Understanding Immune Cells in Antibody Production
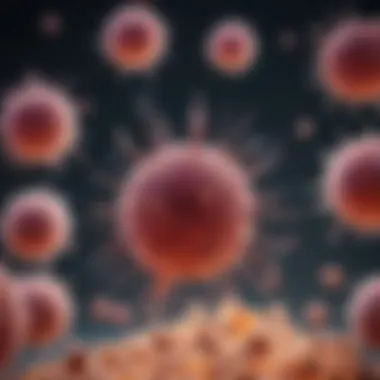
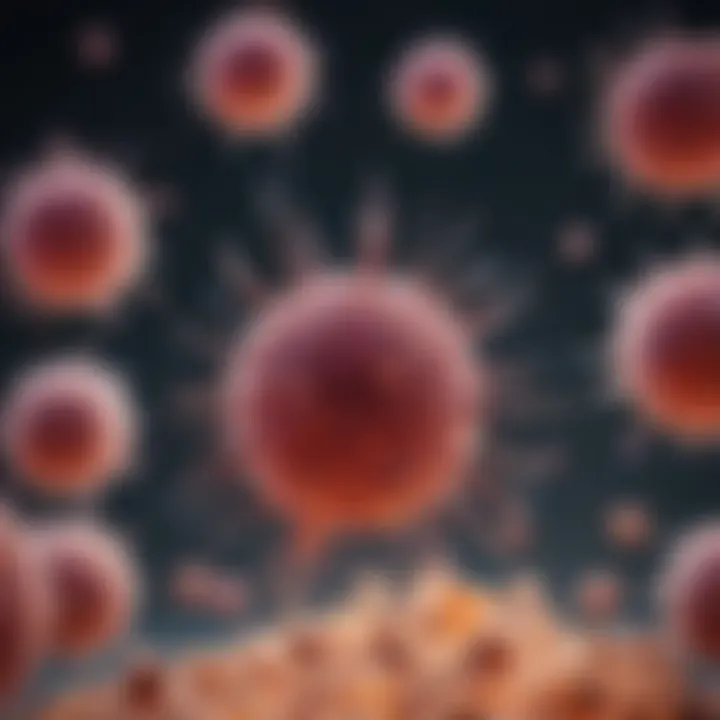
Intro
Understanding the immune system is crucial for grasping how our bodies defend against pathogens. Among its various components, immune cells play a significant role in antibody production. Antibodies are essential proteins that identify and neutralize foreign objects such as bacteria and viruses. This article will explore the diverse immune cells involved, their activation processes, and the broader implications of these functions in health and disease.
Research Overview
Methodological Approaches
Research into immune cells and their functions is multifaceted. Experiments typically employ techniques like flow cytometry, immunofluorescence, and cytokine assays. These methods allow scientists to analyze cell populations, study their behavior, and quantify cytokine release. Animal models, particularly mice, are commonly used to investigate the immune responses in vivo. This research is critical for understanding the mechanisms underlying humoral immunity.
Significance and Implications
The implications of antibody production are vast. Effective vaccination strategies rely fundamentally on a well-functioning immune system to produce antibodies against specific pathogens. Understanding immune cell dynamics also paves the way for treatments in autoimmune diseases, where the body incorrectly targets its tissues. Overall, exploring immune cells can inform future therapies and public health policies, underscoring the relevance of this field of study.
Current Trends in Science
Innovative Techniques and Tools
Recent advances have transformed the landscape of immunology research. Technologies like single-cell RNA sequencing and CRISPR gene editing offer unprecedented opportunities to examine the genetic and functional diversity within immune cell populations. These innovations facilitate a more precise understanding of how different cells contribute to humoral immunity, guiding next-generation therapies.
Interdisciplinary Connections
The study of immune cells is not confined to immunology alone. Researchers from fields such as bioinformatics, molecular biology, and even computer science collaborate to analyze complex data sets. These interdisciplinary approaches enhance our understanding of immunity and promote the development of novel therapies that integrate multiple scientific domains.
