Comprehensive Guide to Gel Electrophoresis Techniques
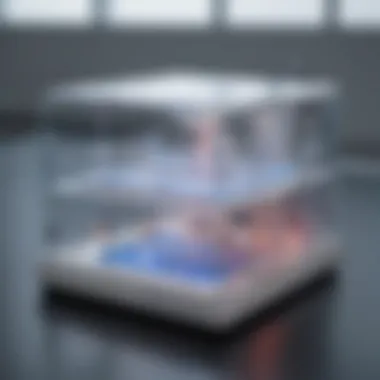
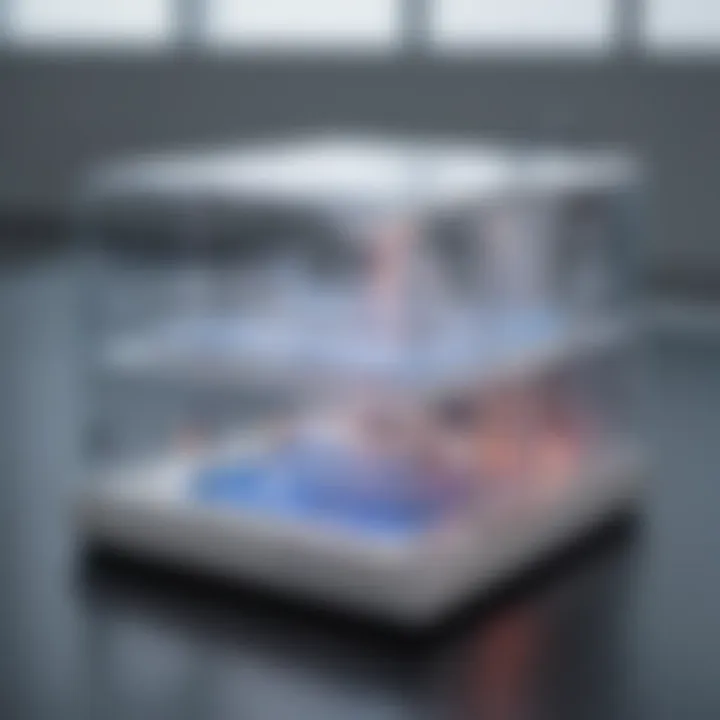
Intro
Gel electrophoresis is a technique that stands at the forefront of molecular biology. It allows scientists to separate nucleic acids and proteins based on their size and charge. This separation is crucial for various applications, including DNA analysis, protein expression studies, and genetic fingerprinting. Understanding the procedural insights into gel electrophoresis can enhance the reliability and accuracy of experimental results.
In this article, we aim to delve into the methodological approaches that define this technique and the practical considerations associated with its use. By examining the foundational concepts and current practices, we will not only illuminate the significance of gel electrophoresis in research but also provide a resource that benefits students, researchers, educators, and professionals alike.
Prolusion to Gel Electrophoresis
Gel electrophoresis is a fundamental technique in molecular biology, crucial for analyzing the size, charge, and purity of nucleic acids and proteins. This method enables researchers to separate biological macromolecules within a gel matrix subjected to an electric field, unlocking insights into their biochemical properties. With an ability to facilitate genetic research, disease diagnostics, and development of biopharmaceuticals, mastering gel electrophoresis becomes integral for academics and professionals alike.
Definition and Purpose
Gel electrophoresis refers to the process of separating charged molecules, like nucleic acids and proteins, based on their size and charge. When an electric field is applied, molecules move through a gel matrix made of agarose or polyacrylamide. The movement depends largely on their size; smaller molecules travel faster than larger ones.
The primary purpose of this technique is to analyze and characterize biomolecules. In genetic research, it aids in assessing the quality of DNA samples, while in protein analysis, it allows for examining protein expression and purity. This versatility makes gel electrophoresis a crucial component in laboratories worldwide.
Historical Development
The concept of gel electrophoresis was first explored in the mid-20th century. Initially, researchers used simple forms of electrophoresis, but advancements led to the development of agarose gel in the 1970s. Scientists began to recognize the potential of this technique in molecular biology. Over the years, researchers refined protocols and gel compositions, leading to the establishment of polyacrylamide as a versatile medium for complex protein separations.
Noteworthy is the introduction of various staining techniques that allow visualization of molecular bands after electrophoresis. Techniques like ethidium bromide staining revolutionized the field, making the analysis non-destructive and efficient. As innovations continued, gel electrophoresis became more widely adopted across diverse scientific disciplines, marking its significance in both research and clinical settings.
