Immunofluorescence Staining: Key Insights and Developments
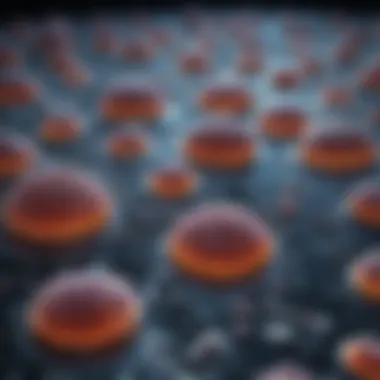
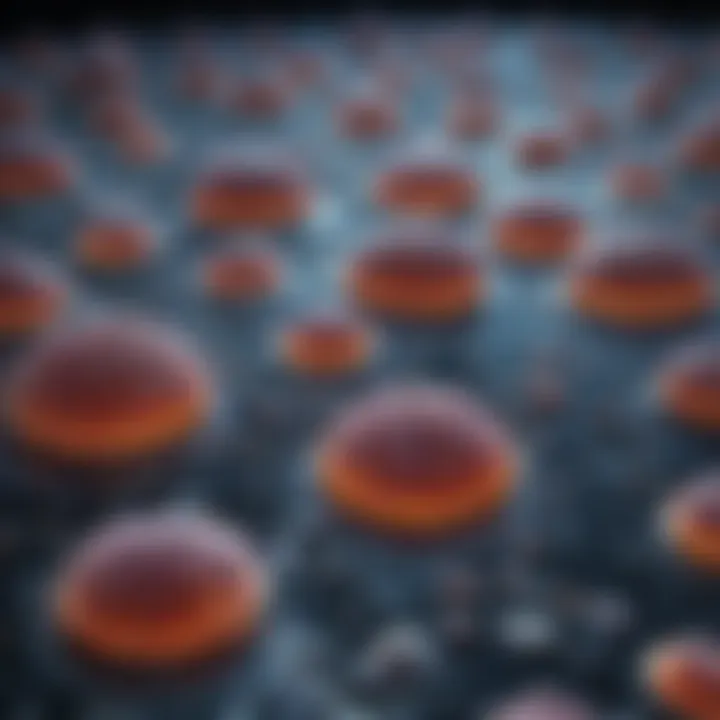
Intro
Immunofluorescence staining is a cornerstone in the toolkit of cellular biology, enabling the visualization of proteins within cells. In today’s scientific landscape, understanding the transport and interactions of proteins is crucial for a wide array of research fields, including cancer biology, neuroscience, and immunology. As scientists peel back the layers of cellular functions, immunofluorescence serves as a powerful lens, allowing for clearer insights into the roles specific proteins play in health and disease.
This article embarks on a journey through immunofluorescence staining, discussing its foundational principles, diverse applications, and the promising tools and technologies that lie on the horizon. With a blend of technical insights and practical implications, readers will gain a robust understanding of how this technique continues to shape modern biology and opens pathways for innovative research.
Research Overview
Immunofluorescence staining hinges on the principle that specific antibodies can bind to targeted antigens in biological samples, such as tissue sections or cell cultures. This binding produces a fluorescent signal, usually visible under a fluorescence microscope, allowing scientists to pinpoint the location of proteins. Two major methodologies dominate this area: direct staining and indirect staining.
Methodological Approaches
Direct staining employs antibodies that are conjugated to a fluorescent dye. This straightforward method allows for rapid results but may lack sensitivity when targeting low-abundance proteins.
Indirect staining, inversely, utilizes a two-step process. Initially, a primary antibody binds to the target antigen. Subsequently, a secondary antibody conjugated with a fluorescent dye binds to the primary antibody. This method significantly amplifies the signal, making it easier to visualize proteins, even in low concentrations.
Significance and Implications
The implications of immunofluorescence staining stretch across numerous scientific realms. For instance, in oncology, it assists in identifying tumor markers that guide therapeutic decisions. In neuroscience, it helps to unravel the complexities of synaptic interactions. Beyond individual disciplines, the method showcases interdisciplinary synergy, merging aspects of chemistry, biology, and medical science.
"Immunofluorescence staining is not just a technique; it’s a bridge connecting molecular biology with clinical applications."
Current Trends in Science
As we advance, several currents within the field highlight the innovation and efficacy of immunofluorescence staining. New techniques are emerging, paired with enhancements in imaging technology, which not only improve visual resolution but also reduce background noise.
