High Frequency Inductors: Fundamentals and Applications
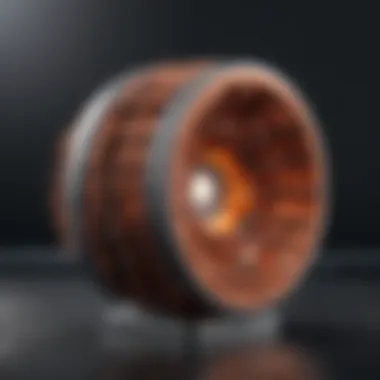
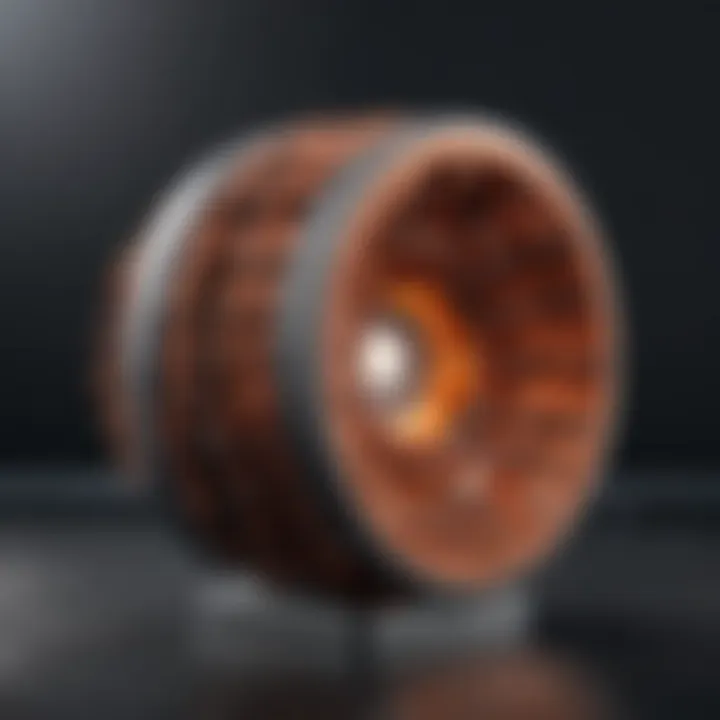
Intro
High frequency inductors play a crucial role in modern electronic circuits and devices. These components are critical in filtering, energy storage, and signal processing. Their behavior and efficiency become significantly different when operating at elevated frequencies, requiring a unique understanding of their properties and design considerations. This article aims to provide a comprehensive overview of high frequency inductors, covering their construction, functionality, and applications.
Research Overview
Understanding the role of high frequency inductors requires a closer examination of the methodologies applied in their exploration. Researchers often adopt diverse approaches to analyze and evaluate inductors' characteristics at high frequencies, which include theoretical models, simulations, and experimental setups. The blend of these methodologies allows for a well-rounded understanding of how inductors function in various applications.
Methodological Approaches
- Simulation Techniques: Advanced software programs like Ansys HFSS or COMSOL Multiphysics are frequently used to model and simulate inductor behavior under different conditions.
- Experimental Validation: Actual prototypes are fabricated and tested to gather empirical data. Measurements can include inductance, quality factor, and thermal performance.
- Theoretical Analysis: Theoretical frameworks are developed to predict inductor performance, taking into consideration factors like skin effect and proximity effect at high frequencies.
Significance and Implications
The significance of high frequency inductors cannot be overstated. These components are foundational in technologies such as RF (Radio Frequency) amplifiers, switch-mode power supplies, and telecommunications. As technology advances, the demand for more efficient and compact inductors grows. Thus, understanding their behavior aids in the design of better electronic devices, improving performance while reducing size and cost.
Current Trends in Science
In recent years, advances in materials science and fabrication techniques have begun to reshape how high frequency inductors are designed and utilized.
Innovative Techniques and Tools
The integration of new materials can lead to enhanced performance. For instance, the use of ferrite cores, which have high permeability, improves inductance while minimizing losses. Additionally, multilayer constructions provide a compact design, enabling the fabrication of surface mount inductors suitable for modern applications.