Fundamental Principles of Electrophoresis
Electrophoresis is a critical technique in molecular biology, enabling the separation of molecules like nucleic acids and proteins based on their characteristics. Understanding the fundamental principles of electrophoresis is essential for effectively applying the technique in research and diagnostics. This knowledge allows the user to optimize conditions for better resolution and results.
The Role of Electric Field
The electric field is paramount in electrophoresis. It drives the charged molecules through the gel medium. The strength and direction of the electric field influence the speed at which molecules migrate. A higher voltage typically increases migration speed, but care should be taken to avoid overheating the gel. Keeping the electric field stable is important to achieve consistent results under various experimental conditions.
Migration of Molecules
Molecules in an electric field move towards the electrode of opposite charge. For example, negatively charged nucleic acids move toward the positive electrode. The migration rate is determined by the charge-to-mass ratio of the molecule. Larger or more complex molecules may migrate more slowly compared to smaller, simpler ones. Therefore, knowing how molecules move helps in selecting the right conditions for separation.
Factors Affecting Mobility
Several factors impact how quickly and effectively molecules migrate through the gel. Understanding these can improve the outcomes of gel electrophoresis.
Size of Molecule
The size of the molecule largely determines its mobility in the gel. Larger molecules face more resistance from the gel matrix, which slows their movement. Conversely, smaller molecules travel faster. This relationship between size and mobility is crucial when deciding on gel concentration. If a higher resolution for smaller fragments is needed, a gel with higher concentration can be used, while a lower concentration is effective for larger molecules.
Charge
The charge of the molecule is another vital factor. Molecules with a greater net negative or positive charge will move faster toward their respective electrodes. Therefore, variations in pH can alter the charge of biomolecules, thus impacting their separation during electrophoresis. Carefully controlling pH ensures reproducible results and desired separation outcomes.
Gel Composition
The gel composition is an important aspect that influences the electrophoresis process. Different materials, such as agarose or polyacrylamide, offer various pore sizes, which affects molecule mobility. Agarose gels are suitable for separating larger nucleic acids, while polyacrylamide gels provide better resolution for smaller fragments. The choice of gel composition can greatly affect resolution, efficiency, and clarity of the resulting bands. Understanding these aspects aids in selecting the most appropriate gel type for specific applications in electrophoresis.
Key Takeaway: Understanding the fundamental principles of electrophoresis, including the roles of the electric field, molecule migration, and factors affecting mobility, plays a vital role in optimizing this technique for effective research and diagnostics.
Materials Required for Gel Electrophoresis
The materials used in gel electrophoresis are fundamental to the method's success. Each component plays a specific role, influencing the effectiveness of the separation of nucleic acids or proteins. Understanding the various materials can help ensure optimal results, making it critical for both novice and experienced practitioners.
Gel Preparation Materials
Agarose
Agarose is a polysaccharide derived from seaweed, widely employed in gel electrophoresis for separating nucleic acids. Its importance lies in its gel formation properties, allowing for a versatile matrix. Agarose gels provide adequate resolution to analyze fragments of various sizes, from small oligonucleotides to large DNA fragments.
One key characteristic of agarose is its adjustable concentration, which allows for tailoring the gel for specific applications. Concentrations typically range from 0.5% to 2%, with lower concentrations facilitating the separation of larger fragments. This adaptability makes agarose a common choice in laboratories.
The unique feature of agarose is its ability to form a porous gel, permitting the migration of molecules based on size. However, it may not provide sufficient resolution for separations at the protein level, where polyacrylamide is often favored.
Polyacrylamide
Polyacrylamide is a synthetic compound that is valued for its high resolution and adaptability in protein and nucleic acid analysis. When it comes to resolving small differences in molecular size, polyacrylamide gels prove more effective than agarose gels. Their ability to provide tighter control over pore size is a significant factor here, often making polyacrylamide the preferred choice for detailed protein analysis.