Innovative Techniques and Tools
Recent developments include super-resolution microscopy and multiplexing techniques, enabling simultaneous examination of multiple proteins within a single sample. These innovations allow researchers to draw intricate maps of cellular environments, revealing interplay between various biomolecules. Moreover, proximity ligation assays have broadened the scope of immunofluorescence by allowing for the detection of protein-protein interactions. These advancements signal a shift towards more detailed and accurate representations of cellular functions.
Interdisciplinary Connections
The relationship between immunofluorescence staining and fields like bioinformatics, systems biology, and materials science is becoming ever more pronounced. For example, bioinformatics tools are utilized to analyze large datasets generated by high-throughput imaging, transforming raw data into actionable insights. Likewise, collaborations with materials scientists have led to the development of novel fluorophores that enhance the clarity and brightness of images.
Prelude to Immunofluorescence Staining
Immunofluorescence staining has carved its niche in the realm of cellular biology. This technique, pivotal for visualizing specific proteins within cells, plays a fundamental role in bridging the gap between various scientific disciplines. Whether one is delving into disease mechanisms, neural pathways, or developmental processes, the ability to pinpoint the location of proteins is invaluable. It gives clarity to complex biological systems and allows for a deeper understanding of various processes that govern life.
One might wonder why the understanding of this technique is so crucial. As science advances, more intricate questions arise regarding cellular function, signaling pathways, and disease pathology. Immunofluorescence staining offers researchers a window into these biological mysteries. Through the application of specific antibodies that bind to target proteins, scientists can not only confirm the presence of these molecules but also discern their spatial distribution within cellular structures. Hence, the significance of this method cannot be overstated.
Historical Context
The roots of immunofluorescence staining run deep in the annals of scientific history. It all began in the mid-20th century, when researchers started to experiment with antibodies marked by fluorescent dyes. The groundbreaking work of Coons et al. in the 1940s laid the groundwork for what would become a standard technique in laboratories worldwide. Their pioneering experiments, primarily focused on tissue sections, showcased the power of using fluorescent labels to visualize antigens within tissues.
As research progressed through the decades, the technique underwent refinements. By the late 20th century, advancements in microscopy and the synthesis of improved fluorescent dyes had made this method more accessible and versatile. Specificity of antibodies also improved, reducing the instances of cross-reactivity and enhancing the clarity of results. Each innovation built upon the last, allowing immunofluorescence staining to become a cornerstone in both clinical diagnostics and research.
Significance in Cellular Biology
In the landscape of cellular biology, immunofluorescence staining stands out as an irreplaceable tool. Its significance lies in several critical aspects:
- Localization of Proteins: The primary strength of this technique is its ability to reveal the precise location of proteins within cells. This spatial information helps elucidate protein functions and interactions in various biological contexts.
- Disease Mechanisms: Often, studying the distribution of proteins provides insights into pathological conditions. For instance, the mislocalization of certain proteins can be a hallmark of diseases like cancer, making immunofluorescence crucial for understanding these disorders.
- Developmental Studies: In developmental biology, tracking protein expression patterns at different growth stages aids in comprehending cell differentiation processes. Thus, the role of immunofluorescence in frontline research is monumental.
Fundamental Principles
The fundamental principles of immunofluorescence staining are crucial. They provide the basis for understanding how this technique works, enabling researchers to visualize proteins within cells effectively. Knowledge of these principles supports a wide range of applications, from pathology to developmental biology, underscoring the flexibility and functionality of the method. The underlying mechanisms, choice of fluorophores, and microscopy techniques used are key components that impact results significantly.