Interdisciplinary Connections
The field of high frequency inductors cannot be divorced from other scientific disciplines. Innovations in materials science, electrical engineering, and physics continually inform the design and application of inductors. For example, insights from nanotechnology lead to the development of inductors with improved efficiency and reduced size. Such interdisciplinary collaboration is crucial for pushing the boundaries of what is achievable in high frequency applications.
"High frequency inductors are not just components; they are pivotal in the evolution of electronic systems, making them integral to just about any modern electronic device."
Epilogue
High frequency inductors are essential components, influencing the performance of numerous electronic devices. By understanding their construction, methodologies, and applications, one can appreciate the significance they hold in our technology-driven world.
Prelude to Inductance
Inductance is a crucial concept in electrical engineering and physics. It describes the property of an electrical conductor to store energy in a magnetic field when an electric current passes through it. This principle underlies many essential technologies, particularly in high-frequency applications such as communication systems and power devices. Understanding inductance enables engineers and designers to optimize circuit functionality and performance.
The importance of inductance can be highlighted in various ways. First, it is essential for analyzing circuit behavior, especially in alternating current situations. Reactance, which includes capacitance and inductance, must be fully understood to design effective and efficient circuits. Moreover, inductance plays a significant role in energy transfer processes, which is vital in the stability of power systems.
Moreover, the rise of modern technologies relies on inductive components. High frequency inductors are critical in filtering applications, energy storage, and magnetic devices. They ensure signal integrity and contribute to the overall efficiency of electronic systems. Therefore, this article delves deeper into inductance, exploring its characteristics and implications while emphasizing its relevance in contemporary engineering and technology.
Defining Inductance
Inductance is defined as the ratio of the induced electromotive force (emf) to the rate of change of electric current that produces it. This property can be quantified in henries, symbolized by the letter L. A higher inductance value indicates a greater ability to store energy in the magnetic field. Two primary factors influence inductance: the number of turns in a coil and the type of core material used.
In practical applications, inductance influences circuit behavior at various frequencies. As inductance increases, the coil can store more energy, but this can also lead to higher reactance at higher frequencies, affecting the circuit's overall performance. Understanding this relationship helps designers avoid undesirable effects while optimizing component selection and placement.
The Concept of Reactance
Reactance is an essential aspect of AC circuits, representing the opposition to the flow of current caused by inductance and capacitance. Inductive reactance specifically describes how an inductor resists changes in current. It is directly proportional to the frequency of the current and the inductance of the coil, represented by the formula:
[ X_L = 2\pi f L ]
where (X_L) is the inductive reactance, (f) is the frequency, and (L) is the inductance. As frequency increases, inductive reactance also increases, influencing circuit performance significantly.
In many applications, managing reactance is crucial for maintaining signal integrity. For instance, in radio frequency (RF) and microwave circuits, the care in shaping reactance helps improve transmission quality and ensures minimal signal loss. Understanding the concept of reactance is, therefore, vital in high frequency inductor application, as it informs many design choices.
Characteristics of High Frequency Inductors
Understanding the characteristics of high frequency inductors is essential for harnessing their full potential in various applications. These components play a critical role in shaping the response of electrical circuits under high frequency conditions. Key elements such as frequency response, impedance, and potential losses are paramount when analyzing these inductors.
Frequency Response
Frequency response refers to the behavior of an inductor as it reacts to different frequencies of electrical signals. High frequency inductors are designed to operate efficiently in the megahertz to gigahertz range. This response emphasizes how the inductor's reactance increases with frequency. A fundamental point is that the inductance value can determine how effectively the component reduces high frequency noise in circuits.
Practical implications arise when choosing inductors for specific applications. For instance, inductors with higher self-resonant frequencies will perform better in RF (Radio Frequency) applications, providing a flatter response across varying frequencies.