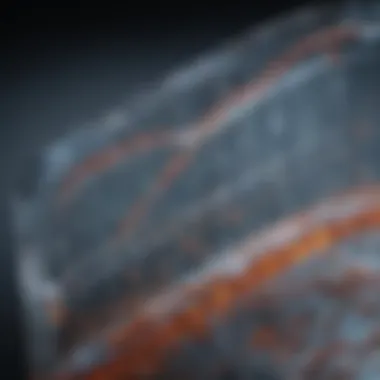
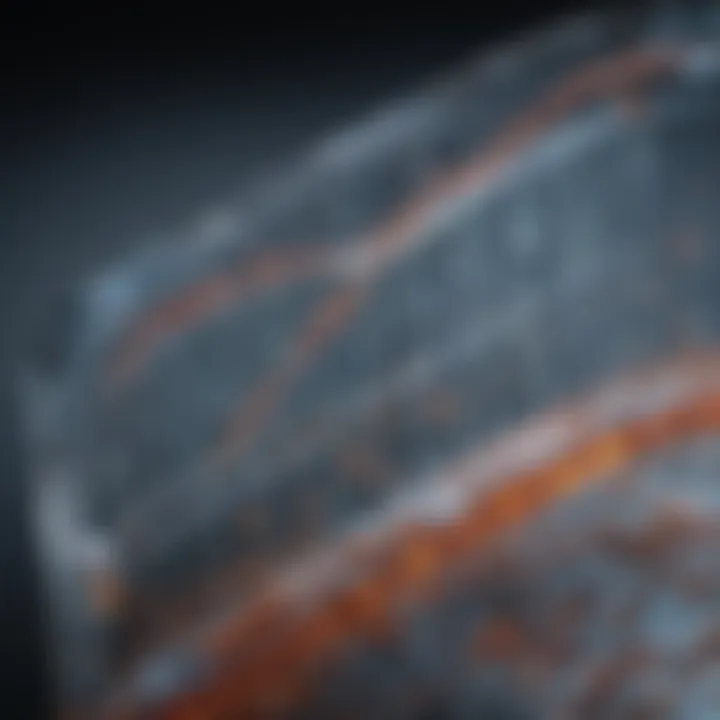
The standout characteristic of polyacrylamide is its gradient formation capability, which enhances the separation of closely sized molecules. This is particularly beneficial in methods like SDS-PAGE, where precision is paramount.
Despite its advantages, the preparation of polyacrylamide gels can be more labor-intensive and requires special precautions, as acrylamide is a neurotoxin before polymerization. This must be considered in practical applications.
Buffer Solutions
Buffer solutions maintain a stable pH environment during electrophoresis, which is crucial for the integrity of samples. Commonly used buffers include TAE (Tris-Acetate-EDTA) and TBE (Tris-Borate-EDTA). These buffers help mitigate pH changes that can occur due to ion migration during electrophoresis.
The essential characteristic of buffer solutions is their ionic composition, which can influence the conductivity of the gel as well as the migration rates of different molecules. Appropriate buffer choice can enhance resolution and the overall quality of results.
One notable feature of buffers is their ability to solubilize nucleic acids and proteins, ensuring that they remain in a stable state during the separation process. Nevertheless, the choice of buffer should align with the type of analyte and experimental objective.
Electrophoresis Apparatus
The equipment needed for gel electrophoresis is just as important as the materials. Each part of the apparatus contributes to the functionality and efficiency of the electrophoresis process.
Power Supply
A power supply is critical for creating the electric field necessary for electrophoresis to occur. It delivers a steady current, allowing molecules to migrate through the gel. The voltage applied can greatly influence the speed and separation quality of the molecules.
A key characteristic of the power supply is its ability to regulate voltage and current. This flexibility is beneficial for optimizing electrophoresis conditions based on specific experimental parameters.
High-quality power supplies often offer consistent performance and reduce the risk of overheating the gel, which can lead to artifacts in the results. However, it is essential to select a power supply compatible with the gel size and expected current levels.
Gel Casting Equipment
Gel casting equipment sets the foundation for gel preparation. Essential components include casting trays, combs, and accessories that ensure the gel solidifies correctly. Proper equipment is necessary to form uniform gels, which directly impacts separation effectiveness.
The essential feature of gel casting equipment is its design for easy assembly and disassembly. This usability is beneficial for researchers who require frequent gel preparations as it saves time and reduces contamination risks.
Additionally, the choice of casting equipment can impact the ease of gel handling and image quality post-electrophoresis. It is vital to consider this aspect for consistent experimental outcomes.
Staining Protocols
Staining protocols play a vital role in visualizing separated molecules after they have migrated through the gel. Common staining methods include ethidium bromide for DNA and Coomassie Brilliant Blue for proteins. The selection of a staining method can significantly affect sensitivity and specificity in visualization.
A notable characteristic of staining protocols is their ability to bind selectively to specific molecules. This helps in accurately assessing the presence and quantity of analytes after electrophoresis.
