High Pressure Freezing: Key Insights into Cryopreservation

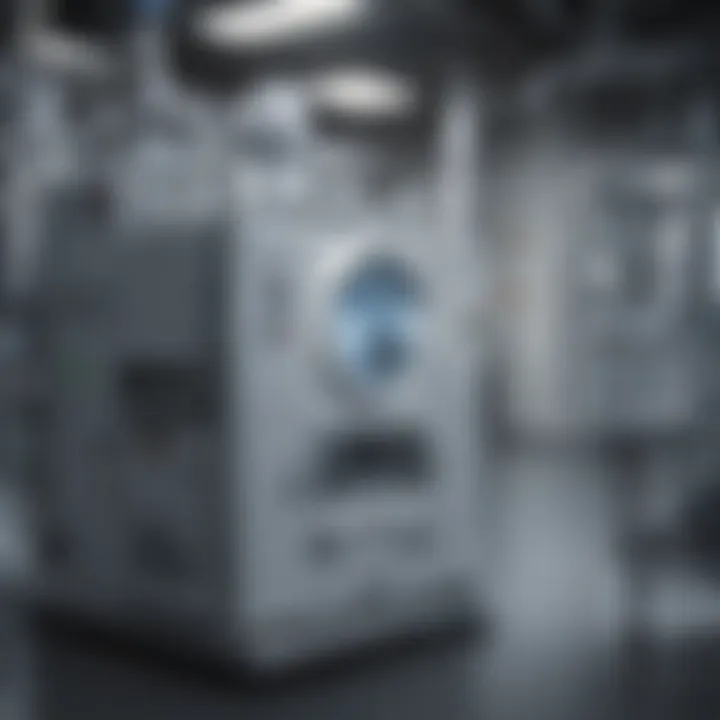
Intro
High pressure freezing (HPF) represents a transformative approach in the field of cryopreservation, merging the principles of physics and biology to deliver vital advancements in research methodologies. This technique allows researchers to preserve biological samples with unprecedented fidelity, effectively mitigating damage caused by ice crystal formation during traditional freezing methods. As biological materials face dramatic changes when subjected to low temperatures, HPF has emerged as an essential tool in various research fields, ranging from cellular biology to materials science.
Research Overview
Methodological Approaches
High pressure freezing operates on the principle of applying immense pressure to specimens before subjecting them to rapid cooling. This process enables the formation of a glass-like state known as vitrification, which prevents the formation of ice crystals. The methodological framework of HPF encompasses:
- Pre-treatment of samples to optimize preservation quality.
- Utilization of high pressure freezes which can reach pressures of up to 2100 bar (around 30,000 psi).
- Immediate transfer of specimens into liquid nitrogen or another cryogenic medium after the pressure is released.
These steps facilitate retention of native structures and biochemical activities, critical for accurate analysis. This approach is precise, allowing scientists to examine intricate cellular and subcellular components in their natural state, offering deeper insights into functionality at cryogenic temperatures.
Significance and Implications
The implications of high pressure freezing are vast. By avoiding ice crystal damage, researchers can conduct studies that were previously unattainable without HPF. Areas significantly impacted by this technology include:
- Cellular Research: Improved understanding of cell structure and dynamics at cryogenic temperatures.
- Cryobiology: Enhanced techniques for preserving biological specimens for transplantation.
- Nanomaterials Science: Insights into material stability and properties in low temperature conditions.
In essence, HPF is not merely a technique but a paradigm shift in how researchers approach the preservation of biological materials.
Current Trends in Science
Innovative Techniques and Tools
The landscape of high pressure freezing is evolving. New methodologies and tools aimed at increasing efficiency and accuracy are emerging frequently. Recent advancements include:
- Development of automated high pressure freezers that improve reproducibility.
- Integrative software systems for real-time monitoring of freezing and thawing processes.
These innovations not only simplify procedures but also expand the potential applications of HPF in various scientific disciplines.