“The immune system is a complex network, and every detail counts in understanding its response to threats.”
Overall, the exploration of immune cells in antibody production is a dynamic and evolving field, impacting various aspects of medical science and practice.
Prelims to the Immune System
The immune system is indispensable for maintaining health. It protects the body from pathogens, including bacteria, viruses, and parasites. Understanding its mechanisms is crucial for researchers and healthcare professionals. This section will cover the essential functions and components of the immune system, setting the stage for a detailed exploration of antibody production.
Overview of Immune Function
At its core, the immune system comprises a network of cells, tissues, and organs that collaborate to fend off infections. The primary purpose is to identify and neutralize foreign invaders. Key components include:
- Lymphocytes: Immune cells like B cells and T cells play a pivotal role.
- Antigen-presenting cells: These cells help in recognizing pathogens.
- Cytokines: Small proteins that mediate communication between cells.
Each element works harmoniously to ensure effective immune response. Importantly, the immune system learns from encounters with pathogens, adapting for improved responses in future infections.
Types of Immune Responses
The immune response can be categorized mainly into two types: innate and adaptive immunity.
- Innate Immune Response: This is the body's first line of defense. It provides a rapid, but non-specific reaction to pathogens. Cells involved in this response include macrophages and natural killer cells. They act quickly to contain infections and initiate further immune activities.
- Adaptive Immune Response: This type is more specialized and slower to activate. It relies on B cells and T cells. B cells produce antibodies, while T cells help regulate immune responses. This response has a memory component, allowing for more efficient reactions in the future.
Both types are crucial for overall immune health. Understanding these aspects aids in comprehending the subsequent sections on antibody production. The interplay between these immune components underscores the complexity and sophistication of the immune system.
Antibodies: Structure and Function
Antibodies play a crucial role in our immune defense. Understanding their structure and function is paramount. Antibodies are specialized proteins produced by B cells in response to antigens. Antigens are harmful substances, such as pathogens, that trigger an immune response. The unique structure of antibodies enables them to bind specifically to these antigens.
Definition and Importance of Antibodies
Antibodies, also known as immunoglobulins, are glycoproteins that are essential for identifying and neutralizing pathogens. There are five classes of antibodies: IgG, IgA, IgM, IgE, and IgD, each with distinct roles in the immune response.
The importance of these molecules cannot be overstated. They are pivotal for several reasons:
- Targeting Pathogens: Their primary function is to recognize and bind to specific antigens on pathogens. This process marks the pathogens for destruction.
- Neutralization: By binding to pathogens, antibodies can neutralize toxins or viruses, preventing them from entering or harming cells.
- Activation of Complement System: Antibodies can activate the complement system, a group of proteins that enhance the ability of antibodies to clear microbes and damaged cells.
- Immune Memory: Some antibodies remain in circulation for long periods. This memory leads to a faster and more effective response during subsequent exposures to the same pathogen.
In summary, antibodies serve as a critical defense mechanism within the immune system, providing the body with the ability to recognize and efficiently combat infections.