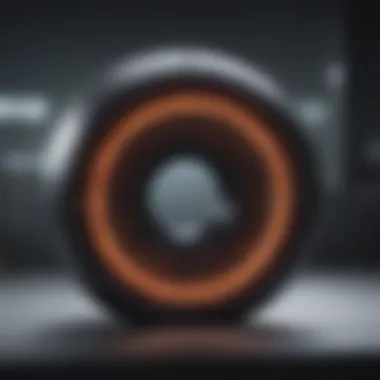
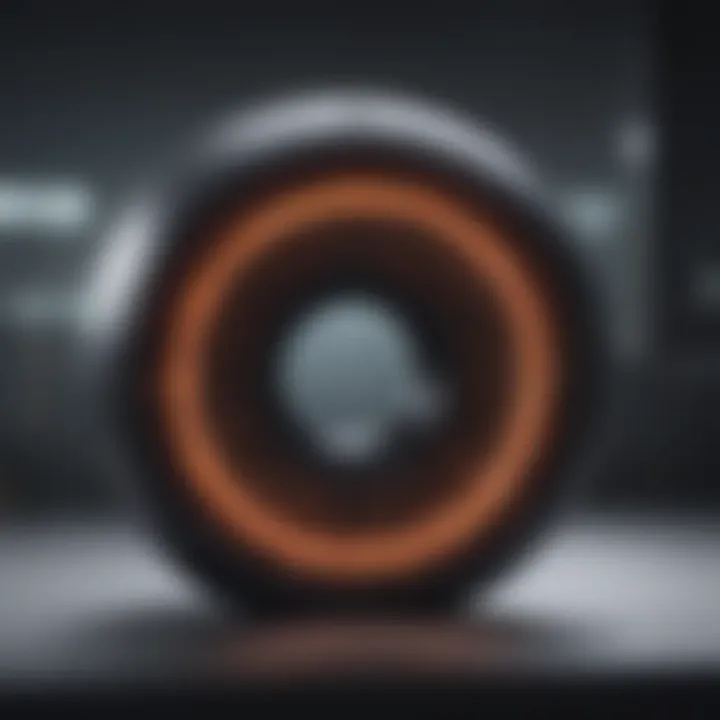
Key considerations include:
- Self-Resonant Frequency: This is the frequency at which the inductance and capacitance of the inductor resonate. Beyond this frequency, the inductor behaves differently.
- Quality Factor (Q): This indicates the efficiency of the inductor. A higher Q value means less energy loss and better performance at high frequencies.
Impedance and Losses
The impedance of a high frequency inductor denotes how much it resists the flow of alternating current. It is influenced by both the inductive reactance and any inherent resistance within the coil. A low impedance is favorable for high frequency applications, as it allows for more efficient energy transfer.
However, losses can occur in high frequency inductors, and they can significantly impact performance. Two main types of losses are associated with high frequency inductors:
- Copper Loss: This loss occurs due to the resistance of the wire used in the winding. As the frequency increases, the skin effect exacerbates this issue, as current tends to flow near the surface of the conductor.
- Core Loss: Relating to the magnetic core material, core loss can stem from hysteresis and eddy currents. Selection of materials that minimize these losses is crucial in high frequency designs.
"High frequency inductors must balance impedance and losses for optimal performance in demanding applications."
Types of High Frequency Inductors
Understanding the various types of high frequency inductors is crucial in both academic and practical applications. Each type presents unique advantages and limitations based on their construction and material properties. This section explores three primary categories of high frequency inductors: air core, ferrite core, and steel core inductors. The insights into these types will help in selecting the most appropriate inductor for specific functions and performance requirements.
Air Core Inductors
Air core inductors, as the name suggests, utilize air as the core material. This design primarily affects the inductance value and the inductor’s behavior at high frequencies. One of the main advantages of air core inductors is their low core losses, which is vital in high frequency applications. Because there are no ferromagnetic materials, these inductors can operate without introducing additional losses due to saturation.
In air core inductors, the winding is usually tightly coiled to maximize the magnetic field. This simplicity in construction allows for ease in design and customization. However, it is important to note that air core inductors generally have lower inductance values compared to their ferrite and steel counterparts. For applications requiring high inductance, users may need to increase the number of turns, which, in turn, can affect size and resistance. Therefore, one must balance between size, resistance, and inductance when choosing air core inductors.
Ferrite Core Inductors
Ferrite core inductors incorporate ferrite materials which are ceramic compounds that exhibit magnetic properties. They are favored in applications that demand higher inductance values. Ferrite cores are excellent at preventing losses associated with magnetic saturation, and they often provide higher permeability than air. This results in greater inductance in a more compact size compared to air core inductors.
