Exploring Scanning Tunneling Microscope Images
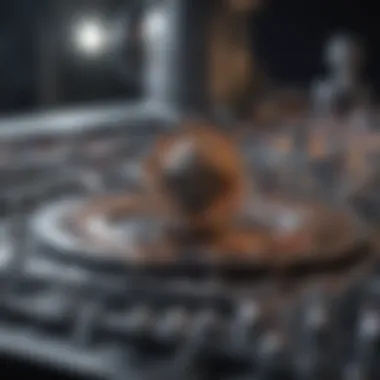
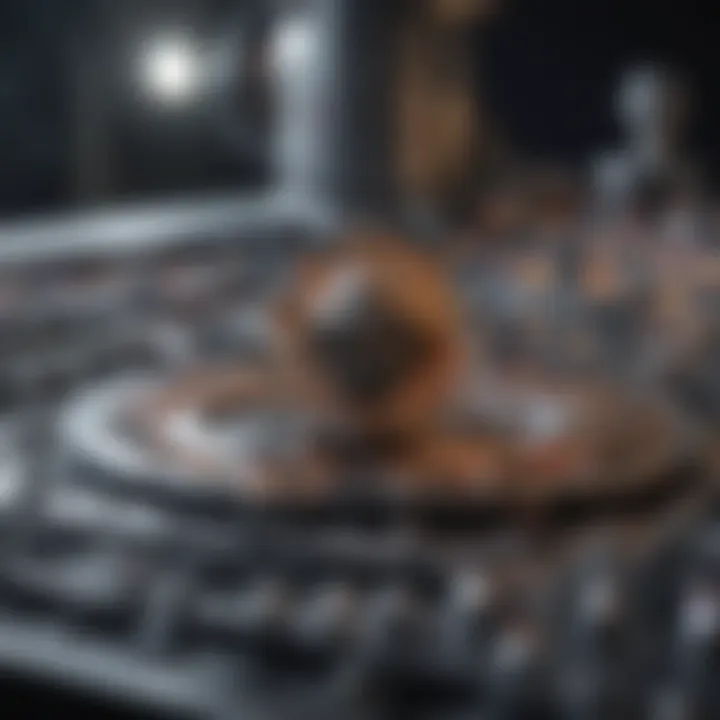
Intro
The exploration of scanning tunneling microscopy (STM) images has gained significant attention in the fields of nanoscience and material characterization. This focus is driven by the ability of STM to visualize atomic structures and properties in materials with remarkable precision. Understanding STM images is crucial for researchers, students, and professionals engaged in advanced scientific investigations.
Scanning tunneling microscopy operates on principles that allow a sharp probe to scan surfaces at the atomic level. As it moves close to a conductive surface, quantum tunneling of electrons occurs, creating an image with intricate detail. The resulting STM images provide insight into the electronic properties and surface structures of materials.
As STM technology advances, so does its relevance in various scientific disciplines. Researchers harness these images to develop new materials, understand their properties, and visualize phenomena that cannot be seen with traditional instrumentation.
Research Overview
Methodological Approaches
The methodological strategies employed in examining STM images are varied and multifaceted. Typically, the image acquisition process relies on maintaining a stable tip-to-sample distance, allowing for high-resolution imaging. Specific techniques may include:
- Constant Current Mode: The tip remains at a stable current, adjusting its height to maintain a constant tunneling current, thus preserving image quality.
- Constant Height Mode: The distance between the tip and surface is kept constant, allowing for faster scans but requires careful sample condition management.
Research also involves processing STM images through algorithms that enhance clarity and detail. Techniques like filtering and averaging help to refine the images and uncover features that may not be immediately visible.
Significance and Implications
STM images are not just tools for visualization; they serve as a foundation for interpreting material properties at the atomic level. The ability to analyze these images contributes to advancements in:
- Material science: Understanding correlations between atomic structure and material properties.
- Nanotechnology: Designing new nanoscale devices with enhanced functionalities.
- Surface chemistry: Investigating chemical reactions at the surface level.
In the broader context, the implications of STM images extend to industries such as electronics, where the miniaturization of components requires a deep understanding of materials on an atomic scale.
Current Trends in Science
Innovative Techniques and Tools
Recent developments in STM technology have led to innovative imaging techniques that enhance the resolution and capabilities of STM.
- Spin-Polarized STM (SP-STM): This technique allows researchers to visualize the electronic spin of electrons, providing new insights into magnetic materials.
- Functionalized Probes: Engineers are creating specialized tips that can interact with specific surface features, improving the precision of imaging.
These advancements not only improve the quality of images but also expand the range of applications for STM across different materials and conditions.