Types of Antibodies
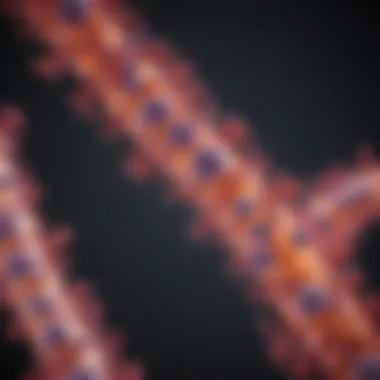
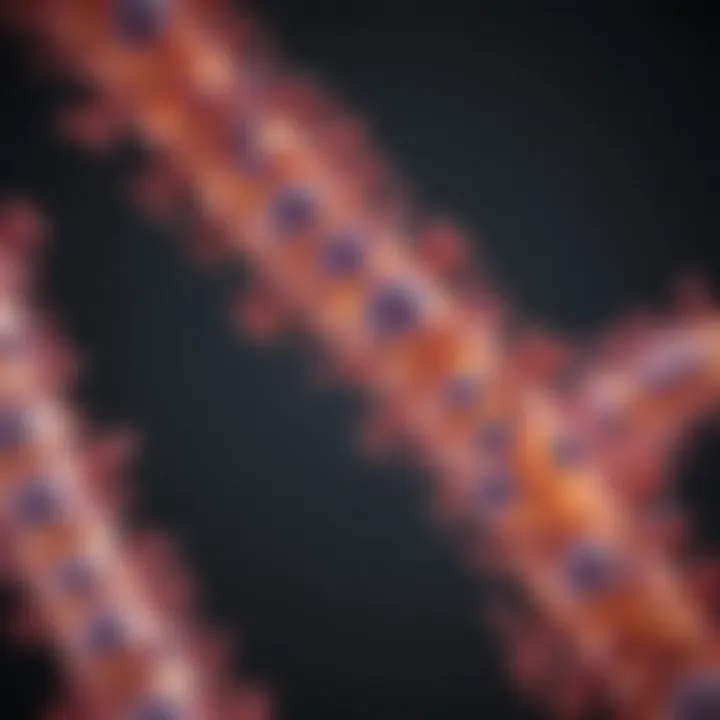
The classification of antibodies reflects their diverse roles in the immune system. Each type has unique features and functions, contributing to a well-coordinated immune response:
- IgG: This is the most abundant antibody in the bloodstream. IgG provides long-term protection by neutralizing toxins and viruses, and it can cross the placenta, offering fetal protection.
- IgA: Found primarily in mucosal areas like the gut, lungs, and saliva, IgA plays a crucial role in mucosal immunity. It prevents the adherence of pathogens to epithelial cells.
- IgM: The first antibody produced in response to an infection, IgM is effective at forming complexes with antigens. It facilitates the clearance of pathogens from the bloodstream.
- IgE: This antibody is primarily involved in allergic reactions. IgE binds to allergens and triggers histamine release from mast cells, leading to inflammation and other allergic responses.
- IgD: Although its function is less understood, IgD is found on the surface of B cells and is believed to play a role in initiating B cell activation.
"Antibodies are not just tools of the immune system; they reflect the complex interactions between pathogens and host defenses."
By recognizing these different types and their specific functions, we can enhance our understanding of how antibody responses shape overall immunity and guide therapeutic developments.
The Cells Involved in Antibody Production
Understanding the cells involved in antibody production is essential to appreciating the complexities of the immune response. Immune cells work together in a highly coordinated fashion. Their interplay is necessary for the generation and maintenance of effective antibody responses that protect the body against pathogens.
Within this section, we will explore the pivotal roles of B cells, T cells, and antigen-presenting cells in the generation of antibodies. Each of these cell types contributes uniquely to the outcome of immune responses. Recognizing these contributions can elucidate potential therapeutic strategies and inform clinical applications as well.
B Cells: The Key Players
B cells are the primary cells responsible for antibody production. They are a type of lymphocyte derived from bone marrow and play a critical role in the adaptive immune response. When B cells encounter antigens, they undergo a process called activation. This activation leads to proliferation and differentiation into plasma cells, which are the cells that secrete antibodies.
The process of B cell activation hinges on several factors, primarily the binding of specific antigens to B cell receptors. This binding initiates a signaling cascade that prompts their activation. Furthermore, helper T cells provide essential signals in the form of cytokines and direct cellular interactions.
During activation, B cells also experience class switching, allowing them to produce different types of antibodies based on the needs of the immune response. This flexibility is key to an effective defense mechanism.
T Cells: Regulatory Support
T cells play a fundamental regulatory role during the immune response, supporting B cells in their efforts to produce antibodies. There are two main types of T cells involved in this process: helper T cells and regulatory T cells.
Helper T cells, or CD4+ T cells, enhance the activity of B cells. They provide necessary signals that help B cells to mature. Helper T cells release cytokines that guide B cell differentiation into plasma cells. This interaction is vital for establishing a robust immune response.
