Exploring the Genetic Code: The Blueprint of Life
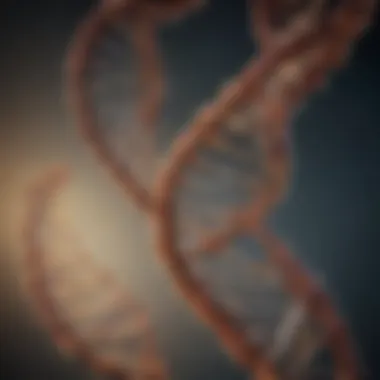
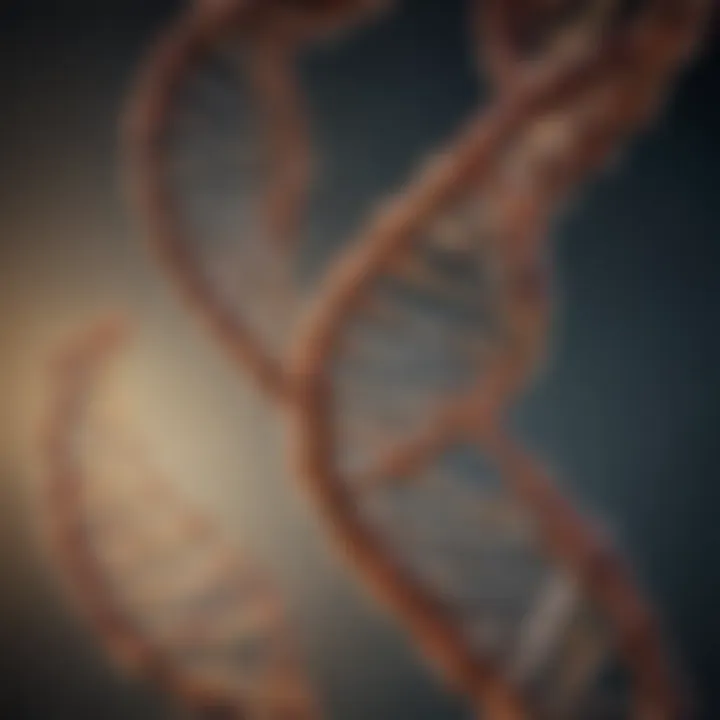
Intro
The genetic code represents a foundational aspect of biology. It is a systematic blueprint that directs the production of proteins in every living organism. At its core, the genetic code consists of sequences of nucleotides which unfold into the breathtaking complexity of life. Understanding how these sequences interact and function can deepen knowledge across various fields such as medicine, biotechnology, and genetic research.
Incorporating insights from evolutionary biology, recent advancements in genomics have reshaped our understanding of heredity and genetic expression. Not only does the research into the genetic code enhance our grasp of fundamental biological processes, but it also paves the way for innovative applications in health and technology.
Research Overview
Methodological Approaches
Researching the genetic code employs numerous methodologies. These include:
- Sequencing Techniques: Techniques such as Sanger sequencing and next-generation sequencing are employed to decode the precise order of nucleotides.
- Bioinformatics Tools: Software programs analyze genetic data, providing insights into genetic variations and mutations.
- Laboratory Experiments: Experimental approaches often validate theoretical models. Techniques such as CRISPR allow for targeted genetic modifications.
Each of these approaches adds a layer of understanding, revealing how DNA is not just a static molecule, but a dynamic participant in biological processes.
Significance and Implications
The study of the genetic code is not purely academic. Its implications are vast and impactful. This research is crucial for:
- Medical Advances: The understanding of genetics has led to the development of personalized medicine, where treatments can be tailored according to an individual’s genetic makeup.
- Agricultural Enhancements: Genetic insights have enabled the engineering of crops to increase yield and resistance to pests.
- Ethical Considerations: As knowledge expands, ethical discourse surrounding gene editing and hereditary interventions becomes more pressing.
"Understanding the genetic code is paramount not just for biology, but for the future of humanity itself."
Current Trends in Science
Innovative Techniques and Tools
Recent advancements in technology have transformed the field of genetics. Innovative techniques, such as CRISPR-Cas9, facilitate precise genome editing. Additionally, single-cell sequencing allows researchers to explore the genomic landscape of individual cells, revealing cellular diversity within tissues.
Interdisciplinary Connections
The genetic code intersects with various disciplines. Bioinformatics merges biology with computer science, leading to groundbreaking discoveries. Collaborations among geneticists, ethicists, and legal experts help navigate the complex implications of genetic research, ensuring responsible advancements.