Interdisciplinary Connections
HPF bridges several fields as it intertwines biology with physics and engineering. Its applications are being explored in:
- Biomedical Engineering: For improving the storage of biomaterials and organs.
- Food Science: Addressing cryopreservation techniques for better food product longevity.
The interdisciplinary nature of HPF continues to facilitate collaborations among scientists, promoting shared knowledge and diverse applications. This rich synergy underscores the importance of high pressure freezing in advancing scientific methodologies across disciplines.
High pressure freezing is a crucial innovation in the landscape of scientific research and has transformed how we approach cryopreservation of biological systems.
As we progress further into a data-driven future, understanding the intricacies and advantages of high pressure freezing will be essential for researchers, educators, and professionals aiming to exploit its full potential in their respective fields.
Prologue to High Pressure Freezing
High pressure freezing (HPF) represents a significant advancement in the methodologies used for cryopreservation. As scientists strive for more effective ways to preserve biological and material samples, HPF provides a promising solution that minimizes damage typically associated with traditional freezing methods. The rapid freezing accomplished through HPF is critical for maintaining the integrity of cellular structures and avoiding the formation of ice crystals that can disrupt essential cellular systems.
The importance of this technique extends beyond just preservation; it also impacts areas including cell biology, material science, and food safety. By employing high pressure, samples can achieve a frozen state instantly, thus retaining their native characteristics and functionality. This method is particularly advantageous for sensitive biological specimens, such as proteins and organelles, which can succumb to alterations induced by ice crystal formation during conventional freezing processes.
Definition and Overview
High pressure freezing is a method that involves applying extreme pressure to samples prior to their freezing. This technique alters the way water behaves when it transitions from liquid to solid states. By elevating the pressure, the freezing point of water changes, allowing for faster freezing and leading to less structural disruption. In essence, the method bypasses the slow crystallization of water, which is a challenge in traditional freezing. The result is that cells, tissues, and other materials retain their original architecture with minimal artifacts introduced during the freezing process.
HPF is often employed with the aid of specialized equipment, which ensures controlled pressure application and rapid cooling mechanisms. This technological integration is essential to achieve the desired outcomes at the molecular level.
Historical Development of High Pressure Techniques
The evolution of high pressure techniques has developed through interdisciplinary research, combining principles from physics, chemistry, and biology. Initial explorations into high pressure applications began in the early 20th century, motivated by the desire to understand the behavior of materials under extreme conditions. Over time, scientists began to recognize the potential relevance of these principles in biological contexts.
The mid-20th century marked a crucial period when advances in cryobiology prompted further investigation into . From pioneering studies that demonstrated the effects of high pressure on cellular integrity, researchers gradually refined techniques and protocols to enhance preservation outcomes. The systematic development of high pressure apparatus in laboratories laid groundwork for establishing HPF as a standard method in cryopreservation practices. Today, this technique continues to evolve, driven by ongoing research and technological advancements in both life sciences and materials engineering.