Regulatory T cells, on the other hand, act as a balancing mechanism. They modulate the immune response and prevent excessive or inappropriate responses. This regulatory role is crucial to maintain homeostasis and prevent autoimmune reactions, which can occur if B cells produce antibodies against the body's own tissues.
Role of Antigen-Presenting Cells
Antigen-presenting cells (APCs) are another integral component in the antibody production process. These cells, which include dendritic cells, macrophages, and B cells themselves, are responsible for capturing, processing, and presenting antigens to T cells.
When APCs present antigens, they use specialized molecules called major histocompatibility complex (MHC) proteins. This interaction is critical for the activation of T cells, which in turn influences B cell activation and subsequently antibody production.
The ability of APCs to efficiently present antigens influences the quality and magnitude of the antibody response. A strong and well-coordinated involvement of APCs can enhance the immune response, resulting in the generation of high-affinity antibodies that are essential for effective pathogen clearance.
In summary, understanding the roles of B cells, T cells, and antigen-presenting cells in antibody production provides insights into the immune system's complexity. This knowledge is crucial for advancing immunological research and therapeutic interventions.
B Cell Development and Activation
B Cell Development and Activation is a critical aspect of the immune system’s ability to produce antibodies. This section focuses on how B cells arise, mature, and become fully functional. Understanding these processes is essential to appreciate the overall complexity of humoral immunity and the various factors influencing it.
Stages of B Cell Maturation
B cells originate from hematopoietic stem cells in the bone marrow. The primary stages of B cell maturation include:
- Pro-B Cells: These are the earliest stage in B cell development. They undergo proliferation and begin to rearrange their immunoglobulin (Ig) genes.
- Pre-B Cells: In this phase, the rearrangement of the heavy chain genes occurs. Successful rearrangement leads to the expression of the pre-B cell receptor (pre-BCR).
- Immature B Cells: Once a functional heavy chain is expressed, the cell assembles with light chains to form a complete B cell receptor (BCR). These immature B cells then undergo selection processes to eliminate self-reactive cells.
- Mature B Cells: Finally, the immature B cells that pass selection migrate to peripheral lymphoid tissues and complete their maturation. They express both IgM and IgD on their surface and are now considered mature but naive B cells, ready to respond to antigens.
These stages are vital as they ensure a diverse repertoire of B cell receptors, crucial for recognizing various antigens.
Processes Leading to Activation
B cell activation is a multi-step process that requires specific signals. Below are the main processes involved:
- Antigen Recognition: Mature B cells circulate through lymphoid tissues. Upon encountering an antigen, the BCR binds and internalizes the antigen.
- Co-stimulation: For full activation, B cells require additional signals from T helper cells. This often happens through the interaction between CD40 on B cells and CD40L on T cells, which promotes further B cell differentiation.
- Cytokine Signaling: Cytokines produced by helper T cells contribute to the activation and proliferation of B cells. Cytokines like interleukin-4 and interleukin-21 are particularly important for promoting B cell growth and differentiation.
- Clonal Expansion: Once activated, B cells undergo clonal expansion, creating a population of cells that produce the same specific antibodies. This step is crucial for generating a robust immune response.
The interplay of signals during B cell activation significantly impacts the efficiency of humoral immunity, influencing the scope and effectiveness of the immune response.
By understanding the stages of B cell maturation and the processes that lead to their activation, one gains insight into how the body produces antibodies in response to pathogens. This knowledge is vital in applied immunology, vaccine development, and therapeutic interventions.