Mechanism of Fluorescent Labeling
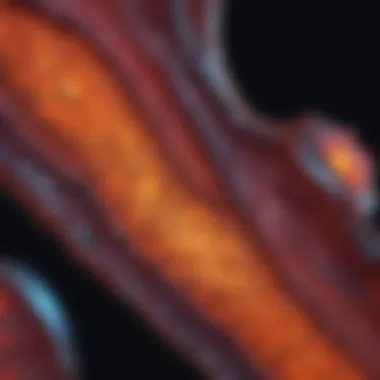
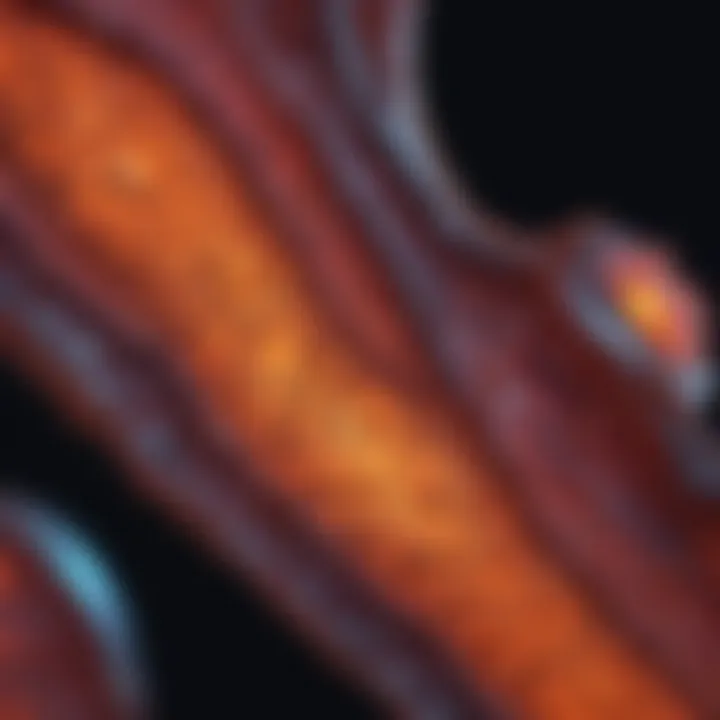
Fluorescent labeling involves attaching a fluorescent dye or label to antibodies that specifically bind to target proteins. The process starts with the preparation of a sample, usually involving fixation and permeabilization. Once prepared, fluorescently labeled antibodies are introduced to the sample. These antibodies bind to their specific antigens, allowing researchers to detect and visualize the proteins of interest. The magic happens when these bound antibodies are exposed to light of a specific wavelength—this excites the fluorophores, causing them to emit light at a longer wavelength. This principle of fluorescent energy transfer is pivotal as it allows for the identification of proteins through fluorescence microscopy.
Types of Fluorophores
Fluorophores are chemical compounds that emit light upon excitation, and their selection is vital in immunofluorescence. Various types of fluorophores, such as fluorescein isothiocyanate (FITC) and rhodamine, are frequently used. Each different fluorophore has its characteristics, including excitation and emission wavelengths. When selecting a fluorophore, factors like brightness, photostability, and potential for spectral overlap must be considered. The choice of the right fluorophore can significantly enhance the clarity and quality of the results in an experiment. Thus, understanding the properties and usage of different fluorophores is essential in immunofluorescence staining.
Fluorescence Microscopy Techniques
Fluorescence microscopy techniques are the means through which the results of immunofluorescence staining are visualized. Each technique offers unique advantages and specialties that cater to different research needs. The choice of microscopy affects the resolution and clarity of the images obtained. The primary categories include wide-field microscopy, confocal microscopy, and super-resolution microscopy. Each has its own characteristics that determine its effectiveness for particular applications.
Wide-field Microscopy
Wide-field microscopy is one of the simplest forms and is widely used due to its straightforward setup and ease of use. It illuminates the entire sample at once, which leads to a high signal but can come with background noise. This method is particularly beneficial for large area scans where the overall view of multiple cells is necessary. A significant advantage of wide-field microscopy is its rapid image acquisition, making it effective for observing dynamic processes in real-time. However, the downside is that the illumination of the entire field can result in poor optical sectioning, which may obscure details in three-dimensional samples, leading to decreased resolution in some instances.
Confocal Microscopy
Confocal microscopy offers better resolution and contrast by using a focused laser beam to scan the sample point by point. This technology allows for optical sectioning, enabling researchers to capture images of specific layers within thicker samples. The main appeal of confocal microscopy is its ability to produce high-quality, detailed images while reducing background noise. This method is highly favored in studies requiring precise localization of proteins, as it can selectively illuminate a specific focal plane. Nevertheless, it requires more extended acquisition times and more complex equipment, which may limit its application in some fast-paced experimental contexts.
Super-resolution Microscopy
Super-resolution microscopy is an advanced technique that pushes the resolution limits beyond what traditional optical methods can achieve. By employing specific principles, such as stimulated emission depletion (STED) or localized surface plasmon resonance (LSPR), this method can visualize structures at the nanometer scale. It is beneficial when studying intricate cellular structures that require exceptional detail, offering insights that were once unattainable. However, it also comes with considerable complexity and demands high levels of technical expertise. Additionally, due to its sophistication, the equipment and operational costs can be somewhat prohibitive.