Good staining protocols are straightforward and allow for rapid visualization while minimizing background noise. However, some stains, like ethidium bromide, require careful handling due to their mutagenic properties.
Creating an Agarose Gel
Creating an agarose gel is a fundamental step in the gel electrophoresis process. It involves several considerations that directly impact the separation and analysis of biomolecules such as nucleic acids and proteins. The gel acts as a molecular sieve, permitting smaller fragments to travel faster than larger ones under the influence of an electric field. Understanding how to create a suitable agarose gel is essential for obtaining reliable and reproducible results in experiments.
Choosing the Appropriate Concentration
The concentration of agarose is a critical factor influencing the gel's resolving power. A lower concentration, typically around 0.5-1%, is suitable for larger DNA fragments above 10 kilobases, while a higher concentration, generally between 1.5-3%, is preferred for separating smaller fragments up to 500 base pairs. Choosing the correct concentration enables the gel to resolve different sizes effectively.
When selecting the appropriate concentration, keep the following in mind:
- The size of the nucleic acids or proteins intended for analysis.
- The desired resolution—higher resolution requires a higher concentration.
- The gel's thickness, which might require adjustments in concentration for optimal performance.
Casting the Gel
Casting an agarose gel involves preparing the gel solution and pouring it into a casting tray. Begin by measuring the required quantity of agarose powder according to the previously determined concentration. Heat it with buffer solution until it dissolves completely. Ensure that the agarose solution is clear and free from any undissolved particles.
After cooling slightly, pour the agarose solution into a casting tray with a comb embedded to form wells. Allow the gel to solidify at room temperature or in a refrigerator to accelerate the process. Solidification usually takes 20-30 minutes. Once solidified, carefully remove the comb to create wells for sample loading. This procedure ensures that the gel maintains its structural integrity, allowing for precise molecular separation during electrophoresis.
By following these detailed steps to create an agarose gel, researchers can improve the quality of their electrophoresis experiments, leading to clearer, more interpretable outcomes.
Sample Preparation
Sample preparation is a vital step in the gel electrophoresis process. The quality and nature of the sample can significantly influence the outcome of the experiment. Proper sample preparation ensures that the molecules are in a suitable state for electrophoretic separation, thereby leading to reliable and reproducible results. This section will explore the nuances in selecting appropriate sample types, as well as the importance of dilution and loading buffers.
Selecting the Appropriate Sample Type
Choosing the right sample type is crucial for efficient and accurate electrophoresis outcomes. Two primary categories of samples often analyzed are nucleic acids and proteins, each having distinct characteristics.
Nucleic Acids
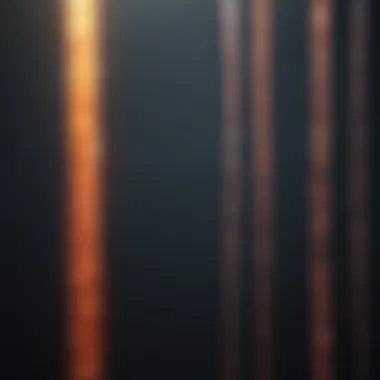
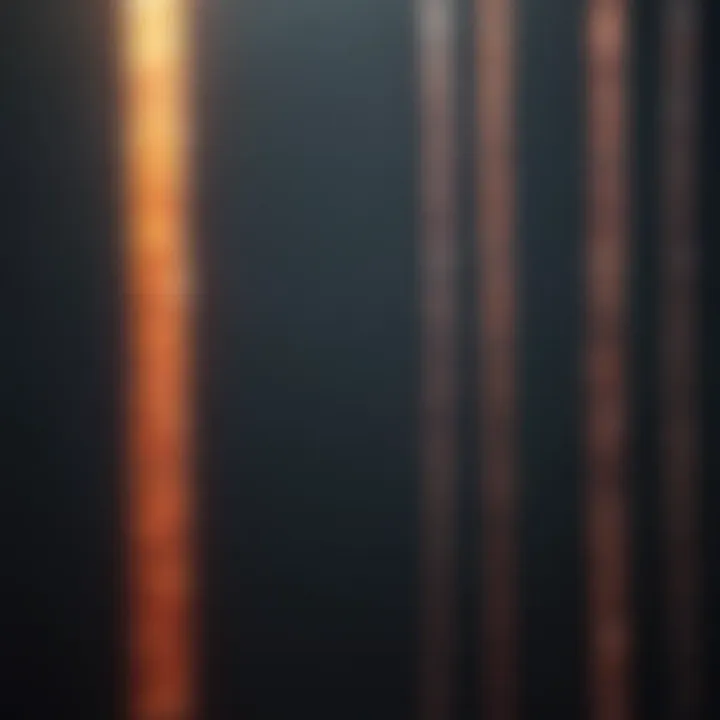
Nucleic acids, including DNA and RNA, are commonly used in gel electrophoresis. Their high polarity and uniform charge-to-mass ratio are key characteristics that make nucleic acids ideal for separation by this method. In molecular biology, nucleic acids play a central role in various applications such as genetic fingerprinting, sequencing, and cloning.