Overall, these elements combine to elevate the understanding of the genetic code, marking its essential role in life sciences. As we navigate this intricate web, the future possibilities remain boundless.
Prolusion to Genetic Code
The genetic code is a crucial concept in biology as it serves as the foundation for how living organisms inherit traits and function. Understanding the genetic code is essential for comprehension of various biological processes, including heredity, evolution, and even the complex mechanisms underlying diseases. This section introduces key elements that define the genetic code, historical milestones that have shaped our understanding, and the importance of this knowledge in the broader context of biological studies.
Definition of Genetic Code
The genetic code refers to the set of rules by which information encoded within DNA or RNA sequences is translated into proteins, the essential building blocks of cells. In simpler terms, it dictates how sequences of nucleotides are converted into amino acids. Nucleotides, the basic units of DNA, consist of a sugar, a phosphate group, and a nitrogenous base. The code is made up of sequences of three nucleotides, known as codons, where each codon corresponds to a specific amino acid or a signal such as "start" or "stop" during protein synthesis. Understanding this translation process is fundamental to molecular biology and genetics.
Historical Context
The journey to deciphering the genetic code has been a long and intricate one. Early discoveries involved the identification of DNA as the genetic material in the 20th century. Key figures such as James Watson and Francis Crick made significant contributions by proposing the double helix structure of DNA in 1953. This breakthrough laid the groundwork for later research. Further studies revealed the existence of codons and their role in protein synthesis, culminating in the recognition of the universal genetic code in the 1960s. Research by scientists like Marshall Nirenberg and Har Gobind Khorana helped identify specific codons and their corresponding amino acids. These foundational discoveries not only expanded our understanding of genetics but also paved the way for advancements in biotechnology.
Importance in Biology
The genetic code's significance in biology cannot be overstated. It explains how traits are passed from one generation to another. Additionally, it underpins the processes of evolution by providing mechanisms for variation and adaptation. In the context of medicine, understanding this code has led to breakthroughs in gene therapy, where damaged genes can be repaired to treat diseases. Furthermore, the manipulation of the genetic code through techniques like CRISPR has opened new avenues in genetic engineering. This versatility underscores the code's role as a central element in various fields of research, including genetics, molecular biology, and biotechnology.
Understanding the genetic code provides insights not only into the processes of life but also into practical applications that can transform medicine and biotechnology.
Structure of DNA
The structure of DNA is a fundamental aspect of genetics that underpins the entire biosphere. Understanding this structure allows us to appreciate how genetic information is stored, replicated, and expressed in living organisms. The intricacies within its architecture not only illuminate the mechanisms of heredity but also reveal pathways for innovations in biotechnology and medicine. The understanding of DNA structure shapes our approach to various scientific inquiries, making it vital for students, researchers, and medical professionals alike.
Basic Unit: Nucleotide
Nucleotides are the fundamental building blocks of DNA. Each nucleotide consists of three key components: a phosphate group, a sugar molecule, and a nitrogenous base. The sugar in DNA is deoxyribose, which is crucial in distinguishing DNA from RNA, where the sugar is ribose. There are four types of nitrogenous bases found in DNA, categorized into two groups: purines (adenine and guanine) and pyrimidines (cytosine and thymine).
The sequence of these nucleotides encodes genetic information. For instance, specific combinations of these bases determine the traits that may be expressed in an organism, making the configuration of nucleotides essential for everything from physical characteristics to metabolic processes.
Double Helix Model
The double helix model of DNA, proposed by James Watson and Francis Crick in 1953, provides a visual representation of the DNA structure. In this model, two strands of nucleotides coil around each other, forming a helix. Each strand is composed of a sugar-phosphate backbone with nitrogenous bases projecting inward, where they form pairs. This coiling allows for compact storage of genetic data within the cell nucleus and represents an evolutionarily advantageous structure.