Interdisciplinary Connections
The relevance of STM images stretches across disciplines, bridging chemistry, physics, and engineering. Researchers from various fields collaborate to leverage STM technology for diverse applications such as:
- Developing catalysts for energy conversion.
- Analyzing biomaterials for medical applications.
- Understanding nanoscale phenomena in semiconductors.
This interdisciplinary approach enhances the collective understanding of material properties and pushes the frontiers of research in fundamental and applied sciences.
STM technology continues to evolve, offering new perspectives in the study of materials at the atomic level.
By exploring the intricate details provided by STM images, scientists can contribute to groundbreaking discoveries that will shape future technologies.
Preface to Scanning Tunneling Microscopy
Scanning tunneling microscopy (STM) occupies a pivotal role in the evolving landscape of nanoscience and materials research. It offers a unique ability to visualize and characterize surfaces at the atomic level. Since its inception in the 1980s, STM has transformed scientific understanding of material compositions and structures. Thus, its importance cannot be overstated. In this article, we delve into various aspects of STM, providing a well-rounded comprehension of its principles, implications, and challenges in image interpretation.
The advantages of STM include its high spatial resolution and ability to create topographic maps of conductive surfaces. Researchers can observe atoms and correlate their arrangements with material properties. STM images serve as a crucial foundation for interpretations across disciplines, including physics, chemistry, and material science. However, the technique also presents challenges such as image noise and artifacts that can obscure true representations of surface structures.
Understanding the fundamentals underlying STM technology is essential. It enables readers to appreciate the intricacies and the technological advancements that have brought STM to its current state. Furthermore, exploring the historical context of STM provides insights into how the technology has evolved and the significant milestones achieved over the years.
"STM represents a significant leap in our capability to investigate material properties at atomic levels, contributing to innovations in numerous fields."
In the subsequent sections, we will examine the foundational principles that define STM, trace its development through history, and provide an analytical framework for both image acquisition methods and image interpretation. The rich tapestry of knowledge surrounding STM paints a comprehensive picture, making it an indispensable tool for scientists and engineers alike.
Fundamentals of STM Technology
The essential concept of scanning tunneling microscopy is based on quantum tunneling. This phenomenon occurs when a conductive tip made of a conductive material approaches a conductive surface at a nanometer distance. When the tip is close enough to the surface, electrons can tunnel between the tip and the sample. The conductance measured during this tunneling gives a real-time representation of the surface topography.
The system operates on a feedback loop that maintains a constant tunneling current. Any variations in the current indicate changes in height, allowing the microscope to scan and create detailed images of the surface.
Historical Context and Development
The journey of scanning tunneling microscopy began with the groundbreaking work of Gerd Binnig and Heinrich Rohrer at IBM Zurich in 1981. Their invention won the Nobel Prize in Physics in 1986, highlighting its significance in the field. Initially, the focus was primarily on semiconductor surfaces, which benefited greatly from STM’s capabilities. Over time, STM evolved to explore a broader range of materials, including insulators and biological samples.
As technology progressed, efforts aimed to enhance image resolution and reduce noise in STM imaging became priority. Developing various techniques and strategies, researchers began to integrate STM with other methods, solidifying its status as a cornerstone of nanotechnology. This historical perspective underlines the importance of STM in advancing modern science.
Mechanics of Scanning Tunneling Microscopes

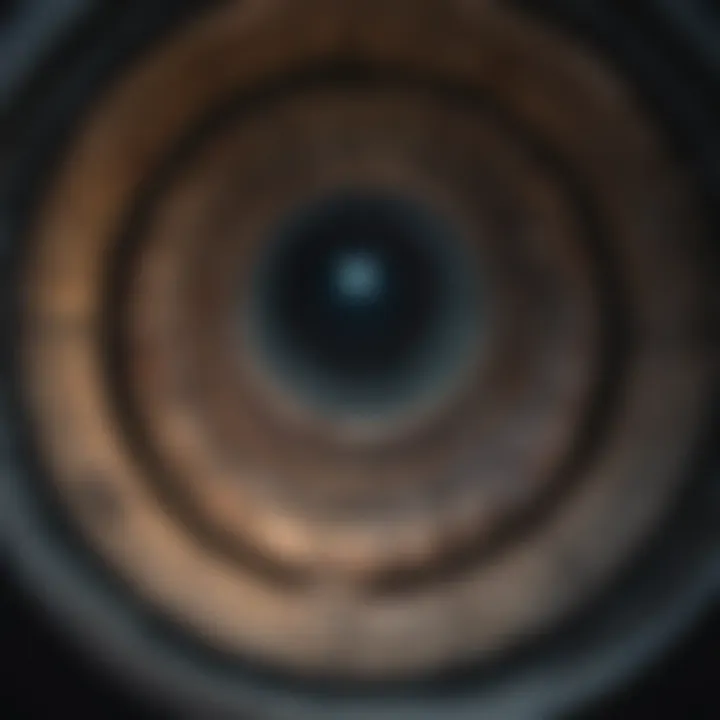
The mechanics of scanning tunneling microscopes (STM) are fundamental to understanding how this instrument operates at the atomic level. STMs enable researchers to visualize surfaces with extraordinary precision and play a crucial role in various fields, such as nanotechnology and material science. By grasping the mechanics of STM, one can appreciate its unique capabilities, such as atomic resolution and the analysis of electronic properties on a nanoscale.