One unique feature of nucleic acids is their predictable migration through the gel matrix based on size. Smaller molecules migrate faster than larger ones, allowing for a clear resolution of size differences. However, nucleic acids can be susceptible to degradation from nucleases, which is a potential disadvantage if proper handling techniques are not observed. Ensuring the integrity of the sample is essential prior to electrophoresis to obtain accurate results.
Proteins
Proteins are another critical category analyzed through gel electrophoresis. The complexity of proteins often lies in their diverse structures, sizes, and charges. Each protein's characteristics can affect its migration rate in a gel.
Proteins are popular in various applications including enzyme assays, and investigating interactions among biomolecules. A key advantage of protein analysis is that many proteins can be visualized and quantified using specific staining methods. The challenges with proteins, however, include their possible denaturation during sample preparation. This denaturation can result in loss of functional and structural properties, affecting the electrophoresis outcome.
Dilution and Loading Buffers
The role of dilution and loading buffers in gel electrophoresis cannot be overstated. These buffers ensure that the samples are in the optimal concentration and pH for electrophoresis.
Just the right dilution will provide a good balance in visibility during migration, which helps with assessing band intensity and separation clarity.
Loading buffers generally contain a dye for visual tracking and a dense component like glycerol to ensure that the sample stays at the bottom of the well during loading. The use of loading buffers standardizes sample entry into the gel, maintaining consistency across experiments. Without properly prepared samples, one may encounter issues such as band smearing or distortion that can compromise the results.
In summary, effective sample preparation—through appropriate selection of sample type and careful formulation of dilution and loading buffers—sets the foundation for successful gel electrophoresis. Each choice affects the subsequent analytical outcomes, making this step essential for reliable and valid results.
Running the Gel Electrophoresis
In gel electrophoresis, running the gel is a crucial phase where the actual separation of biomolecules takes place. This phase transforms the prepared gel and samples into a functional tool for analysis. Proper execution directly influences the clarity, resolution, and overall success of the results. Understanding the dynamics involved during this phase can lead to significant enhancements in experimental outcomes.
Setting Up the Apparatus
The first step in running gel electrophoresis involves the diligent setup of the apparatus. It is vital to ensure that every component is correctly arranged to avoid complications later. The key elements include the gel box, the electrodes, the buffer, and the gel containing the samples.
Start by placing the gel in the gel box. Ensure the wells are facing the positive electrode. It is important to fill the gel box with running buffer till it covers the gel adequately. The buffer acts as the medium through which the current will pass, and it also maintains the pH, crucial for preserving the integrity of nucleic acids or proteins. Next, connect the electrodes to the power supply, confirming that they are firmly attached and correctly oriented. Verify that the settings on the power supply are appropriate for the specifics of your experiment, as this will determine the voltage and current during the run. Adjusting these parameters is fundamental. Too high settings can lead to overheating or smearing, while too low can impede the desired resolution.
Operating the Power Supply
Once the apparatus is set, the next step is to operate the power supply. This entails powering on the equipment and observing the initial current flow through the system. Adjust settings based on the type of samples and gel used. A common approach is to start at a low voltage to ensure even distribution of the current.
Monitor the runtime, as the electrophoresis can last from 30 minutes to several hours, depending on the size of the molecules and gel concentration. Always keep an eye on the progress. Staining methods often include tracking dyes that can help visualize the migration of samples. By the end of the run, you should ensure that the separation is adequate based on the expected band positions.
"Running gel electrophoresis is not merely a process; it is the key to unlocking the complex biology hidden within your samples."
Visualizing the Results
The visualization step in gel electrophoresis plays a crucial role in interpreting the data generated from the process. This phase is essential for confirming the presence, size, and quantity of the separated nucleic acids or proteins. By employing effective visualization methods, researchers can obtain vital insights that maximize the utility of their investigative efforts.