Mechanisms of Antibody Production
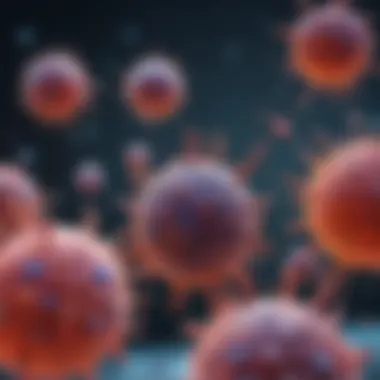
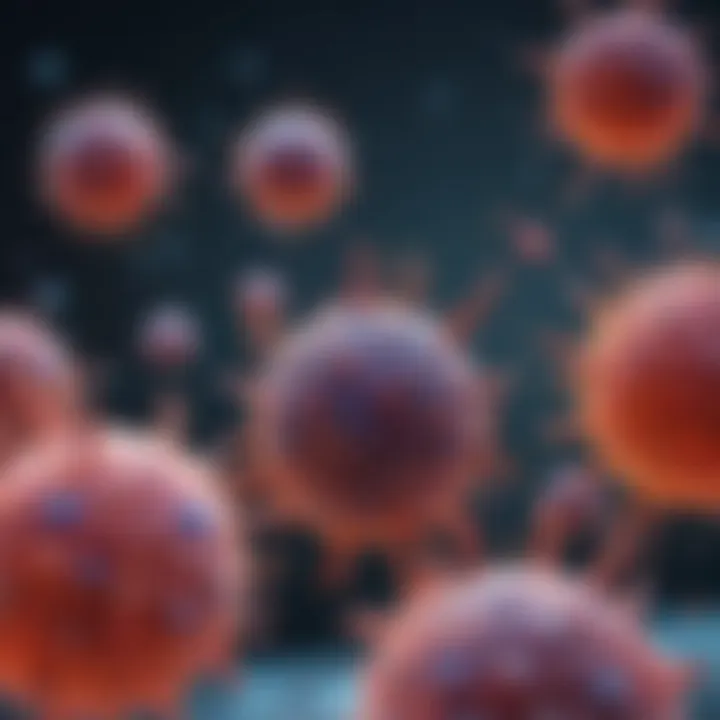
The mechanisms of antibody production are central to understanding how the immune system defends against pathogens. This section explores the intricate processes involved and highlights their importance in humoral immunity. Understanding these mechanisms is vital not just for academic enrichment but also for practical applications in medical therapies and vaccine development.
Class Switching
Class switching, also known as isotype switching, is a process that allows B cells to produce different classes of antibodies, such as IgM, IgG, IgA, and IgE, from a single immunoglobulin gene. This switch is crucial because it enables the immune response to adapt to varying types of infections.
When a B cell encounters an antigen, it initially produces IgM antibodies, which respond to infections rapidly. However, as the immune response evolves, signals from T helper cells and cytokines stimulate the B cells to switch to other classes. Each class serves distinct functions:
- IgM: First responder, effective in forming immune complexes.
- IgG: Offers long-term protection and can cross the placenta, providing immunity to the fetus.
- IgA: Mainly found in mucosal areas, crucial for protecting surfaces like the gut and respiratory tract.
- IgE: Plays a role in allergic reactions and defense against parasites.
Importance of Class Switching:
- It enhances the effectiveness of antibodies.
- It allows for targeted immune responses.
- It contributes to immune diversity, improving the ability to combat various pathogens.
Affinity Maturation
Affinity maturation is a process that increases the affinity of antibodies for their specific antigens. This refinement occurs in the germinal centers of lymph nodes, where B cells undergo somatic hypermutation. During this process, the variable region of the antibody genes is mutated, leading to the production of different antibody variants.
B cells that produce antibodies with a higher affinity for the antigen are preferentially selected for survival. This cyclic process results in:
- Enhanced binding strength between antibodies and antigens.
- Increased efficiency in pathogen neutralization.
- Improved longevity of memory B cells, ensuring better responses to future infections.
Key Points about Affinity Maturation:
- Vital for sustained immune protection.
- Contributes to the effectiveness of vaccines.
- Affects the design of therapeutic antibodies in clinical applications.
This advancement in understanding antibody production mechanisms is foundational for future immunological research and development of new therapies.
The Cytokine Environment
Cytokines play a crucial role in directing the immune response, especially in the context of antibody production. These small proteins, secreted by various immune cells, act as signals that help cells communicate. Understanding the cytokine environment is essential for grasping how immune cells work together to mount effective responses against pathogens.