Basic Operating Principles
The basic operating principles of STM are rooted in quantum mechanics. At the heart of its function is the tunneling phenomenon, which occurs when a sharp metallic tip is brought close to a conductive surface without direct contact. The key concepts to understand include:
- Tunneling Current: The current that flows between the tip and the substrate is due to electron tunneling. This current is highly sensitive to the distance between the tip and the surface.
- Feedback Mechanism: As the STM scans the surface, it employs a feedback loop to maintain a constant tunneling current. This adjustment allows the tip to glide along the surface, creating an accurate topographical map.
- Scan Mode and Imaging: STM can operate in different modes, including constant current mode and constant height mode, which influence image quality and resolution.
The STM's ability to translate tunneling current changes into topographic images makes it an invaluable tool in nanoscience. This process highlights the essential nature of distance on atomic interactions.
Components of an STM Setup
A standard STM setup comprises several crucial components that work together to facilitate imaging at the atomic level. Understanding these components is essential for both the effective operation and improvement of STM technology.
- The Tip: Typically made from a metal such as tungsten or platinum-iridium, the tip must be sharp, with a radius on the order of nanometers, to achieve the necessary resolution.
- Sample Stage: This is where the sample is mounted. It can be controlled in three dimensions, allowing for precise positioning with respect to the tunneling tip.
- Scanning Mechanism: This consists of piezoelectric actuators that control the movement of the tip over the surface. Precision here is vital for maintaining the correct distance during imaging.
- Control Electronics: This includes all the circuitry necessary to maintain the feedback loop and to process the tunneling current data into images.
- Computer System: For data acquisition and analysis, a computer aids in interpreting the signals based on the STM's operational modes.
Together, these components provide an integrated system that allows scientists to explore materials at unprecedented resolutions.
"Understanding the mechanics of STM is key to unlocking its full potential in nanoscience."
The combination of these elements streamlines the method of acquiring high-resolution images of surfaces, assisting researchers in advancing their work with various applications in material science and beyond.
Acquisition of STM Images
The acquisition of images using scanning tunneling microscopy (STM) is a fundamental mechanism that underpins the technology’s extensive applications in nanoscience. STM provides images at the atomic scale, enabling researchers to visualize surface structures and electronic properties of materials. Understanding this process is crucial for interpreting the information conveyed by STM images. The significance lies not only in the methodological framework but also in the implications these images have on scientific research and material characterization.
The acquisition process begins with the delicate operation of positioning a sharp conductive tip very close to the sample surface. The tunneling current, which flows between the tip and the conductive surface, is directly related to the distance between them. As the tip scans across the surface, variations in the tunneling current allow for the generation of a topographical image. One key benefit of this process is its ability to provide real-time imaging of surface features with unprecedented resolution.
Beyond just the mechanical operation, the acquisition of STM images encompasses crucial considerations, including environmental isolation, temperature stability, and noise reduction. These factors are pivotal in mitigating disturbances that could adversely affect the quality of the images produced. Furthermore, each element of the scanning process can reveal a wealth of information, identifying material defects or electronic properties that could lead to breakthroughs in research.
"Proper acquisition of STM images not only ensures high fidelity results but also enhances the reliability of subsequent analyses."
Scanning Process Explained
The scanning process in STM is intricate and requires a precise approach to ensure accurate image acquisition. It typically utilizes a feedback loop that adjusts the height of the tip in response to the tunneling current. When the tip is positioned too far from the surface, the tunneling current decreases, prompting the system to lower the tip. Conversely, if the tip is too close, the current increases, and the system reacts accordingly to maintain a constant tunneling current.
This feedback mechanism is vital as it ensures that the tip remains an optimal distance from the surface, generally between 1 to 10 angstroms. The speed and scanning rates can also be adjusted based on the resolution required. The interaction between the tip and surface is not just a physical scan; it is an observation of electronic interactions that depend on various factors like surface condition and material types.
This process allows for the acquisition of various types of images, including topographic and spectroscopic data, based on how the tunneling current is manipulated during the scanning. Understanding the intricacies of the scanning process informs researchers about the properties of the surface being analyzed.