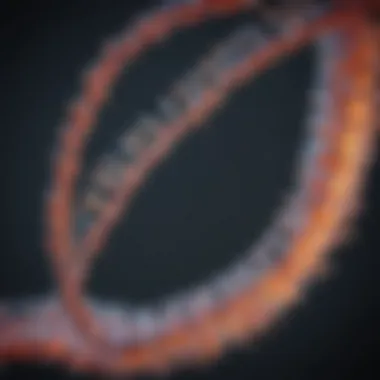
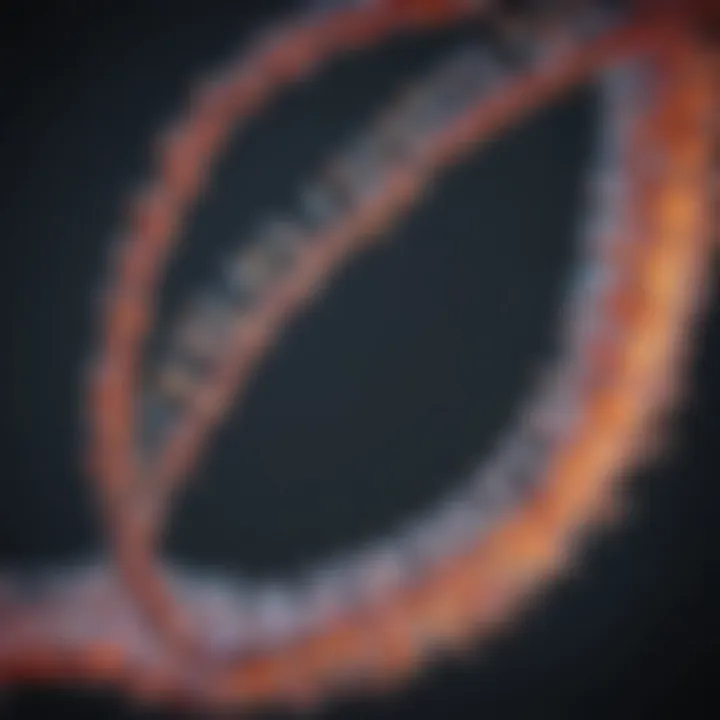
"The double helix structure of DNA is fundamental to understanding how genetic information is copied and transmitted across generations."
Moreover, this arrangement facilitates the process of replication, where each strand can serve as a template for producing a complementary strand. The double helix structure protects the genetic code and maintains integrity during cellular processes.
Base Pairing Rules
Base pairing rules outline how the nitrogenous bases align between the two strands of DNA. Specifically, adenine pairs with thymine, and guanine pairs with cytosine. This specificity is crucial in ensuring accurate replication and transcription processes. The hydrogen bonds formed between the base pairs further stabilize the helix structure.
The significance of these rules becomes evident during DNA replication. The mechanism relies on these pairs to restore the complete DNA molecule. If the base pairing rules are disrupted due to mutations or external factors, it can lead to genetic anomalies with far-reaching implications for an organism's development and health. Understanding base pairing thus opens avenues for studies in mutation effects and potential therapeutic interventions.
Components of the Genetic Code
The genetic code is fundamental to understanding how organisms function. It acts as a universal language for the instructions embedded in DNA. Within this framework, components such as codons, amino acids, and start and stop codons play critical roles. These elements are the building blocks of life, influencing everything from the proteins produced to the traits expressed in living beings. Grasping the intricacies of these components is not only essential from a biological perspective but also impacts areas like genetics and biotechnology.
Codons
Codons are sequences of three nucleotides that correspond to specific amino acids in a protein synthesis process. Each set of three nucleotides, for example, adenine (A), uracil (U), and guanine (G), encodes a signal for the addition of a particular amino acid to the growing polypeptide chain. This triplet code is key to translating the genetic information carried by DNA into proteins, which perform most life functions.
The significance of codons extends beyond individual amino acids; they also determine the overall structure and function of proteins. For instance, the codon AUG not only codes for methionine but also serves as the start signal for translation. Thus, understanding codons is essential for comprehending genetic instructions and their execution.
Amino Acids
Amino acids are organic compounds that serve as the building blocks of proteins. There are twenty standard amino acids that combine in different sequences to form proteins, each with unique properties. The sequence of amino acids in a protein is dictated by the corresponding codons in the messenger RNA (mRNA).
The nature of these amino acids—polar, nonpolar, acidic, or basic—dictates how a protein folds and ultimately functions within an organism. For researchers and students alike, grasping the relationship between amino acids and codons provides insights into protein synthesis and functionality, showing why amino acids are central to life.
Start and Stop Codons
Start and stop codons are critical signals in the translation process of the genetic code. A start codon, most commonly AUG, indicates where protein synthesis should begin. This codon also specifies the first amino acid, methionine. On the other hand, stop codons—UAA, UAG, and UGA—do not code for any amino acid but instead signal the termination of protein synthesis.
Understanding these codons is crucial. Errors in recognizing start or stop signals can lead to nonsensical proteins that disrupt cellular functions. For instance, mutations near these codons can cause frameshift errors or truncated proteins, which are often nonfunctional or deleterious. Thus, start and stop codons not only orchestrate the translation machinery but also help maintain the integrity of gene expression.