Role of Cytokines in Immune Response
Cytokines are often described as the language of the immune system. They influence a variety of immune activities, which include the growth, differentiation, and activation of immune cells. Some key functions of cytokines in immune responses are:
- Stimulating B Cell Activation: Certain cytokines, such as interleukin-4 (IL-4) and interleukin-21 (IL-21), are particularly vital for the activation and proliferation of B cells.
- Enhancing T Cell Functions: They are also significant in modulating T cell responses, which can indirectly affect antibody production by helping B cells understand when to respond.
- Facilitating Communication: Cytokines enable different immune cells to work together efficiently, forming a complex network that ensures a balanced immune response.
"Cytokines are integral to immune response, impacting how immune cells collaborate to defend the body."
The delicate balance and proper timing of cytokine release determine whether the immune response is effective or leads to harmful outcomes. Therefore, studying this environment can lend insights into potential therapeutic interventions.
Cytokines Impacting B Cell Function
B cells require specific cytokines for proper development and antibody production. The primary cytokines that influence B cell functions include:
- Interleukin-4 (IL-4): Vital for B cell activation, IL-4 promotes their growth and survival. This cytokine helps in class switching, allowing B cells to produce various antibody types tailored to different pathogens.
- Interleukin-5 (IL-5): Primarily supports B cell differentiation and activity. It is crucial for the production of eosinophils, which can further mediate immune responses.
- Interleukin-6 (IL-6): This cytokine supports the transition of B cells into antibody-producing plasma cells. It contributes to the overall immune response during infection.
The presence and concentration of these cytokines influence how B cells develop and function. A well-regulated cytokine environment is crucial for generating an effective antibody response, underscoring the interconnectedness of the immune system.
Understanding how these cytokines regulate B cells could lead to advancements in therapies that target immune-related conditions, showcasing the significance of the cytokine environment in health and disease.
The Role of Memory Cells
Memory cells are a critical aspect of the immune system, specifically in the context of antibody production. They serve to enhance the efficiency and speed of the immune response during subsequent encounters with pathogens. This section will explore the formation of memory B cells and their role in reactivation during subsequent infections, elucidating their importance in long-term immunity.
Formation of Memory B Cells
The formation of memory B cells occurs after an initial exposure to an antigen. When naive B cells encounter their specific antigen, they undergo activation. This activation involves the proliferation and differentiation of B cells, resulting in the production of effector B cells, primarily plasma cells, which produce antibodies.
However, not all activated B cells become plasma cells. Some differentiate into memory B cells. These memory B cells remain in the body for years or even decades, and they have a distinct function. Unlike plasma cells, which are short-lived and responsible for immediate antibody secretion, memory B cells can quickly respond to the same antigen upon re-exposure.
The process of formation can be affected by various factors, such as the type of antigen, the presence of helper T cells, and cytokines in the immune environment. When B cells undergo class switching and affinity maturation, they create high-affinity memory B cells capable of producing more effective antibodies during future encounters with the relevant pathogen.