Image Resolution and Quality Factors
Image resolution is a critical characteristic of STM imaging that defines the detail and clarity of the resultant images. Several factors impact the resolution quality, most notably, the sharpness of the tip, which influences the tunneling current's sensitivity. A sharpened tip with only a few atoms in width can greatly enhance image clarity by allowing finer measurements as it interacts with individual atoms on the surface.
Another factor influencing image resolution is the stability of the scanning environment. Any form of vibration or air currents can interfere with the precision required for accurate measurements. Techniques such as using vibration isolation tables and maintaining a suitable vacuum environment can enhance image quality significantly.
In addition to environmental factors, electronic noise plays a significant role. Background noise can lead to artifacts that obscure genuine features in the images. Researchers must strive to minimize this by isolating the system from electronic interference.
In summary, achieving high-resolution images in STM not only requires optimal equipment settings but also a controlled environment. This ensures that the data gathered contributes effectively to the overarching goal of material characterization.
Understanding and applying these principles allows researchers to derive deeper insights into material behavior at the atomic level.
Types of STM Images
Understanding the different types of STM images is crucial in comprehending how scanning tunneling microscopy contributes to the fields of nanoscience and materials characterization. Each type of image offers unique insights into material properties, atomic structures, and electronic states. The distinctions among topographic, spectroscopic, and current-voltage characteristics images allow researchers to select the most suitable imaging technique for their specific objectives. The choice of STM image type can significantly influence data interpretation and subsequent research decisions.
Topographic Images
Topographic images are the most commonly produced STM images. They provide detailed maps of the surface topography of a sample at the atomic level. This is achieved by tracking the variations in tunneling current as the STM tip scans across the surface. The resulting images reflect the contours and features of the surface morphology, allowing for accurate measurements of height fluctuations at the nanoscale.
The information derived from topographic images is particularly valuable for material science applications. Researchers can assess features like step edges, defects, and other structural irregularities. When analyzing atomic arrangements, these images can reveal crucial insights into material stability and behavior.
For example, examining a semiconductor's surface can help in understanding its electrical properties and performance in devices. In industries relying heavily on material properties, such as electronics and photonics, the role of topographic images becomes even more critical.
Spectroscopic Images
Spectroscopic images obtained through STM provide more than just topographical data; they also convey information about the electronic states of materials. This type of imaging typically involves techniques such as scanning tunneling spectroscopy (STS), which measures the local density of states of electrons on a surface.
The significance of spectroscopic images lies in their ability to illustrate how varyign electronic properties can alter with the material's surface structure. Insights gleaned from spectroscopic images can inform discussions about electronic transitions and band structures. In applications such as nanotechnology, these images are key to understanding phenomena such as electron tunneling and energy level alignments. Thus, while topographic images focus on geometry, spectroscopic images delve into the fundamental energetic characteristics of materials.
Current-Voltage Characteristics
Current-voltage characteristics, often referred to as I-V curves, offer an additional layer of analysis in STM imaging. This method involves measuring the tunneling current at varying voltage levels, thus establishing a direct relationship between current and voltage.
The information yielded from I-V curves is essential for understanding the electronic properties of a sample. For instance, researchers can gain insights into conductivity, charge transport mechanisms, and the presence of specific electronic states. These curves can be particularly useful when investigating complex materials such as two-dimensional materials or ferroelectric systems, where conventional methods may fall short.
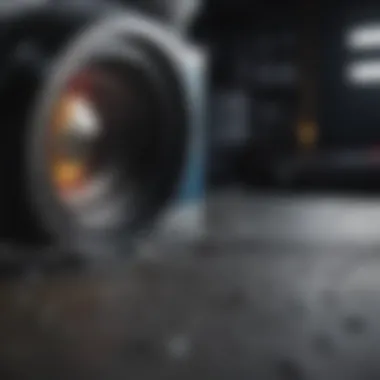
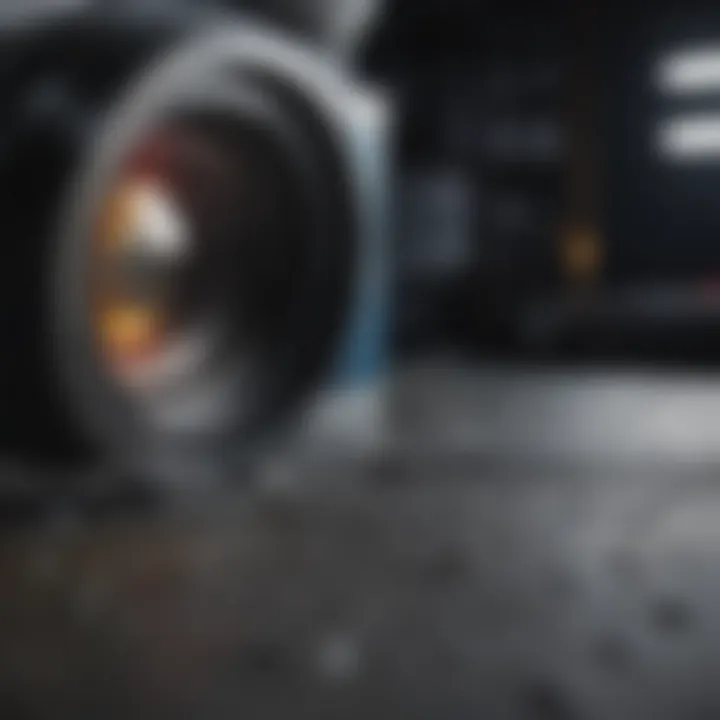
"I-V characteristics can reveal much about the underlying physics of materials, serving as a bridge between experimental observations and theoretical models."