"The precise alignment of codons with their corresponding amino acids ensures the fidelity of genetic information during protein synthesis."
Transcription and Translation Processes
Transcription and translation are two essential processes that decode the genetic information contained within DNA, culminating in the production of proteins. These processes allow the cell to interpret the language of the genetic code and convert it into functional entities. Understanding these mechanisms provides insight into how genes are expressed and regulated, which is crucial for various applications in biology and medicine.
Transcription Mechanism
Transcription is the first step in gene expression. It involves synthesizing a complementary RNA strand from a DNA template. This process occurs in the cell nucleus for eukaryotic organisms. Key points about transcription include:
- Initiation: The enzyme RNA polymerase binds to a specific region called the promoter, signaling the start of transcription. This binding initiates the unwinding of the DNA strands.
- Elongation: RNA polymerase moves along the DNA template strand, adding RNA nucleotides complementary to the DNA bases. This results in a growing strand of messenger RNA (mRNA).
- Termination: Transcription proceeds until a termination signal is reached, causing RNA polymerase to detach and release the newly synthesized mRNA strand.
This mRNA then undergoes post-transcriptional modifications, such as splicing, where introns are removed, and exons are joined together. The finalized mRNA exits the nucleus and enters the cytoplasm to participate in the translation process.
Translation Mechanism
Translation is the process by which the mRNA is decoded to synthesize proteins. This occurs within ribosomes in the cytoplasm. The significant phases of translation are:
- Initiation: The mRNA attaches to the ribosome, and the first transfer RNA (tRNA) molecule binds with the start codon on the mRNA, bringing a specific amino acid.
- Elongation: The ribosome facilitates the binding of tRNA molecules to the mRNA sequence, ensuring that amino acids are added in the correct order. Each tRNA carries a specific amino acid, which is linked to the growing polypeptide chain through peptide bonds.
- Termination: The process concludes when the ribosome reaches a stop codon on the mRNA. No tRNA corresponds to stop codons, leading to the release of the completed polypeptide chain, which then folds into a functional protein.
Role of Ribosomes
Ribosomes play a pivotal role in the translation process. They are complex molecular machines composed of ribosomal RNA (rRNA) and proteins. Key functions of ribosomes include:
- Decoding mRNA: Ribosomes read the sequence of the mRNA in sets of three nucleotides (codons), matching each codon with the appropriate tRNA and its corresponding amino acid.
- Catalyzing Peptide Bond Formation: Ribosomes facilitate the formation of peptide bonds between amino acids, effectively linking them to build a protein.
- Ensuring Fidelity: Ribosomes maintain accuracy by ensuring that tRNAs match the correct codons on the mRNA, thus preventing errors in protein synthesis.
Understanding transcription and translation processes is crucial in genetics, biotechnology, and medicine, as alterations to these processes can lead to various diseases and can inform innovative treatments.
Genetic Code Variability
Genetic code variability is a pivotal aspect of molecular biology that influences the way organisms express their genetic information. This variability extends beyond mere genetic sequences, encompassing the nuances of gene expression and the adaptations of organisms to their environments. Recognizing the importance of genetic code variability enhances our understanding of evolutionary processes and the mechanisms behind biological diversity.
Universal Genetic Code
The universal genetic code forms the foundation upon which all known life operates. Nearly all organisms, from simple bacteria to complex mammals, utilize the same codon table to translate nucleic acid sequences into proteins. This uniformity suggests a common evolutionary origin and is evidence for the universality of biological processes.