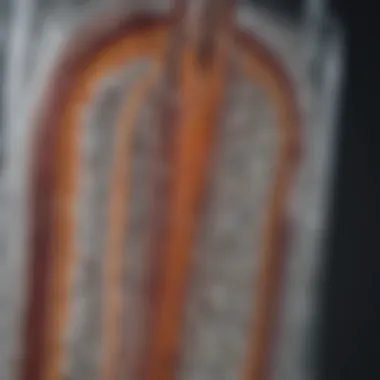
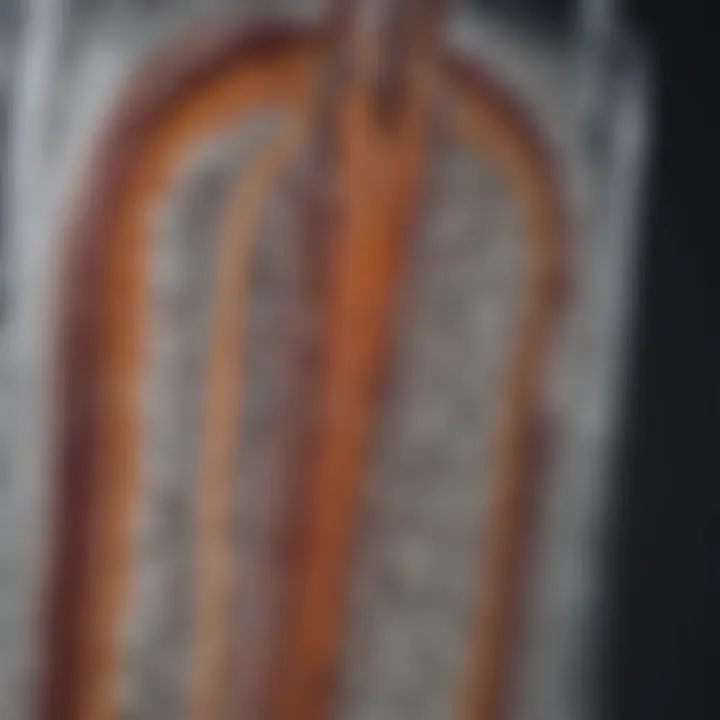
Fundamental Principles of High Pressure Freezing
High pressure freezing (HPF) is grounded in key scientific principles that differentiate it from traditional freezing methods. Understanding these fundamental principles helps elucidate the technique's advantages, especially in preserving biological samples and materials. The significance of these principles lies in the ability of HPF to retain native structures, which is crucial for various applications in research. This section will delve into the thermodynamics of freezing and the concept of phase transitions under extreme pressure, emphasizing their relevance in cryopreservation methodologies.
Thermodynamics of Freezing
The thermodynamic framework of freezing is essential to comprehend how high pressure influences the freezing process. In simple terms, thermodynamics focuses on how energy is transferred during phase changes, particularly from liquid to solid states. Under standard pressure, ice crystals form as water freezes. These ice crystals can physically disrupt the cellular structures, leading to damage in biological specimens and other materials.
However, when high pressure is applied during the freezing process, the phase transition dynamics change. Increased pressure alters the freezing point of water, allowing it to remain in a liquid state at lower temperatures. Consequently, this results in a rapid freezing process that minimizes the time frame for ice crystal formation. Retaining smaller, more evenly distributed ice structures rather than larger, jagged ones contributes to maintaining the physical integrity of the sample.
Notably, at high pressures, the thermodynamic equilibrium shifts. This shift means that under specific conditions, the energy required to initiate the phase transition is significantly reduced. As a result, the freezing process becomes more efficient. This principle underlies many of the applications of HPF in fields ranging from biological research to material science.
Phase Transitions Under Pressure
Phase transitions are intrinsic to the process of high pressure freezing and are thoroughly affected by the conditions surrounding them. When applying pressure, water does not simply freeze; the transition involves complex interactions between temperature, pressure, and molecular structure. Under high pressure, the movement of water molecules becomes constrained. This physical restraint prevents the orderly crystal lattice formation typical of ice.
Jeremy J. Borkowski observed that during HPF, there's an essential change in the volume and density of the sample as pressure increases. Because transitions occur without the formation of significant ice crystals, biological materials maintain their cellular architecture more effectively. This feature is particularly beneficial in studies examining cellular interactions, as it allows researchers to observe native conditions.
In addition, understanding phase transitions paves the way for advancements in HPF techniques. Ongoing research explores ways to fine-tune pressure and temperature parameters. The goal is to optimize sample preservation without compromising cellular viability. This continual refinement of the method underscores the relevance of phase transitions as a focal point in high pressure freezing research.
High pressure freezing leverages thermodynamic principles to create an environment where undesirable phase transitions can be avoided, preserving sample integrity.