In summary, the myriad types of STM images—topographic, spectroscopic, and current-voltage characteristics—each serve distinct purposes and contribute uniquely to nanoscience research. As techniques continue to evolve, the precision and interpretative power of these images will only increase, further enhancing the understanding of materials at the atomic scale.
Interpreting STM Images
Interpreting STM images forms a critical aspect of utilizing scanning tunneling microscopy for research and application. This process encompasses various methodologies and considerations. Understanding how to accurately interpret these images can greatly enhance material characterization and lead to significant advancements in fields like nanotechnology and material science.
When analyzing STM images, it is essential to consider several factors which influence the outcome. These factors include resolution, surface cleanliness, and tunneling parameters. Each of these aspects can dramatically affect image quality and the data derived from the images. The interpretations drawn from STM images are crucial for making informed conclusions about material properties at the atomic level.
Moreover, the accurate interpretation of STM images is not only beneficial but necessary. It provides insights into the electronic structure and surface properties of materials. This guidance is particularly relevant when characterizing novel materials or those with complex electronic characteristics. Therefore, a firm grasp of the interpretation process enables researchers to extract meaningful information, ensuring that outcomes are scientifically valid.
Effective interpretation of STM images leads to profound implications in both fundamental and applied research.
Critical Factors in Image Analysis
Several critical factors must be considered during the image analysis of STM data. One of the primary elements is the scan parameters, including bias voltage and tunneling current. These parameters dictate the nature of interactions at the atomic scale, thus impacting the resulting images. Additionally, variations in tip sharpness can result in different imaging artifacts, influencing the accuracy of the observations made.
Another vital element is surface cleanliness. Contaminants or adsorbates on the substrate can obscure features in the STM image. It is important to ensure that samples have been properly prepared and that the surface is as clean as possible. This meticulous attention to detail enhances the clarity and reliability of the STM image.
Furthermore, software algorithms used for image processing play a critical role in the final analysis. The methods applied for noise reduction and image enhancement significantly influence the interpretation outcome. Thus, careful selection of analytical techniques and tools is indispensable in producing reliable data from STM images.
Characterization of Surface Structures
Characterizing surface structures through STM images allows scientists to understand the atomic arrangements and electronic properties of materials. One of the benefits of using STM is its ability to provide real-space images with atomic resolution. This capability enables the mapping of surface defects, reconstructions, and other structural features, making it a versatile tool in material science research.
In STM images, unique patterns often reflect the underlying atomic lattice. Researchers can identify various surface structures, including monolayers, multilayers, or nanostructures. This detailed observation is crucial for evaluating new materials or technologies, such as graphene or semiconductor devices.
Moreover, the interpretation of spectroscopic STM images can reveal insights into local electronic states. By analyzing variations in the tunnel current, researchers can gather information on the density of states at the surface level. This information can greatly inform the understanding of electronic properties, guiding future material design.
In summary, interpreting STM images is a multifaceted process that demands careful consideration of several critical factors. The ability to accurately characterize surface structures arises from a thorough understanding of the technology's capabilities and limitations. By focusing on these aspects, researchers can derive valuable insights that contribute to progress in nanoscience and material characterization.
Applications of STM Imaging
The significance of scanning tunneling microscopy (STM) extends far beyond mere imaging of surfaces at the atomic level. In recent years, the applications of STM imaging have expanded across various scientific disciplines. This section will explore how STM plays a crucial role in multiple fields, particularly in material science, biological sample imaging, and electronics and nanotechnology. Each application emphasizes benefits, methodologies, and considerations that highlight STM's importance within these contexts.
Material Science Applications
In the realm of material science, STM is invaluable for its capacity to provide high-resolution images of materials' internal structures. This technology enables researchers to observe surface properties, mechanisms of growth, and atomic arrangements. With STM, scientists can characterize novel materials, such as graphene and carbon nanotubes, facilitating progression in nanotechnology.