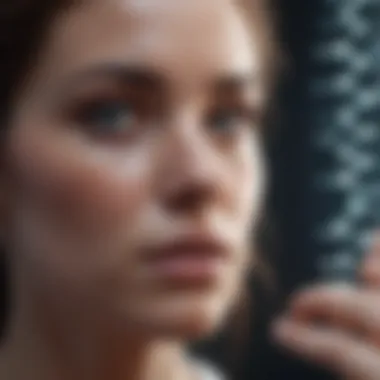
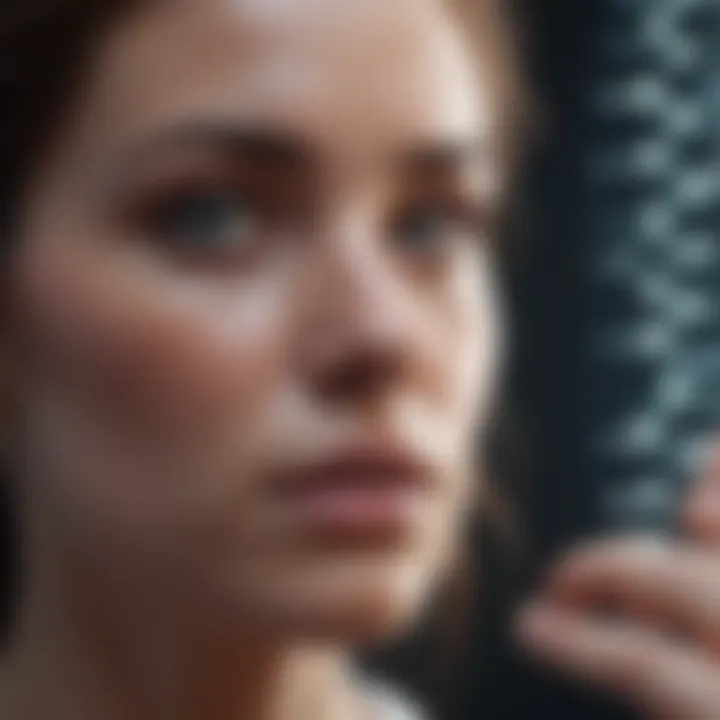
The codons, which are triplets of nucleotide bases in DNA and RNA, correspond to specific amino acids. The following points highlight the significance of the universal genetic code:
- Conservation Across Species: The similarity in genetic coding among diverse organisms suggests profound evolutionary connections.
- Predictable Protein Synthesis: This predictability allows scientists to use model organisms in research, helping to understand human biology and diseases.
- Biotechnological Applications: Genetic engineering relies on the universal genetic code to manipulate organisms for desired traits or medical treatments.
The implications of having a universal genetic code are profound. It allows for the integration of genes across different species, facilitating advances in genetics and bioinformatics.
Exceptions to the Rule
Despite the general consistency of the universal genetic code, there are notable exceptions that complicate the picture. These anomalies provide insights into evolutionary adaptations and the flexibility of genetic processes.
Some of the significant exceptions include:
- Mitochondrial Codons: Certain mitochondrial genomes exhibit variations in their codon assignments. For instance, in some species, AUA codes for methionine instead of isoleucine.
- Ciliate Paramecium: It uses a distinct genetic code, showing that not all organisms strictly follow the universal code. For example, UAA and UAG serve as codons for glutamine in some ciliates.
- Atypical Codon Usage: Some organisms show an unusual preference for particular codons, which can be a result of their unique evolutionary pressures or environmental interactions.
These exceptions indicate that while the genetic code serves as a rule, nature is not bound by it. Variability in the genetic code not only highlights the adaptability of life forms but also prompts deeper questions regarding the evolutionary pathways that led to such phenomena.
"Variation in the genetic code exemplifies nature's adaptability and is critical for understanding evolutionary biology."
Influence of Genetic Code on Organismal Development
The genetic code is fundamentally intertwined with the development of organisms. It is a set of instructions that guides cellular activities, influencing everything from how cells differentiate into various types to how they communicate with each other. Understanding the influence of the genetic code on organismal development is crucial for various disciplines within biology and medicine. This section explores gene expression and genetic regulation, illuminating how the genetic code intricately shapes life.
Gene Expression
Gene expression is the process by which information from a gene is used to synthesize functional gene products, often proteins. This process is tightly regulated and can be influenced by both genetic and environmental factors. The initiation of gene expression begins when RNA polymerase binds to a promoter region of the DNA. This is followed by the transcription of the gene into messenger RNA (mRNA), which then travels to the ribosome for translation into a protein.
The significance of gene expression cannot be overstated. It determines the characteristics of an organism, including physical traits and functionality within various biological systems. For example, different cell types in an organism express unique sets of genes. A muscle cell expresses different genes than a nerve cell, leading to distinctly different structures and functions.
Here are key aspects of gene expression:
- Regulation: Regulating when and how much gene expression occurs is critical for normal development, as unregulated expression can lead to issues such as cancer.
- Environmental Impact: Factors like temperature, nutrition, and stress levels can significantly impact how genes are expressed.
Genetic Regulation
Genetic regulation is the control of the timing and level of gene expression. This includes various mechanisms that cells use to turn genes on or off in response to internal and external signals. One fundamental aspect of genetic regulation is the role of transcription factors, which are proteins that bind to specific DNA sequences, influencing the transcription of downstream genes.