Using ferrite cores allows for more efficient energy transfer, making these inductors suitable for RF applications and power supplies. However, one must consider that ferrite materials can limit the frequency response due to core losses, especially at very high frequencies. Additionally, the temperature coefficient of ferrite may lead to changes in inductance values under varying conditions, requiring designers to account for thermal effects when implementing these inductors in circuits.
Steel Core Inductors
Steel core inductors utilize laminated or solid steel cores to enhance inductance. Similar to ferrite inductors, steel maintains a high magnetic permeability, which allows for elevated inductance in a limited physical space. These inductors are often employed in power applications where endurance against thermal fluctuations is crucial.
One of the challenges with steel core inductors, however, is the increased risk of core saturation, particularly under high current conditions. Core losses can also accumulate due to hysteresis and eddy currents, which are significant in high frequency environments. Careful design and material selection are essential to mitigate these issues. While steel core inductors are robust, their performance can diminish if not properly designed for their intended frequency range.
"Selecting the right type of inductor is paramount to achieving desired performance in electronic circuits."
In summary, choosing between air core, ferrite core, and steel core inductors involves understanding their specific characteristics and application contexts. Each type has benefits and consideration factors, which should align with the overall goals of the electronic design.
Construction and Design Principles
The construction and design principles of high frequency inductors form the backbone of their functionality and effectiveness in applications. These principles are vital for optimizing performance, minimizing losses, and ensuring reliability under various operating conditions. A comprehensive understanding of the elements involved in the design process helps engineers and researchers make informed decisions when developing or selecting inductors for specific uses.
Winding Techniques
Winding techniques directly influence the characteristics of high frequency inductors. The method of winding affects the inductor's inductance value, resistance, and overall quality factor. There are generally two main winding methods: the circular winding and the helical winding. Circular winding is often preferred for its ability to provide uniform magnetic fields, while helical winding tends to be more compact. The choice of technique largely depends on size constraints and the frequency of operation.
Proper winding also seeks to reduce parasitic capacitance, which can degrade performance at high frequencies. Techniques such as bifilar winding and Litz wire winding are employed to combat these issues. Bifilar winding uses two wires twisted together, which can significantly reduce the magnetic field losses. Litz wire is composed of many very thin insulated wires bundled together. This structure minimizes skin effect and improves high-frequency performance.
Selection of Core Materials
Core materials play a critical role in the performance of high frequency inductors. Different materials, such as ferrite, iron powder, and air, provide varied magnetic properties and efficiencies. The selection of core materials significantly impacts the inductor's inductance value, magnetic saturation, and losses.
Ferrite cores are the most commonly used in high frequency applications due to their high magnetic permeability and low eddy current loss. They are effective at reducing core losses significantly, especially in RF applications. Iron powder is another material option which offers good performance in specific configurations. The trade-off exists between core loss and structural loss, pushing designers to choose the right material carefully based on application needs.
Dielectric Considerations
Dielectric materials are significant in the construction of high frequency inductors. They determine the insulation properties and can influence the inductive components' overall performance at high frequencies. Factors such as dielectric strength, dissipation factor, and temperature stability are paramount in the selection process.
When constructing inductors, dielectric materials should be selected to ensure minimal loss at operating frequencies. Polyimide and PTFE are commonly used to achieve high performance over a range of frequencies. A well-chosen dielectric will help maintain stability and efficiency while minimizing unwanted resonances and losses during operation.
In summary, careful attention to winding techniques, core material selection, and dielectric considerations is essential for the construction and design of high frequency inductors. Understanding these principles enables the development of inductors that meet the performance demands of modern electronic applications.
Functional Applications of High Frequency Inductors
High frequency inductors are integral components in various electronic systems. Their functional applications range across multiple domains, influencing the efficiency and performance of power supplies, RF applications, and filtering operations. Understanding these applications not only reflects their importance but also elucidates how they enable technological advancements. This discussion aims to provide clarity on the specific roles high frequency inductors play, highlighting their benefits and considerations in design and application.