Key advantages include:
- Atomic Resolution: STM offers unmatched resolution, allowing direct observation of individual atoms and molecules.
- Surface Reconstruction Studies: Researchers can investigate surface changes under various environmental conditions, leading to insights about stability and reactivity.
- Electrical Properties: The technique aids in examining the electrical conductance of materials at the nanoscale.
The role of STM in endeavors like nano-engineering adds great value, helping to synthesize materials tailored for specific applications, such as catalysts or semiconductors.
Biological Samples Imaging
When applying STM in biological research, scientists aim to explore the nanostructures of cell membranes, proteins, and DNA. High-resolution imaging capabilities allow high-fidelity images of biological samples without the need for extensive sample preparation. This is especially critical, as many biological structures are sensitive to environmental changes.
Important considerations for STM with biological samples include:
- Sample Conductivity: Biological samples typically require conductive coatings or are studied under certain conditions to facilitate imaging.
- Moisture Conditions: Maintaining a suitable environment during imaging is essential to preserve biological activity and structure.
- Health and Safety: Biological materials must be handled under appropriate conditions to avoid contamination or degradation.
Through these applications, STM contributes significantly to the understanding of fundamental processes in biology, offering exploratory pathways in drug discovery and understanding molecular interactions.
Electronics and Nanotechnology Research
The impact of STM in electronics and nanotechnology is profound. Researchers utilize it to investigate semiconductor interfaces, metal-insulator transitions, and charge transport mechanisms. Gaining insights into the electronic properties at the nanoscale is crucial for advancing electronic devices and improving efficiency.
Benefits of STM in this sector include:
- Device Characterization: STM assists in the development of nanoscale electronic components, critical for future technology.
- Analysis of Surface States: Understanding surface electronic states aids in the design of better materials for energy efficiency.
- Technology Integration: STM findings guide the integration of new materials into existing technology, enhancing performance.
The interdisciplinary nature of electronic and nanotechnology research encourages collaborations that push the boundaries of current capabilities. As STM technology evolves, its potential applications are also expanding, which can play a significant role in future innovations.
STM’s versatility makes it an essential tool across diverse scientific fields, pushing the frontiers of knowledge.
Overall, the implications of scanning tunneling microscope imaging stretch across multiple disciplines, establishing it not only as a tool for observation but also as a fundamental catalyst for discoveries in material science, biology, and electronics.
Challenges in STM Imaging
Scanning tunneling microscopy (STM) is a pivotal tool in nanoscience for its capability to visualize surfaces at atomic resolution. However, it is not without its challenges. Understanding these challenges is crucial for both researchers and practitioners in the field. Knowing the limitations and sources of error can help in refining techniques and developing best practices for image acquisition and analysis. This section outlines two significant challenges: limitations of current technology and issues related to data noise and image artifacts.
Limitations of Current Technology
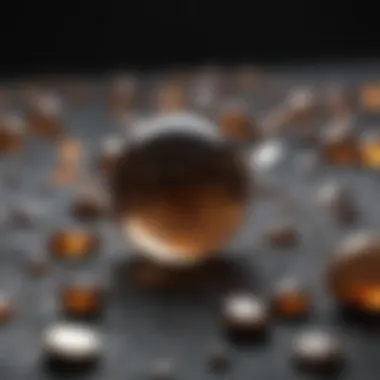
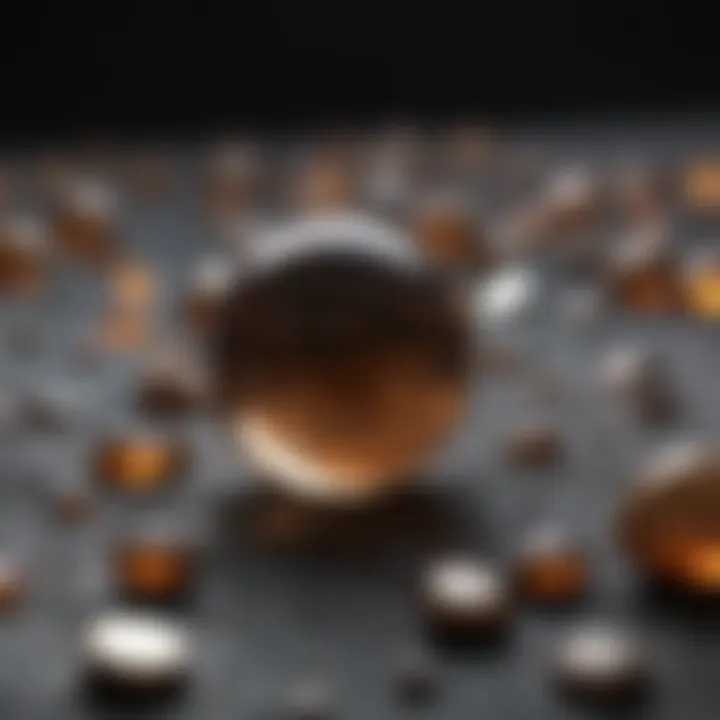
Despite the remarkable capabilities of STM, current technology imposes several limitations. One primary concern is the necessity for ultra-high vacuum conditions. STM instruments require an environment free of contaminants to avoid interference with the scanning probe. This restricts studies to a limited range of conditions, which may not always be representative of real-world scenarios.