The regulation of genetic expression ensures that the right genes are active at the right times. Here are several critical aspects of genetic regulation:
- Feedback Mechanisms: Many regulatory pathways involve feedback loops, allowing organisms to maintain homeostasis.
- Epigenetic Modifications: Chemical modifications to DNA or histone proteins can alter gene expression without changing the DNA sequence itself. These modifications can be influenced by environmental factors and stress, providing a dynamic layer to genetic regulation.
- Developmental Stages: Different genetic regulation patterns are observed at various developmental stages, guiding an organism from a single cell to a complex multicellular entity.
"Gene expression and genetic regulation are gateways to understanding how organisms develop and adapt."
The influence of the genetic code on development is profound. It dictates not only the physical aspects of living beings but also their abilities to adapt and interact with their environments. As research progresses, greater insight into gene expression and regulation will likely yield novel approaches in fields such as regenerative medicine, genetic therapy, and beyond.
Mutations and Genetic Code Integrity
Mutations play a crucial role in the integrity of the genetic code. They represent variations in the DNA sequence, which can lead to changes in the genotype and potentially affect the phenotype of an organism. Understanding mutations helps clarify how genetic information is maintained, altered, and sometimes disrupted within biological systems. The integrity of the genetic code is essential for proper cellular function, ensuring that organisms develop and evolve effectively.
Types of Mutations
Mutations can be classified into several categories based on their nature and effect:
- Point Mutations: These are the most common type of mutations, involving a change in a single nucleotide. For example, a cytosine may be replaced by thymine.
- Insertions and Deletions: These mutations occur when nucleotides are added or removed from the sequence. Insertions can lead to frame shifts, potentially altering the entire downstream sequence.
- Frameshift Mutations: A subtype of insertion and deletion mutations, frameshift mutations disrupt the reading frame of the gene, which can have profound effects on protein synthesis.
- Repeat Expansions: These mutations occur when a short sequence of DNA is repeated multiple times. Such mutations can lead to disorders, including some forms of muscular dystrophy.
"Mutations are the raw material for evolution; without them, life as we know it would not exist."
Impact on Phenotypes
The relationship between mutations and phenotypes is significant. The change in a nucleotide sequence can lead directly to alterations in the expression and functioning of genes, thus influencing physical traits or behaviors. The effects can be categorized as follows:
- Neutral Mutations: Many mutations do not affect the phenotype. These are often considered neutral as they do not alter the organism's fitness.
- Beneficial Mutations: Occasionally, mutations provide advantages in survival or reproduction. They can lead to beneficial adaptations to environmental changes.
- Harmful Mutations: Some mutations can disrupt normal biological functions. Examples include genetic disorders such as cystic fibrosis or sickle cell anemia, which arise from specific mutations in critical genes.
The implications of mutations on phenotypes underline the significance of genetic code integrity. Both beneficial and harmful mutations are part of the evolutionary process, indicating that while the genetic code must remain stable, it also needs the flexibility to allow for changes that can lead to new traits and adaptations.
Application of Genetic Code in Biotechnology
The application of genetic code in biotechnology represents a groundbreaking shift in how scientists and medical professionals approach biological problems. Understanding the language of genetic code allows researchers to manipulate organisms at the molecular level. This field is rapidly evolving, providing tools and methods that can lead to significant advancements across various industries. The significance of this topic lies not only in its scientific practicality but also in the ethical considerations surrounding these innovations.