By grasping these fundamental thermodynamic principles and phase behaviors, researchers and professionals can better appreciate the transformative impact of HPF on cryopreservation. The intricate relation between pressure, temperature, and freezing processes reveals a sophisticated methodology that enhances sample preservation in various scientific fields.
Technical Aspects of High Pressure Freezing
Understanding the technical aspects of high pressure freezing (HPF) is essential for leveraging its advantages in various fields, particularly in bioligical sciences and material sciences. This methodology stands out not only for its effectiveness in sample preservation but also for its unique approach by combining extreme pressure with rapid freezing. This section will explore three critical elements: the equipment and setup needed for HPF, the techniques used for sample preparation, and in-situ observations during the freezing process.
Equipment and Setup
The equipment used in high pressure freezing includes high-pressure chambers designed to withstand significant forces while enabling the rapid freezing of samples. The typical system consists of a pressurizing unit and a freezing stage. The pressurizing unit applies the required pressure, usually in the range of 1000 to 3000 Bar, while rapidly cooling the sample to cryogenic temperatures.
Key components of the setup include:
- High-pressure freezer: A crucial apparatus where samples are loaded and subjected to pressure and cooling simultaneously.
- Cooling system: This could involve liquid nitrogen or other refrigeration agents that bring the temperature down swiftly.
- Sample containers: Specific containers need to be used to hold the biological or materials samples securely. These are often made from materials that can withstand high pressure without deformation.
A proper setup not only enhances the efficiency of the freezing process but also minimizes the risk of ice crystal formation, thus preserving the integrity of the sample.
Sample Preparation Techniques
Preparing samples for high pressure freezing is another crucial step. It requires meticulous attention to ensure that the samples are compatible with the HPF process. Common techniques involve the following:
- Embedding: Samples often need to be embedded in a suitable medium before freezing. This medium should be cryoprotective and stable under high pressure.
- Triming samples: Before freezing, samples should be trimmed to a suitable size, as thicker samples may not freeze effectively and could suffer damage.
- Controlling hydration levels: Maintaining proper hydration is important as it influences how well samples can sustain the high-pressure atmosphere.
Each technique aims to optimize the sample's preservation by limiting any potential damage during the freezing process.
In-situ Observations During Freezing
Observing the freezing process in real-time provides invaluable insights into the dynamics of HPF. This step can involve sophisticated imaging techniques that allow researchers to monitor sample behavior as pressure is applied. Key techniques include:
- Cryo-electron microscopy (Cryo-EM): This technique enables detailed imaging of the samples at cryogenic temperatures, observing structural changes without altering the sample's environment.
- Phase contrast microscopy: Helpful in visualizing phase changes in the sample during the freezing process, affirming that no ice crystal formation occurs.
In-situ observations reveal critical information about the sample's structure during freezing, providing an opportunity to refine HPF techniques further and applications.
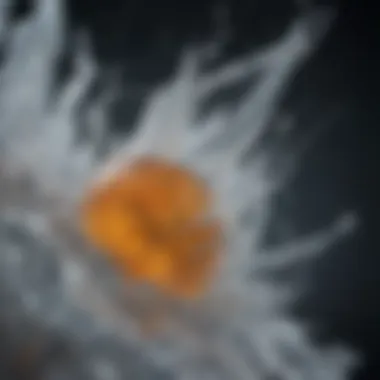
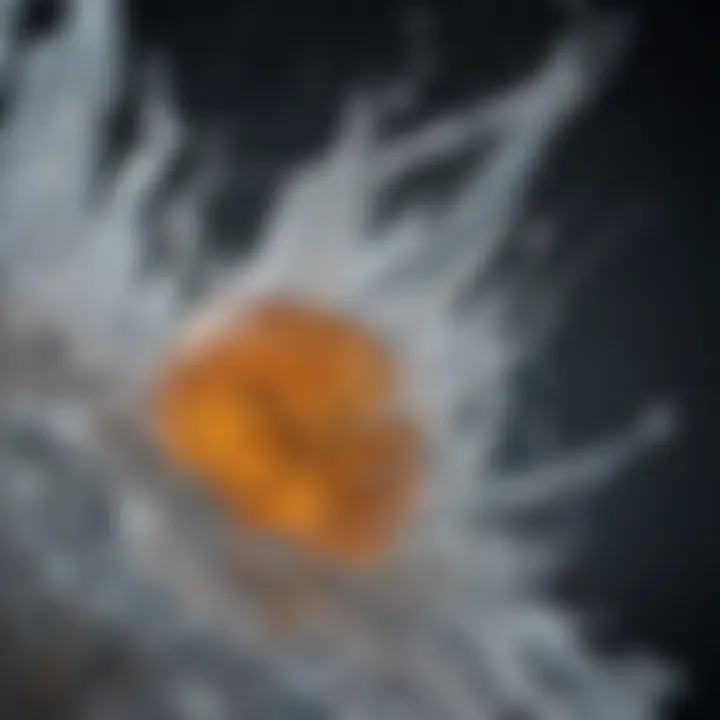
Cryopreservation and Its Importance