Additionally, while STM achieves atomic resolution, it often struggles with the characterization of larger surface areas. The time-consuming nature of scanning can render STM impractical for the examination of larger materials, limiting the technique's applicability in certain fields. There is also a dependence on the conductive nature of materials, meaning insulating or poorly conducting materials pose challenges for imaging.
"The need for conducive atomic surfaces limits the breadth of materials that STM can analyze effectively."
Another challenge is the thermal drift that can occur during imaging, leading to distortions in the final pictures. This drift is a function of temperature variations and can diminish image quality. Overall, while STM represents a significant advancement in imaging technology, it comes with inherent limitations that researchers must navigate effectively.
Data Noise and Image Artifacts
Another major hurdle in STM imaging is the presence of data noise and image artifacts. The open-loop nature of STM makes it susceptible to various types of noise. This noise can arise from electrical sources, thermal fluctuations, and even vibrations in the environment. These disturbances can obscure the true signal being measured and lead to inaccuracies in both the image data and the subsequent analysis.
Image artifacts can stem from several sources, including poor tip conditions or scanning parameters. If the STM tip is contaminated or worn out, it can produce misleading images that do not accurately represent the sample surface. Additionally, improper scanning speed or feedback settings may introduce artifacts, complicating the interpretation of the images.
To mitigate these issues, it is essential to adopt rigorous calibration protocols and employ noise reduction techniques during both image acquisition and analysis. By understanding the origin of noise and actively attempting to minimize its impact, researchers can significantly enhance the quality of STM images.
In summary, the challenges in STM imaging, involving limitations of current technology and issues with data noise and artifacts, play a vital role in shaping the advancement of this field. Addressing these challenges not only improves image quality but also expands the potential applications of STM in material science and beyond.
Advancements in Scanning Tunneling Microscopy
The field of scanning tunneling microscopy (STM) is witnessing continuous evolution, driven by the need for precision and enhanced capabilities in imaging. Advancements in this technology not only improve the resolution of images but also expand the applicability of STM across various scientific disciplines. These developments often focus on refining the mechanisms for image acquisition, enhancing the fidelity of the data captured, and enabling new types of analyses that were previously unattainable.
One significant area of advancement is the development of new materials and tips used in STM setups. This includes innovations in the composition and fabrication of scanning tips that significantly affect the tunneling current, thereby impacting image quality. Tips made from various materials, such as carbon nanotubes or metallic alloys, promote better stability and resolution at the nanoscale level. As a result, researchers can observe subtler features in the surface topology of materials.
Moreover, software and image processing algorithms have seen substantial enhancements. The ability to interpret complex data from STM scans has improved through advanced algorithms that can filter noise and enhance signal clarity. These innovations enable more accurate representations of atomic structures and surface phenomena, leading to greater insights into material properties.
In addition, the integration of automation and AI in STM is becoming increasingly reiterated. Automated systems minimize human error and enhance reproducibility in achieving high-quality STM images. Furthermore, artificial intelligence algorithms can accelerate image analysis and interpretation, turning large datasets into valuable information much faster.
Emerging Techniques in STM
Emerging techniques in STM signify the efforts to push the boundaries of traditional methods. One notable method is non-contact atomic force microscopy (AFM) used in conjunction with STM, which allows researchers to acquire more detailed images without the risk of damaging sensitive surfaces. This advancement opens doors to studying soft materials that were previously difficult to analyze under STM alone.
Additionally, scanning tunneling spectroscopy (STS) has advanced significantly. STS permits researchers to explore electronic properties in greater depth and detail. This technique allows the measurement of current as a function of applied voltage, where conducting the measurements at a finer scale reveals nuances about material behavior and electronic states.
These new methods represent a pivotal shift in how scientists explore and understand atomic structures, facilitating discoveries that contribute to advancements in nanotechnology.
