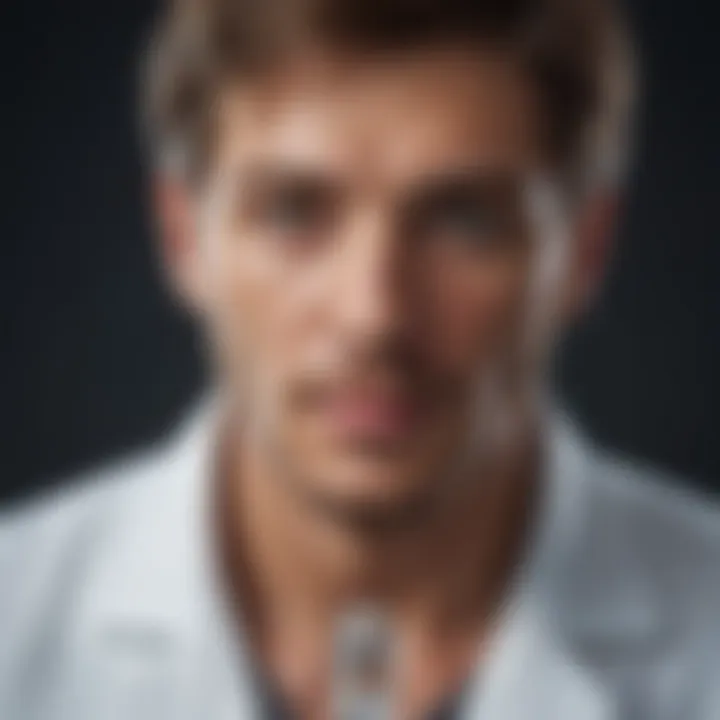
Furthermore, regulatory agencies like the Environmental Protection Agency (EPA) have set guidelines to limit exposure to pyridine in occupational and environmental contexts. It is crucial for professionals endeavoring in fields that utilize pyridine to remain informed about safety standards and toxicity data.
"Understanding the toxicological profile of pyridine and its derivatives allows researchers and industry professionals to harness their benefits while mitigating risks."
In summary, pyridine's role in biochemistry is multifaceted and significant. Its essential functions as a building block in various biochemical pathways, alongside its toxicological considerations, invite ongoing research in both the industrial and healthcare sectors. Balancing its applications with safety assessments remains a crucial endeavor for future developments.
Derivatives of Pyridine
Derivatives of pyridine play a crucial role in various fields, including organic chemistry, pharmaceuticals, and agriculture. They improve the functionality of pyridine, leading to compounds that exhibit unique properties. Understanding these derivatives allows researchers to enhance the usefulness of pyridine in synthesizing new materials and formulations.
Common Derivative Structures
Pyridine has numerous derivatives, each with distinct structures and characteristics. A few of the most notable derivatives include:
- 2,6-Lutidine: A dimethyl derivative of pyridine, which is used in organic synthesis and as a solvent.
- Pyridine N-oxide: An oxidized derivative often applied in the synthesis of various chemicals and in biological studies.
- Nicotine: A well-known alkaloid derived from pyridine, it has significant implications in both agriculture and medicine.
- Isoquinoline: This compound contains a fused ring structure with pyridine, notable in drug development for its diverse biological activity.
Each derivative has its unique properties and applications, making them valuable in different scientific and industrial contexts.
Applications of Derivatives
The applications of pyridine derivatives are extensive and varied. They serve critical roles in:
- Pharmaceutical Industry: Many drugs include pyridine derivatives in their structure. They contribute to the bioactivity of medicines, making them essential in drug design. For instance, nicotine and analgesics often rely on these compounds for their efficacy.
- Agrichemicals: Certain derivatives function as herbicides and insecticides, aiding in crop protection. Examples include pyridine fungicides, which help manage plant diseases.
- Dyes and Pigments: Pyridine derivatives can serve as precursors in dye production, leading to vibrant colors in textiles and other materials.
- Material Science: Due to their unique chemical structure, some derivatives are utilized in creating advanced materials, including polymers and catalysts.
The ability of pyridine derivatives to enhance properties and functions highlights their significance in research and industry.
Environmental Impact of Pyridine
The environmental impact of pyridine, a heterocyclic organic compound, is significant due to its widespread industrial use and potential ecological effects. This section aims to elucidate how pyridine interacts within ecosystems, the benefits of understanding its presence, and the considerations necessary for its management.