Cryopreservation is a critical process for preserving biological samples. It involves cooling cells, tissues, or any biological samples to sub-zero temperatures to halt all biological activity, including the processes that lead to cell death. This technology is essential in various scientific fields, including medical research, agriculture, and conservation biology. As researchers continue to explore intricate biological systems, understanding cryopreservation becomes vital for experimental robustness and sample integrity.
The Role of Cryopreservation in Research
Cryopreservation plays a significant role in enabling long-term storage of biological materials. For instance, in biomedical research, it allows for the preservation of stem cells and reproductive cells, which can be essential in studies related to fertility and genetic disorders. In cancer research, preserving tumor tissues for future analysis can aid in the development of targeted therapies.
Moreover, cryopreservation facilitates
- Sample Sharing: Researchers can share samples without significant degradation, ensuring that experiments maintain reproducibility.
- Elimination of Seasonality: In agricultural research, plants can be preserved regardless of season, allowing for the continuity of breeding programs and genetic analyses.
This ability to maintain sample viability is fundamental to advancing scientific knowledge and facilitating collaborative research endeavors.
Comparison with Traditional Freezing Methods
Traditional freezing methods, such as slow freezing, involve lowering the temperature at a gradual pace. While these methods can be effective, they often lead to the formation of ice crystals, which can cause cellular damage. In contrast, high pressure freezing (HPF) can mitigate such risks, offering the following advantages:
- Minimization of Ice Crystal Formation: HPF reduces the likelihood of ice crystal development, thus preserving cellular architecture.
- Speed of Freezing: It enables rapid freezing processes that prevent the biological impairment generally associated with traditional slow freezing methods.
- Maintaining Structural Integrity: The method ensures that samples retain their native structures, which is crucial for accurate research findings.
Applications of High Pressure Freezing
High pressure freezing (HPF) has emerged as a transformative technique across various scientific domains. Its applications, notably in biology, materials science, and food science, highlight its versatility and the distinct advantages it offers compared to traditional freezing methods. Understanding these applications is crucial for recognizing the significance of HPF in preserving sample integrity and advancing research.
Biological Samples
In the realm of biological research, HPF is invaluable. This method allows for the preservation of cellular structures at cryogenic temperatures, mitigating the detrimental effects of ice crystal formation. When cells are frozen using traditional methods, ice crystals can disrupt the delicate architecture of biological tissues, leading to compromised structural fidelity and function upon thawing. However, HPF creates a glassy state in the biological material, effectively avoiding ice crystal growth.
Key benefits of using HPF for biological samples include:
- Enhanced Sample Integrity: Cells retain their native morphology, which is essential for accurate imaging and analysis.
- Preservation of Cellular Function: The functionality of proteins, lipids, and other biomolecules remains intact, facilitating reliable studies.
- Increased Resolution in Imaging Techniques: Techniques such as electron microscopy benefit from the high-resolution images made possible by HPF.