In summary, the fundamental principles of immunofluorescence staining encompass a technical understanding of fluorescent labeling, the careful selection of fluorophores, and the application of different microscopy techniques. The choice of approach influences not only the clarity and quality of the results but also opens new avenues for scientific inquiry.
Techniques of Immunofluorescence
Immunofluorescence plays a pivotal role in cellular biology research, providing researchers with an ability to visualize specific proteins within their cellular context. The various techniques of immunofluorescence are crucial as they allow for flexibility and specificity in experimental design. Understanding these techniques enhances their applications in disciplines like pathology, neuroscience, and cancer research. Each technique offers unique advantages and challenges that can significantly affect the outcomes of an experiment.
Direct Immunofluorescence Staining
Direct immunofluorescence staining represents one of the more straightforward approaches in this methodology. In this technique, a fluorophore-labeled antibody binds directly to the target antigen within the sample. This direct interaction simplifies the staining process and can produce clearer images, which makes it especially useful for detecting surface proteins.
- Advantages:
- Considerations:
- Simplicity in the protocol
- Shorter incubation times
- Reduced background staining
- Limited versatility, as it requires highly specific antibodies
- Lower sensitivity compared to indirect staining
The ease of use makes it a suitable choice for many types of experiments. However, achieving high specificity can sometimes be a double-edged sword, depending on the quality of the labeled antibody used.
Indirect Immunofluorescence Staining
On the other hand, indirect immunofluorescence is a more intricate approach that utilizes two antibodies. The primary antibody, which is specific to the target antigen, does not carry a label. Instead, it is detected by a secondary antibody, which is labeled with a fluorophore. This method has gained traction due to its increased sensitivity and versatility.
- Advantages:
- Considerations:
- Amplified signal due to multiple secondary antibodies binding to one primary antibody
- Greater flexibility in antibody selection
- Longer protocol and more steps, which might introduce variability
- Potential for cross-reactivity between antibodies
The ability to amplify the signal can lead to discovering proteins at lower abundance, making indirect staining a preferred choice in a vast number of research scenarios.
Multicolor Staining Approaches
Multicolor staining approaches stand out in immunofluorescence by allowing the concurrent visualization of multiple antigens within a single sample. This technique employs various fluorophores, each excited by different wavelengths of light, enabling the detection of several targets at once.
Incorporating multicolor techniques can provide a richer, more detailed landscape of cellular interactions and localization.
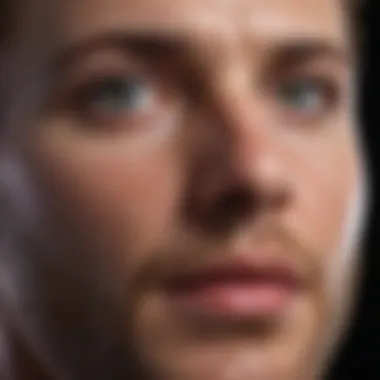
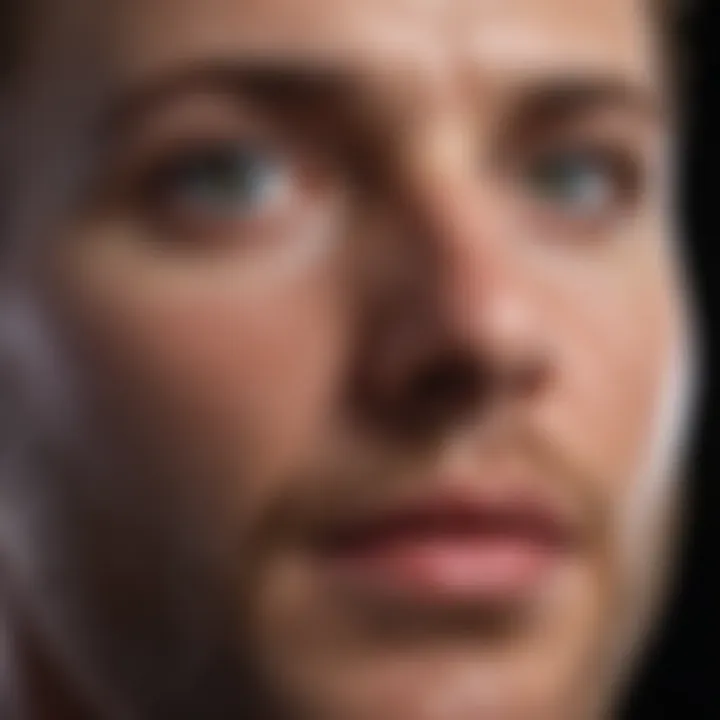
- Advantages:
- Considerations:
- Comprehensive data on protein interactions and colocalization
- High-throughput capabilities
- Complexity in selecting compatible fluorophores
- Increased potential for spectral overlap and fading
Using multicolor staining, researchers can gain insights into the intricate workings of cellular functions and mechanisms. Moreover, this technique can help in understanding interactions between proteins during different cellular processes.