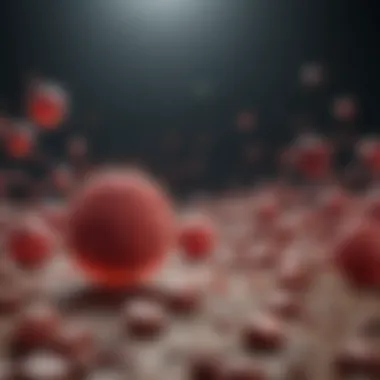
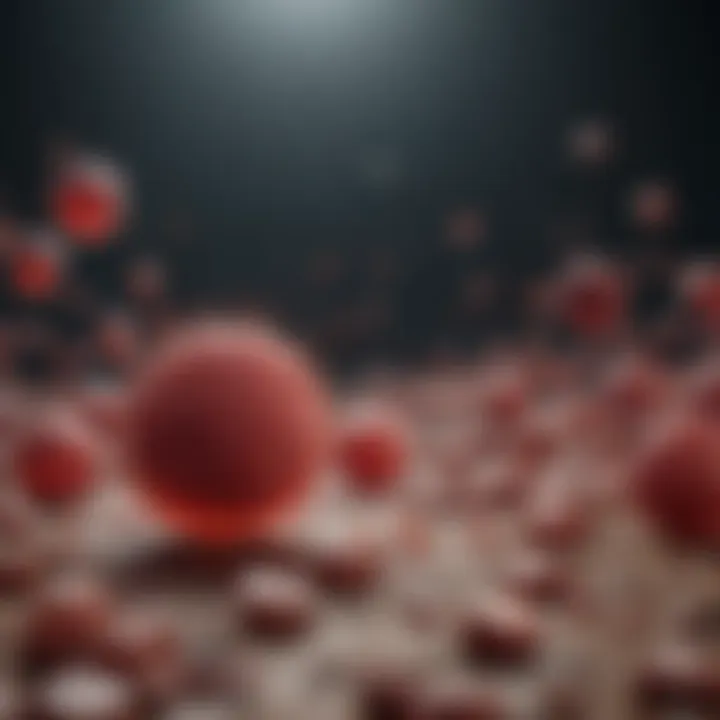
Reactivation in Subsequent Infections
When the immune system encounters the same pathogen again, memory B cells play a vital role in quickly generating a robust antibody response. This reactivation process is fundamental for effective immunity. Memory B cells rapidly proliferate and differentiate into plasma cells, resulting in the production of antibodies within days, significantly faster than during the primary response.
This adaptive response is critical in reducing the severity of subsequent infections or, in some cases, preventing them altogether. Immunizations take advantage of this mechanism by introducing antigens in a controlled manner to create memory B cells without causing disease. This approach enhances the body's defense against various pathogens by equipping the immune system for quicker responses.
Implications of Antibody Production
The process of antibody production in the human immune system has far-reaching implications. Understanding these implications is critical for both scientific research and clinical practice. Antibodies are essential for protecting the body from pathogens. They also play a major role in therapeutic interventions, making their production a focal point in immunology.
Clinical Applications in Vaccination
Vaccination relies heavily on the principle of antibody production. When a vaccine is administered, it introduces a harmless component of a pathogen. This prompts the immune system to respond by producing antibodies specifically tailored to that pathogen. The benefits include:
- Protection: Vaccines prepare the immune system in advance, allowing for a swift response to actual infections.
- Herd Immunity: Widespread vaccination can diminish the spread of disease, protecting those who cannot be vaccinated.
- Long-term Immunity: Some vaccines stimulate memory B cells, leading to long-lasting immunity. This decreases the incidence of disease outbreaks.
Research in this area has led to the development of novel vaccines, including mRNA vaccines like Pfizer-BioNTech and Moderna, which utilize the body’s machinery to produce proteins that resemble those of the virus. These proteins trigger an immune response, resulting in robust antibody production. Vaccination strategies must, however, be assessed continuously to match evolving viruses, as seen with influenza and COVID-19.
Therapeutic Antibodies in Treatment
Therapeutic antibodies, often referred to as monoclonal antibodies, are produced in laboratories to combat diseases. They offer targeted treatment for various conditions, including cancer, autoimmune diseases, and infectious diseases. The creation of therapeutic antibodies involves the following aspects:
- Targeting Specific Antigens: Therapeutic antibodies are designed to bind to specific targets, such as tumor antigens, thereby marking them for destruction by the immune system.
- Reducing Side Effects: Since they are generally more specific than traditional treatments, the use of these antibodies can minimize damage to healthy tissues, leading to fewer side effects.
- Advantages over Conventional Therapies: In some cases, therapeutic antibodies have shown greater efficacy than older treatment methods. For example, Rituximab targets CD20 on B cells, providing effective treatment in certain lymphomas and leukemia.
However, there are challenges in this area. The development of resistance to therapeutic antibodies is an ongoing concern. Therefore, continuous research is necessary to improve efficacy and identify new targets.