Research areas like cell signaling, neurobiology, and developmental biology have all seen advancements owing to HPF. This methodology enables scientists to observe cellular processes at a level of detail that was previously unattainable, thus deepening our understanding of various biological mechanisms.
Material Science
Material science also benefits significantly from HPF. The ability to freeze materials under high pressure facilitates the preservation of their structure and properties. This is particularly important when studying materials that are sensitive to temperature changes or phase transitions. When subjected to HPF, materials often undergo rapid solidification without the formation of defects caused by cooling at normal atmospheric pressure.
Interesting applications in material science include:
- Composite Materials: HPF can be used to study the microstructure of composite materials, offering insights that can influence their manufacturing processes.
- Metallurgy: The technique aids in understanding phase transformations in metals, contributing to enhanced material properties and performance.
- Polymer Science: HPF is utilized for the preservation of polymer structures, which is critical in the development of new materials with specific properties.
These applications are essential for the development of advanced materials with tailored properties, promoting innovation in fields such as aerospace, automotive, and electronics.
Food Science Applications
The food science sector is another area where HPF has shown promising applications. Traditional freezing methods can negatively impact the texture and flavor of food products due to ice crystal formation. With HPF, food items can be frozen while maintaining their cellular structure, thereby preserving their original textures and enhancing sensory qualities.
Practical applications in food science include:
- Preserving Freshness: HPF minimizes degradation of food quality, resulting in better preservation of nutrients and flavors.
- Enhanced Texture: Meats, fruits, and vegetables maintain their firmness and juiciness after freezing and thawing.
- Improved Shelf Life: The reduction in ice crystal formation translates to extended shelf life of frozen products.
These advantages underscore the importance of HPF in modern food processing, ultimately providing consumers with higher quality products.
High pressure freezing preserves biological and material integrity without damaging the samples, making it a key methodology in various research fields.
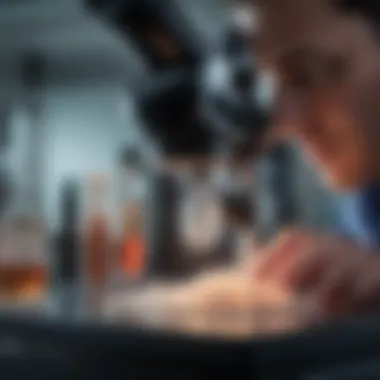
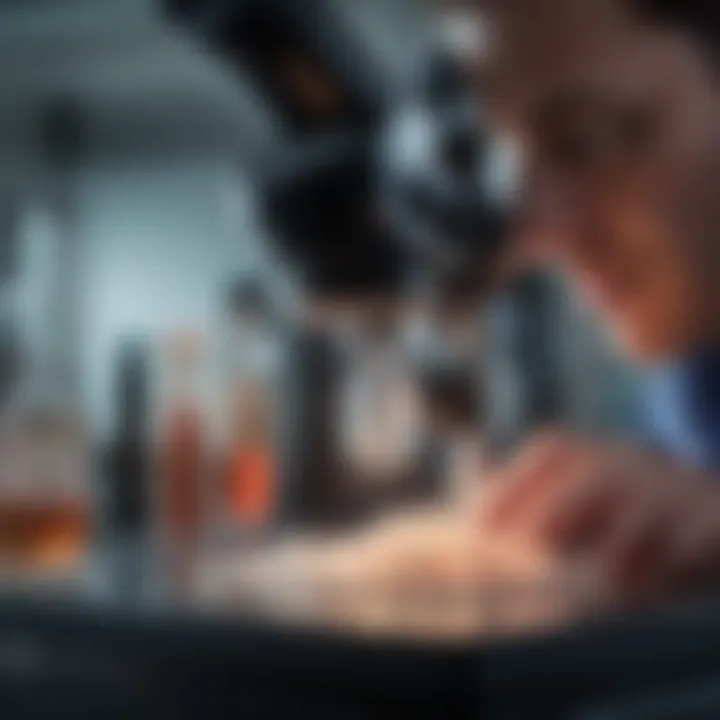
Overall, the versatility of high pressure freezing across biology, material sciences, and food science highlights its critical role in enhancing research and practical applications. Advancements in this technique hold the potential to further broaden its applications, paving the way for innovative approaches in multiple disciplines.