In Power Supplies
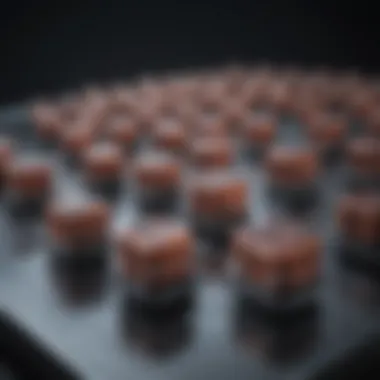
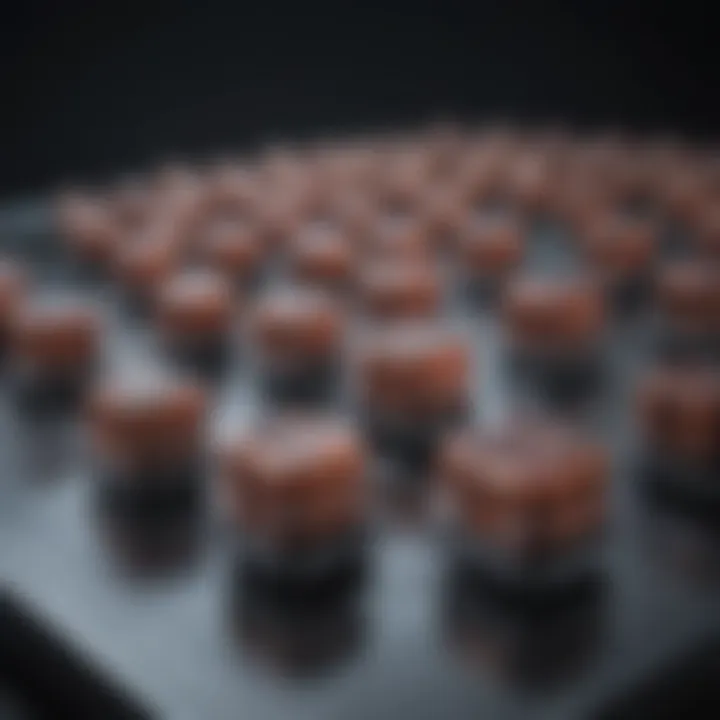
High frequency inductors play a crucial role in power supply circuits. They are frequently used in switch-mode power supplies (SMPS). In these applications, inductors are responsible for energy storage, managing current flow, and helping minimize losses. They contribute to better energy efficiency by ensuring that energy is transferred smoothly during the conversion process.
Additionally, inductors allow for voltage regulation by controlling the path of electrical current and reducing electromagnetic interference. This is especially important in high-frequency environments where noise can distort signals and degrade performance.
Some key advantages of using high frequency inductors in power supplies include:
- Compact Design: Their small size helps in making systems more compact and efficient.
- Weight Reduction: Minimizes the overall weight of the electronic system, which is vital in applications like portable devices.
- Scalability: Allows for scaling the power supply circuit for various load requirements without significant redesign.
In RF Applications
In RF applications, high frequency inductors are essential for controlling and handling RF signals. They are widely used in antennas, transmitters, and RF filters. The inductors ensure that signals are transmitted and received with minimal loss and distortion. In particular, they help to achieve matching impedance, which is critical for optimal energy transfer and device performance.
These inductors can also be used in tuning circuits where adjusting the inductance can change the frequency response, thus enhancing signal clarity. This becomes particularly significant in communication technologies, radar systems, and broadcasting, where precision is crucial.
Benefits include:
- Strong Performance: Enhances signal integrity and minimizes loss.
- Frequency Selectivity: Enables tuning to specific frequency ranges, improving device function.
- Resistance to Harmonics: Helps to suppress unwanted harmonic signals, which can interfere with primary frequencies.
In Filters and Oscillators
High frequency inductors are widely used in designing filters and oscillators. Filters that utilize inductors can selectively pass certain frequencies while blocking others, which is essential in signal processing. These inductors form part of LC circuits, where they work in conjunction with capacitors to achieve desired filtering effects.
Oscillators utilize high frequency inductors to generate stable frequency signals. In these settings, the inductors determine the oscillation frequency by working with capacitors as resonant circuits. Achieving an accurate frequency is vital for applications such as clock generation and sound synthesis.