Pyridine in Ecosystems
Pyridine can enter ecosystems through various pathways, including agricultural runoff, industrial waste, and atmospheric deposition. Its solubility in water enables it to disperse through soil and water systems, potentially affecting aquatic and terrestrial organisms.
- Influence on Aquatic Life: Pyridine’s toxicity to fish and invertebrates has been documented. High concentrations can disrupt metabolic processes, leading to behavioral changes and decreased reproductive success in affected species. Understanding this impact is essential for maintaining biodiversity.
- Soil Microbial Communities: When pyridine enters the soil, it influences microbial communities. Some bacteria can utilize pyridine as a carbon source, while others may be negatively affected. The balance within these communities determines the overall health of the ecosystem.
- Plant Interactions: Pyridine can affect plant growth as well. Some plants may absorb pyridine, which can alter their physiological functions, including nutrient uptake and stress responses. This chain of impact can extend through the food web, affecting herbivores and their predators.
Regulation and Safety
The regulation of pyridine is critical due to its classification as a toxic substance. Various governmental and regulatory bodies address its use and disposal. Ensuring proper handling and application minimizes risks to human health and the environment.
- Regulatory Framework: In many regions, pyridine is regulated under laws concerning hazardous substances. This includes limits on emissions in industrial processes and guidelines for waste disposal. Agencies such as the Environmental Protection Agency (EPA) set standards that industries must follow to mitigate environmental harm.
- Risk Assessment: Regular assessments help determine the possible risks to ecosystems and human health associated with pyridine exposure. Documenting and evaluating data on its toxicity, persistence in the environment, and bioaccumulation potential remains crucial for informed decision-making.
- Safety Measures: Industries utilizing pyridine must adhere to strict safety protocols. Proper use of personal protective equipment (PPE), containment measures during spills, and appropriate waste disposal practices help minimize risks associated with its handling. Educating workers about the hazards of pyridine is an essential part of maintaining safety.
Understanding the environmental impact of pyridine is vital for sustainable practices in its use and management. Awareness and regulation can significantly mitigate negative effects while preserving ecological balance. By prioritizing research and safety, industries can contribute positively to the environment.
Future Perspectives on Pyridine Research
The exploration of pyridine continues to expand, particularly as new technologies and methodologies emerge. This section presents an examination of future perspectives in pyridine research, focusing on novel applications, advances in synthesis, and the evolving understanding of its biological implications. As researchers delve into the complexities of C5N, there is potential for significant breakthroughs that could impact various fields including pharmaceuticals, agriculture, and materials science.
Emerging Applications
In contemporary research, the function of pyridine is shifting beyond its conventional roles. Here are some emerging applications:
- Nanotechnology: Pyridine derivatives are being explored for their potential in fabricating nanomaterials. Their ability to stabilize nanoparticles could lead to advancements in drug delivery systems.
- Agricultural Chemistry: Research is underway to utilize pyridine-based compounds as effective agrochemicals. Their herbicidal and insecticidal properties may contribute to sustainable farming practices by providing alternatives to existing pesticides.
- Biotechnology: Pyridine's role in biosensors and biocatalysts is gaining attention. The capacity to enhance reactions in biotechnological processes presents opportunities in biofuel production and waste management.
These emerging applications illustrate the versatility of pyridine and its relevance to modern scientific challenges. The continued exploration of these areas could lead not only to innovative products but also to environmentally-friendly alternatives.
Challenges and Opportunities
As the research community looks to uncover new facets of pyridine, several challenges and opportunities come to light:
- Sustainability: One of the most pressing challenges is the need for sustainable synthesis methods. Traditional processes may be environmentally detrimental. Developing greener alternatives is vital for future work.
- Regulatory Hurdles: The introduction of new pyridine derivatives into the market often faces regulatory scrutiny. Ensuring compliance with safety and environmental standards can significantly complicate the research and development process.
- Interdisciplinary Collaboration: There is a growing need for collaboration across disciplines. Combining insights from chemistry, biology, and environmental science can lead to innovations that a single field might not achieve.
Addressing these challenges presents a unique opportunity for researchers. Collaborations can foster a richer understanding of pyridine’s roles and lead to the development of new applications that are both effective and responsible.