"The evolution of monoclonal antibodies has transformed the landscape of modern medicine and represents a remarkable achievement in immunotherapy."
In summary, the implications of antibody production extend far beyond basic health. They are pivotal in vaccinations and therapeutic treatments, influencing both individual patient outcomes and public health policies. Consequently, continued research in this domain is essential for tackling future health challenges.
Challenges in Understanding Immunity
Immunity is a complex system with many variables. Understanding the challenges in this area is critical for advancing research and improving therapeutic strategies. Each aspect of antibody production involves intricate interactions among various immune cells, which can sometimes lead to confusion in interpretation and application. Exploring the nuances of immune disorders can help clarify these dynamics.
Autoimmune Disorders and Antibodies
Autoimmune disorders represent a significant challenge in understanding immunity. In these conditions, the immune system mistakenly targets the body’s own cells, leading to the production of antibodies against self-antigens. Common examples include rheumatoid arthritis, lupus, and multiple sclerosis.
The mechanisms behind these disorders involve B cells that produce autoantibodies. These autoantibodies can trigger inflammation and tissue damage, complicating therapeutic approaches. Additionally, cytokines and T cells play a regulatory role, influencing the degree of autoimmune responses.
Research has shown that genetic predisposition and environmental triggers contribute to the development of these disorders. When B cells undergo activation without proper control, this can result in excessive antibody production—a hallmark of autoimmune conditions.
HIV and Antibody Production
The human immunodeficiency virus (HIV) poses another significant challenge in understanding immune responses. HIV targets CD4+ T cells, which are crucial for orchestrating the immune response, including the activation of B cells in producing antibodies. As the virus replicates, it diminishes the functionality of these immune cells.
This impairment greatly affects the body’s ability to produce effective antibodies against HIV. While the immune system does generate antibodies, these may not always neutralize the virus effectively. The rapid mutation of the virus also results in diverse strains, making natural immunity difficult to achieve.
This failure to produce effective antibodies underlines the complexity of developing vaccines against HIV, as acquiring long-lasting immunity has remained a formidable goal.
Future Directions in Immunological Research
The field of immunology is evolving rapidly. Researchers are continuously exploring how immune cells contribute to antibody production and how these processes can be harnessed for medical applications. Recognizing the current trajectory of immunological research is essential for understanding the future landscape of healthcare. As we identify the intricacies involved in humoral immunity, it becomes evident that targeted research can lead to breakthroughs in various areas, including vaccine development and therapeutic strategies.
Advancements in Vaccine Development
Vaccination is one of the most effective ways to prevent infectious diseases. Recent advancements have emphasized the significance of immune cells in generating a robust antibody response. Researchers are exploring novel vaccine platforms, such as mRNA vaccines, which have shown promise in generating specific antibody responses quickly.
The efficacy of vaccines, in part, depends on how well B cells and T cells interact after vaccination. By improving our understanding of these interactions, scientists aim to develop vaccines that provide long-lasting protection with fewer doses.
Factors influencing vaccine responses, such as age, genetic background, and pre-existing immunity, are also under investigation. Personalized vaccines that take these variables into account have the potential to improve outcomes significantly.
Personalized Medicine and Immunology
Personalized medicine tailors treatment based on individual patient characteristics. In immunology, this approach can enhance antibody production tailored to a person's unique immune profile. Through genomic and proteomic technologies, researchers can identify which immune cells are present and how they respond to specific pathogens. This knowledge facilitates the design of targeted therapies that can enhance the body's natural antibody production.
Furthermore, the role of biomarkers in understanding immune responses is becoming more prominent. Identifying these biomarkers could lead to precise interventions aimed at modulating immune activity effectively.
"The next wave of immunological research holds potential not only for therapeutic interventions but also for redefining our understanding of health itself."
As we examine these future directions, it is clear that the integration of innovative scientific approaches will play a crucial role in enhancing our understanding of the immune system and how immune cells contribute to antibody production.