The advantages include:
- Customization: Allows for design of filters for specific applications, enhancing overall performance.
- Stability: In oscillators, contributes to signal stability and frequency precision.
- Robustness: High quality inductors offer performance reliability in rigorous environments.
"The role of high frequency inductors expands across various applications, shaping the very foundation of modern electronic systems."
Modeling and Simulation
Modeling and simulation play crucial roles in understanding and analyzing high frequency inductors. These methodologies allow engineers and researchers to predict inductor behavior under various conditions, providing insights that are critical for design optimization. With high frequency applications, the behavior of inductors can be complex, influenced by numerous factors such as core materials and winding techniques. Thus, proficient modeling helps to visualize electromagnetic performance and potential losses in an efficient manner.
The primary benefit of leveraging modeling techniques lies in their ability to reduce development time. Instead of relying solely on physical prototypes, simulations can point out deficiencies in design early in the process. Both circuit modeling and numerical simulation contribute to achieving accurate assessments of inductors, minimizing costs and maximizing performance in real-world applications.
It is also important to consider the integration of these modeling techniques with design software. This compatibility ensures that simulations reflect practical situations closely. High frequency inductors often operate in intricate environments. The demand for precision in these simulations cannot be understated. Multiple iterations might be required to refine a model, ensuring it aligns closely with experimental data.
"Through modeling, one can significantly reduce prototype testing time and identify key areas of improvement more efficiently."
Circuit Modeling Techniques
Circuit modeling techniques for high frequency inductors typically involve both analytical and simulation methods. Engineers may use software such as SPICE or MATLAB to develop models that predict inductor behavior. A common approach is to use equivalent circuit representations, which simplify the inductance into components such as resistors, capacitors, and inductors.
This representation helps to assess how different components interact at high frequencies. For instance, the series resistance and parasitic capacitance must be accurately modeled, as they can significantly impact performance. By employing these techniques, designers can evaluate transient responses and frequency responses effectively.
Another aspect is the use of parametric modeling, where varying certain parameters can help understand sensitivity and stability of the design. Adjusting factors like core material properties or winding configurations allows for exploring a broader range of possible performance scenarios.
Numerical Simulation Tools
Numerical simulation tools are indispensable for analyzing high frequency inductors. Software such as ANSYS HFSS and COMSOL Multiphysics are widely used to conduct electromagnetic simulations. These programs help visualize field distribution and current flow within inductors.
Finite Element Method (FEM) is commonly employed in these tools, allowing for precise modeling of complex geometries. This technique computes electromagnetic fields in inductor cores, leading to an understanding of how design modifications affect performance.
Additionally, statistical simulation can identify uncertainties in materials and component interactions, offering a broader perspective on how robust the design is against variations in manufacturing. By combining these numerical simulation tools with circuit modeling, one can attain a comprehensive view of an inductor's behavior across frequency ranges.
In summary, both circuit modeling techniques and numerical simulation tools are vital in advancing the design and application of high frequency inductors. They promote a better understanding of complex behaviors, ultimately leading to more efficient and reliable inductor designs.
Challenges in High Frequency Inductor Design
Designing high frequency inductors involves navigating a variety of complex challenges. These issues must be understood and addressed for achieving optimal performance in electronic applications. The design process must balance numerous factors, including efficiency, thermal management, and electromagnetic compatibility. As technology evolves and operating frequencies increase, the importance of these challenges only intensifies in a landscape where precision and reliability are paramount.
Thermal Issues
Heat generation is a critical concern in high frequency inductor design. When inductors operate at elevated frequencies, they can produce significant amounts of heat due to core losses, winding resistances, and other factors. This heat can lead to several problems, including:
- Reduced efficiency: Elevated temperatures negatively impact the inductor’s performance, particularly in terms of power loss.
- Core degradation: Prolonged exposure to high temperatures can cause materials to break down or lose their magnetic properties.
- Component failure: Overheating can lead to failure of the inductor or other components within the circuit, potentially compromising the entire system.