Challenges in High Pressure Freezing
High pressure freezing is an advanced technique that, while invaluable for preserving biological and material samples, comes with its share of challenges. As researchers and practitioners increasingly adopt this method, it is crucial to identify and understand these difficulties. Addressing the challenges can lead to the refinement of techniques and better outcomes in various applications. The two primary areas of concern include technical limitations and biological risks, both of which can impact the efficacy of high pressure freezing.
Technical Limitations
Technical constraints can profoundly affect the outcome of high pressure freezing procedures. Several factors contribute to these limitations, and acknowledging them can help in the pursuit of improved strategies.
- Equipment Variability: The performance of high pressure freezing largely hinges on the equipment used. There are differences in design, capacity, and pressure handling capabilities among various models available in the market. This variability can lead to inconsistent results, making it vital for researchers to choose the right apparatus that suits their specific needs.
- Sample Size and Type Restrictions: Not all samples can undergo high pressure freezing. Certain biological specimens are sensitive to extreme pressures and rapid cooling, resulting in inadequate preservation. Understanding the specific characteristics of each sample type is essential for successful cryopreservation.
- Cost Implications: The cost associated with high pressure freezing equipment and maintenance can be substantial. Smaller laboratories may struggle to allocate the necessary funding to acquire and maintain such specialized equipment, limiting access to this technique.
- Operational Skills: Mastery of high pressure freezing techniques is necessary to achieve optimum results. Lack of trained personnel can lead to errors in setup and execution, ultimately impacting sample integrity.
Biological Risks
Biological risks during high pressure freezing can compromise the quality of the preserved samples. These risks often arise from the biological composition of the samples and the conditions applied during the procedure.
- Cellular Stress: High pressure and temperature changes can potentially cause cellular stress. This stress could lead to alterations in cell membranes and affect overall sample quality. Understanding cellular responses to pressure is important for developing protocols that minimize such risks.
- Ice Crystal Formation: Although high pressure freezing aims to prevent ice crystal formation, improper execution can still lead to crystal growth, especially in sensitive samples. This can compromise the structural integrity of the biological material, negating the benefits of the technique.
- Viability Concerns: The preservation of living cells at cryogenic temperatures must ensure their viability post-thawing. Certain cell types may not recover well after high pressure freezing, leading to questions about the reliability of the methodology for various applications.
- Compound Interactions: Samples may contain various chemical compounds that can interact differently under high pressure and low temperatures. Such interactions could lead to damage or changes in sample characteristics, making careful consideration of sample composition crucial.
In summary, the challenges of high pressure freezing, ranging from technical limitations to biological risks, highlight the need for careful planning and execution to ensure successful outcomes in cryopreservation. Recognizing these challenges is the first step towards advancing the methodology.
Future Directions in High Pressure Freezing Research
The field of high pressure freezing is continuously evolving, driven by technological advancements and increased research demand. It is crucial to explore future directions in this area as they can enhance the efficiency and applicability of this preservation technique. Researchers are investigating innovative methodologies that can further minimize the risks associated with cryopreservation, expand its practical applications, and optimize the processing of biological and material samples. Understanding these future trends will provide valuable insight into how high pressure freezing can continue to advance the sciences and improve experimental outcomes.
Innovative Techniques on the Horizon
Several innovative techniques are emerging in high pressure freezing research. One notable development is the integration of automated robotic systems to facilitate sample handling and freezing processes. Automation can improve precision, allowing for more consistent results, and it significantly reduces human error. Additionally, researchers are exploring the use of alternative cryoprotectants that could minimize cellular damage during the freezing process. For instance, compounds that exhibit better molecular properties may be employed to eliminate or reduce ice crystal formation, preserving the structural integrity of sensitive samples.