Enhancing the clarity of bands or spots on a gel, visualization techniques contribute significantly to the reproducibility and accuracy of experimental results. The ability to discern clear patterns can assist in drawing meaningful conclusions during analysis. Specific details, including gel composition and staining methods, directly and profoundly influence the effectiveness of visualization.
Staining Techniques
Staining techniques are vital for the effective visualization of gel electrophoresis results. These methods allow the target molecules within the gel to display contrasting colors against the background, enhancing the visibility of bands corresponding to nucleic acids or proteins. Among the widely used stains, Ethidium Bromide is one of the most common for DNA visualization. This chemical intercalates between the bases of nucleic acids, fluorescing under UV light. It is essential to follow strict protocols due to its mutagenic properties.
Other stains, such as SYBR Green or Coomassie Brilliant Blue, serve similar functions but can be selected based on specific experimental needs. Choosing appropriate staining techniques involves considering factors like sensitivity, safety, and the type of molecules being examined.
For protein visualization, it’s critical to apply the correct protocol,
- Typically employing coloring agents,
- Balancing factors like gel type and sample concentration.
Using Imaging Systems
After applying staining techniques, utilizing imaging systems helps in capturing and analyzing the results quantitatively. Digital imaging technologies founded in fluorescence and chemiluminescence allow for precise documentation of the gel electrophoresis outcome. These systems can enhance band visibility, enabling users to obtain clear images for analysis.
Many modern imaging systems are equipped with software capable of quantifying band intensity, thus providing data for further statistical analyses. The integration of imaging systems in lab practice promotes higher fidelity in experimental documentation and facilitates more robust data interpretation.
Troubleshooting Common Issues
Troubleshooting is a vital part of performing gel electrophoresis effectively. Poor outcomes can lead to incorrect interpretations of experimental data. Addressing common issues allows researchers to refine their methods and enhances the reliability of their results. This section focuses on two frequent complications: poor resolution and unanticipated band patterns. By understanding these issues, researchers can optimize their procedures and achieve clearer, more interpretable results.
Poor Resolution
Poor resolution in gel electrophoresis can occur due to various factors. When bands do not separate cleanly, it complicates analysis significantly. A few primary reasons for this issue include:
- Concentration of the Gel: Higher concentrations can hinder the mobility of smaller fragments. Conversely, too low a concentration may lead to wider bands and, hence, less resolution.
- Voltage Settings: Using too high of a voltage can lead to overheating and distortion. This can cause the gel matrix to melt and adversely affect result fidelity.
- Buffer Systems: The choice and preparation of buffer solutions influence ion concentration and pH. Inadequate buffering can lead to poor separation.
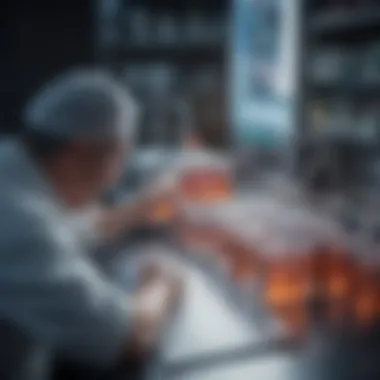
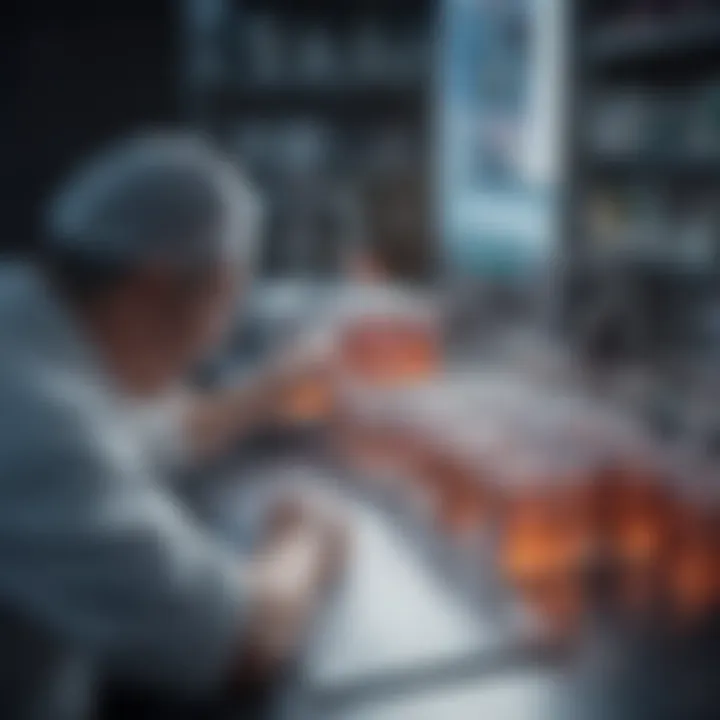
To mitigate poor resolution, start by re-evaluating gel concentration and voltage parameters. Always ensure that buffer solutions are prepared with precision. Another effective strategy is to run a gel with a known DNA ladder alongside your samples. This allows direct comparison and identifies clear size markers for easier analysis.