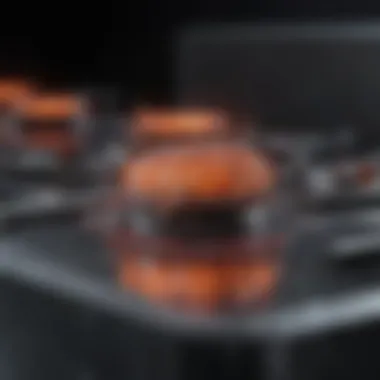
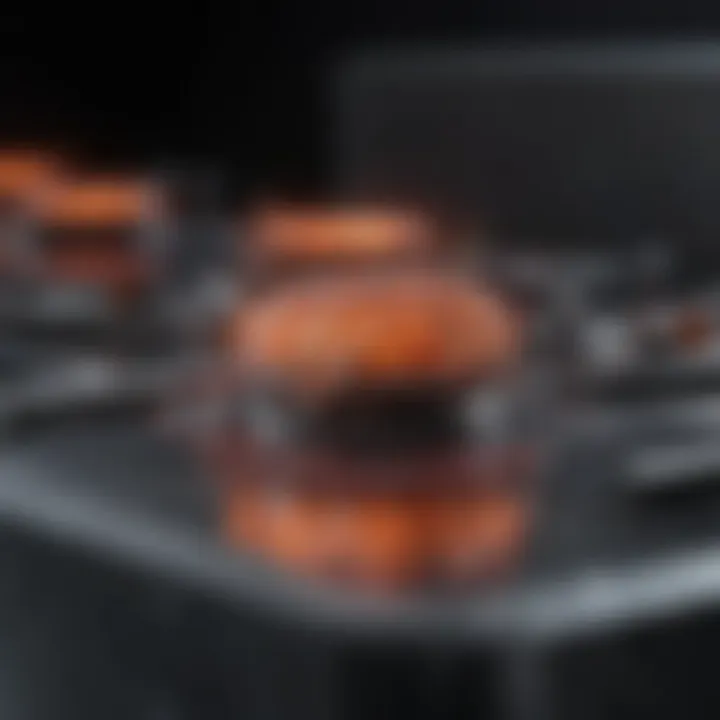
Effective thermal management strategies are thus necessary. Solutions often involve selecting appropriate materials with high thermal conductivity, utilizing heatsinks, or designing the circuit layout for optimal airflow. Attention to these details can greatly enhance both reliability and lifespan of inductor assemblies.
Magnetic Interference
Magnetic interference presents another significant challenge in high frequency inductor design. At higher frequencies, inductors can generate strong electromagnetic fields which may affect adjacent components or circuits. Issues include:
- Crosstalk: Unwanted coupling between inductors and nearby circuits can lead to signal integrity problems, influencing the overall functionality of the device.
- Performance degradation: Magnetic interference can distort the operating characteristics of sensitive components, such as RF transistors or amplifiers.
- Design constraints: Managing magnetic interference often requires thoughtful layout designs and additional shielding, which can complicate and increase the cost of the design process.
To mitigate these concerns, engineers typically use techniques such as proper circuit layout, incorporating ferrite materials, or employing shielding technologies. Understanding these aspects of magnetic interference can lead to more robust designs and reliable operation in high frequency applications.
In summary, addressing thermal issues and magnetic interference is essential for the successful design of high frequency inductors. As technologies advance, engineers must continually refine their approaches to ensure the inductor’s performance meets rising demands.
Future Trends in Inductor Technology
The future of inductor technology is shaped by the rapid advancements in materials and manufacturing techniques. As electronic devices become more compact and performance-oriented, there is a pressing need for inductors that can deliver superior efficiency and reliability in high-frequency applications. This section will discuss key trends that are expected to redefine the role of inductors in modern electronics.
Innovations in Materials
Recent developments in materials used for inductors have prompted notable improvements in their efficiency and effectiveness. Traditional wire and core materials are increasingly supplemented with newer compounds that enhance performance characteristics. For instance, polymer-based materials are being explored for their low-loss characteristics. These materials reduce energy depletion at high frequencies, thus making inductors more efficient.
Moreover, advanced ferromagnetic materials, such as nanocrystalline alloys, offer improved magnetic properties that can lead to smaller and lighter designs without compromising performance. As industries push toward high power density solutions, the innovative application of these materials will likely become common.
Some aspects of the materials innovation trend include:
- Improved electrical conductivity resulting in lower heat generation.