Genetic Engineering Techniques
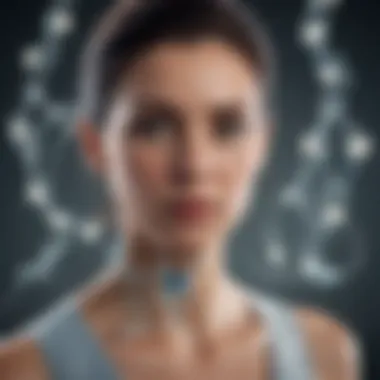
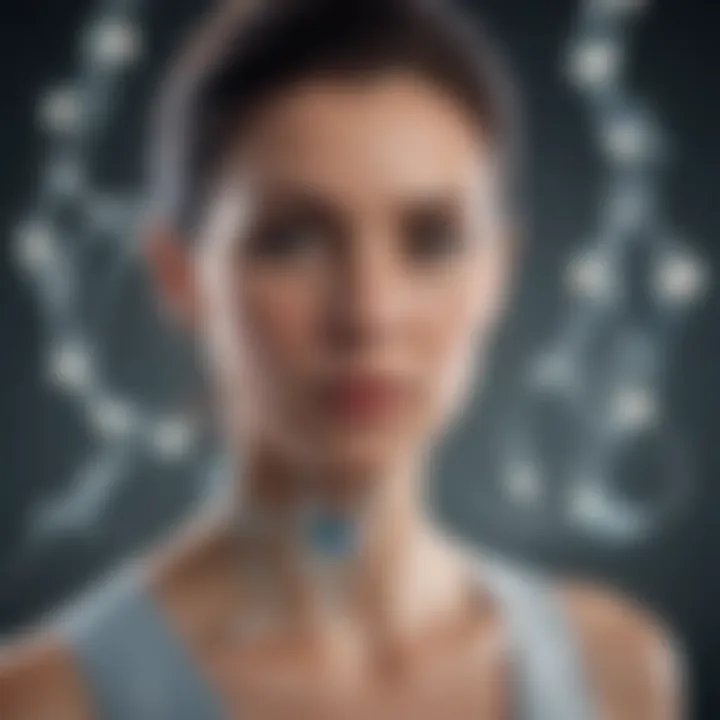
Genetic engineering techniques have revolutionized the possibilities within biotechnology. These methods allow for direct manipulation of an organism's DNA to achieve desired traits. There are several prominent techniques utilized in genetic engineering:
- Recombinant DNA Technology: This process involves combining DNA from different sources to create new genetic combinations. The technology provides the framework for producing insulin, growth hormones, and several vaccines.
- Gene Cloning: This technique allows for the replication of specific genes, enabling the production of proteins and other substances in useful quantities. It is foundational in creating genetically modified organisms (GMOs) that can exhibit traits such as pest resistance.
- Transgenic Organisms: These organisms contain genes from other species. Crops that produce their own pest resistance are examples of this, which highlights the agricultural applications of genetic code manipulation.
These techniques have a vast range of applications including agriculture, medicine, and environmental management. They facilitate the discovery of new treatments for diseases and enable the creation of crops capable of withstanding harsh environmental conditions.
CRISPR Technology
One of the most notable advancements in genetic engineering is CRISPR technology. CRISPR, which stands for Clustered Regularly Interspaced Short Palindromic Repeats, is a revolutionary tool that allows scientists to edit genes with unprecedented precision. It provides a mechanism to cut DNA at specific locations, allowing for targeted modifications.
Benefits of CRISPR include:
- Precision: Researchers can make highly accurate changes to DNA sequences, minimizing off-target effects.
- Efficiency: Compared to traditional methods, CRISPR is faster and less expensive to implement.
- Versatility: It can be used across various organisms, from bacteria to plants and animals, demonstrating its flexibility.
According to recent studies, CRISPR's ability to edit genes not only propels medical research but also raises ethical questions around gene editing in human embryos and related fields.
Despite its promise, CRISPR technology is not without challenges and ethical implications. There are concerns regarding unintended consequences of gene editing, and the potential for misuse of technology raises significant ethical questions. Discussions are ongoing about the regulations and guidelines necessary to navigate the moral terrain of biotechnology squared with human safety and environmental sustainability.
Ethical Considerations in Genetic Research
The exploration of the genetic code extends beyond the scientific implications; it raises significant ethical considerations. Gene editing and genetic manipulation promise advancements in medicine and agriculture but also evoke concerns about the moral implications of altering life itself.
Ethical considerations in genetic resarch are essential to navigating the complex issues that arise from our increasing ability to change genetic material. Engaging with these considerations helps societies to ensure responsible use of powerful techniques. It encourages a balanced assessment of benefits and potential risks associated with genetic manipulations.
Gene Editing Ethics
Gene editing presents myriad ethical dilemmas. The primary concern lies in the potential for unintended consequences. For example, CRISPR technology allows precise modifications to DNA. However, these modifications may lead to unforeseen effects in the genome, possibly impacting not just the individual but future generations as well. Here are some key ethical points:
- Informed Consent: Individuals should understand the risks and benefits of genetic procedures. Consent must be freely given without coercion.
- Equity: Access to gene editing technologies must be equitable. There is a risk of creating disparities between those who can afford treatments and those who cannot.
- Eugenics Concerns: The possibility of using gene editing for "designer babies" raises alarms about societal pressures and ethical boundaries. It leads to questions about what traits are desirable and who decides this.