Unanticipated Band Patterns
Unanticipated band patterns can lead to confusion during analyses. They may arise for various reasons, including:
- Sample Integrity: Degraded nucleic acids or proteins can behave unpredictably. Poor sample storage or handling can be a significant culprit.
- Contamination: Introduction of impurities can result in unexpected bands. Using clean equipment and reagents is paramount.
- Electrophoresis Time: Running the gel for too long can cause bands to diffuse and create artifacts.
To address unanticipated band patterns, it is crucial to maintain high sample integrity. Handle samples with care throughout the experimental procedure. Regular checks for contamination and using appropriate controls can also help safeguard against uneven results. If unexpected bands persist, reevaluate your sample preparation techniques and equipment cleanliness.
The key to effective troubleshooting lies in understanding the variables at play during gel electrophoresis. Fine-tuning these processes can lead to considerably improved outcomes.
Applications of Gel Electrophoresis
Gel electrophoresis has established itself as a fundamental technique with myriad applications across various domains of molecular biology and biochemistry. Its capacity to separate macromolecules based on size and charge makes it invaluable in genetic research and protein analysis, among other fields. Understanding the applications of gel electrophoresis allows researchers and practitioners to utilize its full potential effectively, driving innovation and discovery in their work.
Genetic Research
In genetic research, gel electrophoresis serves several key purposes. It plays an essential role in the analysis of DNA fragments, enabling researchers to visualize specific sequences for genetic mapping, cloning, and sequencing. One of the primary applications is in the assessment of polymerase chain reaction (PCR) products, which are often analyzed via agarose gel electrophoresis to confirm the presence and size of amplified DNA fragments.
The technique is also pivotal in restriction fragment length polymorphism (RFLP) analysis. RFLP involves cutting DNA with specific enzymes and separating the resulting fragments using electrophoresis. This allows for the identification of genetic variations and is widely used in genetic linkage studies and forensic analysis.
Moreover, gel electrophoresis facilitates techniques such as DNA fingerprinting, which is crucial in paternity testing and criminal investigations. The ability to visualize bands corresponding to unique genetic markers ensures that it is reliable and can yield informative results.
Protein Analysis
Protein analysis is another significant application of gel electrophoresis. SDS-PAGE (sodium dodecyl sulfate polyacrylamide gel electrophoresis) is perhaps the most widely known method used in this area. In SDS-PAGE, proteins are denatured and coated with a negative charge, allowing them to be separated based on molecular weight. This technique is crucial for understanding protein structure, function, and post-translational modifications.
The information obtained from SDS-PAGE aids in protein quantification and can help identify aberrant protein forms that may be linked to diseases. Furthermore, gel electrophoresis assists in purification processes such as isolating specific proteins from complex mixtures. This can be fundamental when developing pharmaceuticals or understanding biochemical pathways.
The versatility of gel electrophoresis extends to applications in proteomics, where it is used to analyze complex protein mixtures in systems biology. By integrating gel electrophoresis with mass spectrometry, researchers can achieve a deeper understanding of protein interactions and functions, paving the way for new therapeutic strategies.
Gel electrophoresis remains one of the most powerful techniques for both genetic and protein studies, profoundly impacting research and diagnostics in modern biology.
In summary, gel electrophoresis is indispensable for genetic research and protein analysis. Its ability to simplify complex separations of nucleic acids and proteins enables researchers to derive meaningful insights in various scientific inquiries. As advancements continue, the techniques and applications of gel electrophoresis only seem to grow, solidifying its position as a cornerstone of molecular biology.
Emerging Technologies and Innovations
Emerging technologies play an essential role in enhancing gel electrophoresis techniques. The evolution of material science and automation has greatly impacted the efficacy and reliability of this procedure. With advancing methods, researchers and practitioners can achieve higher resolution, enhanced sensitivity, and increased throughput in their experiments. These innovations not only streamline workflows but also open new avenues for research in molecular biology, genetics, and proteomics.