Another promising avenue of research includes the enhancement of imaging technologies that can be paired with high pressure freezing. By utilizing advanced imaging methods like cryo-electron tomography, scientists can observe cellular changes in real-time during the freezing process. This can unveil new insights into cryobiology and help refine freezing protocols based on observed outcomes.
Potential For Broader Applications
The future of high pressure freezing holds potential for broader applications across various fields beyond biological studies. In materials science, for example, the ability to rapidly freeze samples while preserving their physical state can lead to more effective studies on metal and ceramic materials under extreme conditions. This can advance the development of new alloys and composites with enhanced properties.
Moreover, the food industry could benefit significantly from high pressure freezing methodologies. Managing the quality of perishable goods remains a major concern. By applying high pressure freezing techniques, food products could maintain their original texture and flavor while prolonging shelf life. Researchers are also investigating the possibility of applying high pressure freezing in environmental sciences, particularly in the preservation of diatoms or microorganisms in situ for ecological studies.
Epilogue and Implications
High pressure freezing (HPF) stands out as a pivotal methodology in the realm of cryopreservation. Its ability to preserve biological samples without the detrimental effects of ice crystal formation is crucial. The technique ensures that cellular structures remain intact during the freezing process, enabling better study and understanding of biological processes at low temperatures. This feature addresses one of the significant drawbacks associated with traditional freezing methods, which often compromise sample integrity.
The implications of effectively utilizing HPF extend beyond simple preservation. For researchers, this translates to improved data accuracy and reliability when analyzing delicate samples. The details retained in samples frozen via HPF allow for high-resolution imaging and advanced analysis, which are not achievable through other freezing methods. This capability is particularly beneficial in fields like cell biology, where the need to understand cellular architecture and dynamics is paramount.
In terms of practical applications, various research disciplines can leverage HPF to further explore complex biological phenomena. As such, HPF represents a conduit for innovation in cryobiology, offering the potential to advance therapeutic strategies involving cryopreservation. This shift toward more refined methodologies marks a significant step in enhancing research outcomes, ultimately leading to more effective applications in medical and biological research.
"High pressure freezing emphasizes the importance of cryopreservation techniques that maintain sample integrity across multiple disciplines."
Despite the benefits, there are considerations to bear in mind. Researchers must weigh the costs and technical requirements of HPF against other methodologies. The complexity of setting up high-pressure systems and the potential need for specialized training cannot be ignored. However, with ongoing advancements and growing accessibility, HPF's adoption will likely increase.
Summary of Key Findings
High pressure freezing has emerged as a superior alternative to traditional freezing methods. Key findings regarding HPF include:
- Preservation of native structures: HPF minimizes ice crystal formation, which is essential for maintaining the integrity of biological samples.
- Enhanced imaging capabilities: The technique supports high-resolution analysis, crucial for scientific research and diagnostics.
- Diverse applications: HPF is valuable in various fields, including cell biology, material science, and food science, enabling broader exploration of cryopreservation.
- Technological advancements: Ongoing research suggests improvements in HPF technology, making it more accessible and efficient.
Anticipated Developments in the Field
Looking ahead, several anticipated developments in high pressure freezing are noteworthy:
- Innovative techniques: Research is likely to yield new methods that further enhance freezing speed and efficiency while maintaining sample quality.
- Broader application scopes: As HPF technology evolves, new fields may emerge where cryopreservation becomes invaluable, such as regenerative medicine or biotechnology.
- Integration with imaging technologies: Advances in imaging solutions may allow for real-time analysis during the freezing process, providing deeper insights into cellular dynamics.
- Collaborative research efforts: Increased collaborations between technology developers and biological researchers can accelerate HPF's evolution, fostering innovative solutions that push the boundaries of current methodologies.