Implications for Society
The societal implications of genetic research are far-reaching. As science progresses, society faces questions about how to handle genetic information and whose rights it is.
- Privacy: With DNA being more accessible, there are risks related to privacy breaches. Personal genetic data could be misused for discrimination in insurance, employment, or by law enforcement.
- Cultural Perspectives: Different cultures may have varied views regarding genetic modification. Engaging these perspectives is crucial for respectful dialogue.
- Legislation: The speed of advancements in genetic technology often outpaces regulatory frameworks. It is vital that legislation keeps up with these changes to ensure safety and ethical compliance.
These discussions are not merely academic; they are foundational to shaping a future where genetic technologies enhance rather than undermine societal values.
Ultimately, navigating the ethical landscape of genetic research requires collaboration. It involves scientists, ethicists, policymakers, and the public. Only then can we harness the power of genetic code responsibly, ensuring that its benefits are shared by all.
Future Directions in Genetic Research
Future directions in genetic research hold significant promise for the development of innovative solutions in both medicine and agriculture. Continued advancements could reshape our understanding of genetics and its role in health and disease. These explorations could lead to breakthroughs in treating genetic disorders, improving crop resilience, and even combating climate change through genetic modifications.
Advancements in Understanding
Recent technological innovations have transformed the methods used in genetic research. Techniques like high-throughput sequencing allow researchers to quickly decode genetic information from various organisms. This capability has opened new paths for studying genetic variations and their manifestations in different species. For example, employing single-cell RNA sequencing aids in uncovering the complexities of gene expression at a cellular level. Understanding these variations is essential for tailoring personalized medicine strategies, which consider individual genetic backgrounds.
Moreover, computational advancements enable researchers to analyze vast datasets efficiently, detecting patterns and forecasting trends that were previously unattainable. Bioinformatics tools assist in visualizing complex genetic interactions, aiding scientists in developing more precise hypotheses. The potential of these advancements lies in their ability to bridge gaps between theoretical genetics and practical applications in medicine and agriculture.
Potential Discoveries
The landscape of genetic research is ripe with possibilities for future discoveries. One area garnering attention involves gene editing technologies, particularly CRISPR. As researchers become more adept at editing genes with precision, they will likely uncover novel methodologies to combat diseases like sickle cell anemia and certain types of cancer.
Additionally, understanding epigenetics may yield insights into how environmental factors influence gene expression. This understanding can pave the way for preventive strategies in healthcare, addressing conditions that arise from lifestyle and environmental exposures, rather than solely genetic predispositions.
Furthermore, exploration of the microbiome is proving vital. The interplay between our genes and the trillions of microorganisms living within us can illuminate pathways to better health, informing dietary recommendations and probiotic use. Unlocking these relationships can significantly affect how we approach treatments for various conditions.
"The future of genetic research is not just in understanding what genes do, but in harnessing this knowledge to improve human life and the environment."
Culmination
The conclusion serves as a capstone to the intricate exploration undertaken in this article. It encapsulates the breadth of information surrounding the genetic code, emphasizing its significance in biological sciences and its implications for various fields, including medicine, biotechnology, and evolutionary studies.
In this article, we have uncovered the fundamental components of genetic coding, explained the processes of transcription and translation, and discussed the mutations that can affect genetic integrity. These elements outline not only how life operates at a molecular level but also how this knowledge impacts human health and technological advancements.
Summary of Key Points
- The genetic code is the essential framework governing the synthesis of proteins in living organisms.
- Understanding the structure of DNA is crucial as it reveals how genetic information is stored and utilized.
- The role of codons, amino acids, and key processes like transcription and translation are central to gene expression.
- We discussed the variability of the genetic code, acknowledging both its universality and exceptions.
- Mutations can lead to changes in phenotype, underlining the importance of genetic integrity.
- Modern biotechnology has harnessed this knowledge, paving the way for innovations like CRISPR.
- Ethical considerations remind us that with great power comes great responsibility in genetic research.
- The future holds promise for continued exploration and understanding of the genetic code, leading to potential discoveries that can transform our approach to health and disease.
Final Thoughts on Genetic Code
The genetic code serves as an essential organizing principle of life, imparting vast knowledge about the workings of organisms. Its study has transformed our understanding of biological processes and has opened new avenues in genetic research. As science progresses, our comprehension of the genetic code will continue to refine, enhancing our capabilities in medicine, agriculture, and beyond. Thus, understanding this blueprint is not only an academic endeavor but also a vital stepping stone towards improving life on Earth and addressing some of humanity's greatest challenges.