- Higher saturation flux densities, allowing for compact designs.
- Resistance to environmental factors, leading to longer product life cycles.
These innovations do not just aim to meet current needs but also anticipate future demands, such as the merging of inductor designs with other components for multifunctional systems.
Advancements in Manufacturing Methods
As the demand for high-frequency inductors increases, so too does the need for advanced manufacturing techniques. Automation and precision engineering are leading the way in making inductor production more efficient and cost-effective.
New methods such as additive manufacturing, commonly known as 3D printing, are gaining traction. This approach allows for greater freedom in design complexity, enabling intricate geometries that were not possible with traditional manufacturing methods. It not only accelerates prototyping but also allows for low-volume production runs with reduced waste.
On the assembly side, robotic technologies are being utilized to ensure quality and consistency. Automation facilitates the creation of inductors with tighter tolerances and promotes higher production speeds with minimized human error.
Important advancements in manufacturing include:
- 3D printing for enhanced design flexibility.
- Precision winding techniques to improve coil structure and reliability.
- Smart manufacturing systems, incorporating IoT for real-time production monitoring.
Through these innovations, the inductor manufacturing landscape is evolving, aiming to create highly efficient components that can adapt to the needs of next-generation electronic systems.
"The evolution in inductor technology reflects the dynamic nature of electronic systems, where every improvement can lead to significant performance gains."
In summary, understanding these future trends in inductor technology not only prepares industries for upcoming challenges but also encourages informed decisions on material selection and manufacturing processes.
Finale
As we conclude this exploration of high frequency inductors, it is clear that these components play a crucial role in modern electronics. Their ability to manage signals and power efficiently makes them indispensable in various applications. Understanding their design and functionality allows engineers and designers to implement them effectively in their projects.
Summing Up the Importance
High frequency inductors are essential not only in power supplies but also in communication technologies and filtering systems. Their unique characteristics enable them to perform at elevated frequencies, where traditional inductors may fail. This adaptability underscores their significance in a rapidly evolving technological landscape. In various applications, these inductors help reduce noise, improve signal integrity, and enhance performance. Consequently, the importance of incorporating high frequency inductors into electronic designs can hardly be overstated.
Implications for Future Research
The ongoing evolution of materials and manufacturing processes presents both challenges and opportunities for future research in high frequency inductors. Researchers should investigate new core materials that may offer improved performance characteristics. Additionally, advancements in fabrication techniques could lead to more compact designs with enhanced capabilities. Exploring these areas will not only contribute to the understanding of inductance at high frequencies but also innovate in applications that depend on reliable performance.
"Investigation into high frequency inductors may lead to breakthroughs that enhance our technological infrastructure and capabilities."
The implications for advancing research in this field are vast, encouraging a collaborative effort among academic institutions, industry, and independent researchers to explore these critical components further.
Importance of References
References are crucial for several reasons:
- Validation of Information: Citing established texts and research articles lends authority to the claims made in the article. This is particularly important in technical fields like electronics, where the reliability of information can affect design decisions and applications.
- Further Readings: Good references often lead to further exploration. They guide readers to discover more about specific topics, helping to build a comprehensive understanding of high frequency inductors and their roles in various circuits.
- Historical Context: Understanding the evolution of inductors through referenced studies offers insight into how current technologies evolved. It also helps researchers appreciate the challenges faced historically, which can inform current practices.
- Technical Details: Many references provide detailed technical specifications and methodologies that reinforce the concepts discussed in this article. This helps readers grasp complex ideas, such as the nuances of reactance and impedance in high frequency scenarios.
Considerations about References
While utilizing references, it’s important to evaluate their credibility:
- Authorship: Consider who wrote the material. Are they recognized experts in the field of electrical engineering or a related discipline?
- Publication Date: Given the rapid advancements in technology, reference materials should be recent enough to ensure relevance.
- Publisher Reputation: Reputable publishers and journals have a process of peer review, which can enhance the reliability of the information presented.
- Cross-referencing: It is beneficial to compare multiple sources to ensure consistency and accuracy in the information received.
"In technical fields, rigorous referencing is not just a best practice; it is a necessity to drive innovation and ensure safety in applications."