Advancements in Gel Materials
Recent developments in gel materials have transformed traditional gel electrophoresis. Notably, new formulations of agarose and polyacrylamide gels have been introduced. These materials offer improved mechanical properties, making them easier to handle while maintaining consistent pore sizes for optimized separation. For instance, agarose gels can be made with varying concentrations to suit specific DNA fragment sizes, which allows researchers to tailor their methodologies depending on the size of the nucleic acids or proteins they are analyzing.
Furthermore, modifications such as adding chemical cross-linkers to polyacrylamide can enhance gel stability, which is crucial during long electrophoresis runs. The emergence of specialized gels designed for high-resolution applications is significant, as they can resolve smaller differences in molecular weight.
The introduction of ready-to-use gel kits simplifies the preparation process, reducing time and the risk of errors during gel preparation. This allows researchers to focus more on the analytical phase rather than the preparatory steps, increasing overall productivity.
Integration of Automation
The incorporation of automation in gel electrophoresis represents a transformative step forward. Automated systems can manage pipetting, gel loading, and electrophoresis running conditions, minimizing human error and ensuring reproducibility.
For example, robotic liquid handling systems are being used to prepare samples and load them into gels with precision. These systems enhance the accuracy of sample volumes and minimize cross-contamination risks.
Moreover, automated image processing technology simplifies the analysis of gel bands. Systems equipped with advanced imaging software can analyze gel results swiftly and provide quantitative data in real-time. This integration dramatically reduces the time required to obtain results and enhances data reliability.
The potential of these automated solutions extends to high-throughput applications, where researchers require rapid analysis of numerous samples. Automation not only accelerates the workflow but also facilitates the simultaneous running of multiple experiments, proving invaluable in large-scale studies.
Automation in gel electrophoresis is revolutionizing laboratories by increasing throughput and reducing human error. This progress is vital for research at the molecular level.
Ending
The conclusion serves as an essential element in any article, especially in this comprehensive examination of gel electrophoresis. It brings together the myriad of insights discussed throughout the text while emphasizing the importance of this technique in molecular biology. Gel electrophoresis is not merely a laboratory procedure; it is a cornerstone of genetic research and protein analysis. By elucidating the principles, methodologies, and practical considerations outlined earlier, the conclusion reinforces the key takeaways that readers should walk away with.
One significant aspect of the conclusion is summarizing the key points addressed in the article. This recap reinforces the learned content, providing a clearer mental framework for the audience. Readers can reflect on how the discussed methodologies fit into their research and practical applications. Additionally, the conclusion highlights the evolving nature of gel electrophoresis technology, pointing to its relevance in contemporary scientific inquiry.
Summary of Key Points
- Definition and Purpose: Gel electrophoresis is a technique used for separating nucleic acids and proteins based on size and charge.
- Fundamental Principles: Understanding the electric field's role and the factors that affect molecule mobility is essential for successful execution.
- Materials Required: A practical understanding of the materials needed allows for informed preparation and execution of the process.
- Sample Preparation: Proper preparation of samples, including appropriate buffers, is crucial for obtaining accurate results.
- Running the Gel: Detailed procedural insights ensure the correct setup and operation of the electrophoresis apparatus.
- Visualizing Results: Knowledge of various staining techniques is vital for the effective analysis of separation results.
- Troubleshooting Common Issues: Provisions are necessary to handle potential problems and ensure the fidelity of results.
- Applications: The article discusses the significant implications of gel electrophoresis in genetics and protein studies.
- Emerging Technologies: Recognizing the advancements in gel materials and automation highlights the future trajectory of this technique.
Future Directions
The future of gel electrophoresis is ripe with possibilities. Emerging technologies are paving the way for enhancements in how this technique is implemented. Notably, innovations in gel materials could lead to improved resolution and the ability to separate more complex samples. Automation is another area ripe for exploration, potentially streamlining processes and reducing human error.
Advancements in software used for data analysis also promise to facilitate better interpretation of results. As big data continues to expand in the scientific community, gel electrophoresis could integrate with bioinformatics platforms to provide more comprehensive insights.
Ultimately, the study of gel electrophoresis is likely to evolve, leading to refined methodologies that improve accuracy and efficiency. Keeping abreast of these developments will ensure that researchers can leverage gel electrophoresis's full potential in their investigations.