Integrating STM with Other Techniques
Integrating STM with other scientific techniques is crucial for a deeper understanding of material properties. For example, combining STM with X-ray photoelectron spectroscopy (XPS) enables researchers to gather elemental and chemical state information alongside topographic data. This kind of integration offers a more comprehensive analysis of complex materials.
Another valuable combination is with transmission electron microscopy (TEM), where STM provides surface information while TEM offers insights into bulk properties. Together, these techniques foster a more holistic view of material characteristics at different scales.
In summary, the ongoing advancements in scanning tunneling microscopy amplify its relevance across various fields. These improvements not only enhance imaging quality but also broaden the range of materials and structures that can be explored. By integrating STM with other techniques and employing emerging methods, researchers can continue to unravel the intricate details of materials at the atomic level. This foundation is essential for future innovations and applications, reaffirming the pivotal role of STM in advancing nanoscience.
Future Directions in STM Research
The realm of Scanning Tunneling Microscopy (STM) is constantly evolving. Each innovation opens new avenues for exploration in various scientific fields. This section examines the future directions in STM research, emphasizing the importance of upcoming technologies and interdisciplinary approaches. As researchers push boundaries, understanding these advancements is crucial for harnessing the full potential of STM in the global scientific community.
Potential Innovations in Imaging Techniques
Future innovations in imaging techniques may significantly enhance the capabilities of STM. One promising area is the development of faster scanning methods. Current techniques can be time-consuming, especially for large-scale imaging. Innovations like high-speed scanning using advanced piezoelectric actuators are in progress. These would enable researchers to capture dynamic changes in surface structures in real-time.
Another area of development is the integration of artificial intelligence in image processing. Machine learning algorithms could analyze vast amounts of STM data effectively. This could lead to quicker interpretation of results while reducing human error in data analysis. This integration is not just a theoretical concept; multiple organizations are experimenting with these methods, showing promising preliminary results.
Moreover, advancements in materials used for STM probes are on the horizon. Researchers are exploring new conductive materials that could offer better resolution and stability. This would fundamentally alter the quality of data collected, leading to more accurate representations of atomic structures.
Interdisciplinary Applications and Collaborations
Interdisciplinary collaboration represents a critical future direction in STM research. The unique interplay of nanotechnology, biology, and materials science brings together diverse perspectives. By merging these fields, researchers can develop comprehensive frameworks for understanding complex materials at the atomic level, achieving results otherwise unattainable.
One notable example is the collaboration between material scientists and biologists. STM imaging can provide insights into the behavior of biomolecules. This interaction can help in drug development and understanding disease mechanisms. Applying STM in biological studies can also shed light on how biological systems behave at nanoscale levels, leading to significant breakthroughs in medical science.
Additionally, partnerships with industry can foster practical applications of STM technology. By collaborating with tech companies, research can drive innovations that translate into real-world solutions. This could involve the development of more robust nanoscale devices, impacting fields such as electronics and energy.
"The integration of diverse fields will foster innovative solutions that redefine our understanding of science and technology."
In summary, the future of STM research is bright, characterized by potential innovations in imaging techniques and a growing emphasis on interdisciplinary collaborations. These directions promise to expand our understanding of material properties and enhance the practical applications of Scanning Tunneling Microscopy in modern science.
Epilogue
The conclusion of this article is pivotal as it encapsulates the overarching significance of scanning tunneling microscopy images in nanoscience. It offers a summation of the key insights gained through the discourse, emphasizing the intricate relationship between technology and material characterization at the atomic level.
Summarizing Key Insights
Throughout the article, various aspects of scanning tunneling microscopy have been explored, shedding light on its importance in modern science. The essential insights include:
- Fundamentals of STM: Understanding its operational principles is crucial for effective utilization and innovation.
- Image Acquisition and Resolution: The acquisition techniques significantly impact the quality of images produced. Factors like scanning speed and tip-sample distance must be managed with precision.
- Application Diversity: STM is not limited to materials science; its application extends to biological samples and nanotechnology, underscoring its versatility.
- Challenges Faced: The limitations inherent in current technology and issues like data noise are crucial considerations for researchers.
- Future Directions: The article reflects on emerging techniques and interdisciplinary collaborations that may redefine research frontiers.
Each point here highlights not only the operational mechanics of STM but also its broader implications in scientific inquiry.
The Importance of Continued Research
Continuing research in scanning tunneling microscopy is essential for advancing our understanding of materials at the nanoscale. Future innovations can enhance image quality and minimize data noise, further enabling accurate analyses of materials. Collaboration between different scientific disciplines will also foster new methodologies and applications. Institutions and researchers should allocate resources and attention to STM research, as it holds promise for breakthroughs in various fields, particularly in nanotechnology and material science.
Ongoing investment in STM technology is not merely an enhancement of techniques but a crucial step toward deeper insights into material behavior at fundamental levels.