Applications in Research
The realm of research has seen a significant leap forward due to the advent of immunofluorescence staining. This technique allows researchers to visualize proteins within cells, woven into the tapestry of cellular processes. By utilizing fluorescent markers attached to antibodies, scientists can pinpoint the exact location and quantity of specific proteins in tissues and cells. This capability is vital for understanding biological functions and disease mechanisms.
Research employs immunofluorescence staining across multiple disciplines, each reaping distinct advantages like enhanced diagnostic precision, an understanding of molecular pathways, and insights into the intricate interactions within cells. Below, we explore some key research applications.
Cellular Pathology
In cellular pathology, immunofluorescence is a cornerstone technique. It sheds light on abnormal cellular structures that characterize various diseases, such as autoimmune disorders or infections.
- Abnormal Protein Expression: Researchers utilize immunofluorescence to ascertain the expression of proteins that may indicate disease progression, allowing for a tailored therapeutic approach.
- Diagnostic Tool: The precision of this technique aligns with clinical needs for rapid and accurate diagnosis. For instance, in systemic lupus erythematosus, detection of autoantibodies through immunofluorescence can help elucidate the disease state.
- Visualizing Structural Abnormalities: The method can highlight morphological changes in cells, which can be indicative of neoplastic transformations.
Neuroscience Studies
Neuroscience is another field where immunofluorescence shines brightly. It helps researchers map neural pathways and understand brain functions at a molecular level.
- Mapping Neuronal Networks: By tagging specific proteins like neurotransmitter receptors with fluorophores, scientists can visualize how neurons communicate.
- Studying Neurodegenerative Diseases: Immunofluorescence assists in studying conditions such as Alzheimer's, aiding in the detection of amyloid plaques and tau tangles within neuronal cells.
- Investigating Synaptic Function: This technique allows for the examination of synaptic markers, shedding light on synaptic health and plasticity which has implications in learning and memory.
Cancer Research
In cancer research, immunofluorescence offers crucial insights into tumor biology, providing a pathway to understand cancer progression, therapeutic resistance, and potential vulnerabilities.
- Tumor Microenvironment Analysis: By assessing the localization of immune cells in tumors, researchers can gauge the immune response to cancer, ultimately directing immunotherapy strategies.
- Biomarker Discovery: Identification of specific biomarkers through immunofluorescence can predict patient outcomes or responses to therapy, which is instrumental for personalized medicine.
- Studying Cell Cycle Dynamics: This technique enables the observation of protein expressions during different stages of cell division, offering insights into tumor progression and potential therapeutic targets.
Developmental Biology
Developmental biology benefits immensely from immunofluorescence staining, revealing the complexities of cellular differentiation and development.
- Studying Embryonic Development: By identifying cell type-specific markers in embryos, researchers can trace cell lineages and study developmental processes.
- Analyzing Tissue Development and Repair: The technique highlights how specific interactions between proteins drive tissue development and how those processes may falter in diseases.
- Addressing Stem Cell Research: Immunofluorescence provides insights into stem cell differentiation, which is key in regenerative medicine and tissue engineering.
In summary, the breadth of applications for immunofluorescence staining in research highlights its indispensable role in modern science. By bridging gaps in our understanding of disease mechanisms, it paves the way for future advancements in diagnosis and treatment.
Challenges in Immunofluorescence Staining
Immunofluorescence staining, while an invaluable technique in cellular biology, is not without its hurdles. Understanding these challenges is critical for researchers and practitioners who depend on accurate and reliable results. By shedding light on the obstacles encountered during this staining process, one can better appreciate its complexities and limitations. The implications of these challenges are profound, influencing results, reproducibility, and ultimately, the advancement of scientific knowledge.
Antibody Specificity and Cross-reactivity
One of the primary issues in immunofluorescence staining is antibody specificity. The effectiveness of the staining hinges on the ability of antibodies to bind selectively to their target antigens. However, cross-reactivity can muddy the waters. Antibodies may latch onto unintended proteins, leading to false positives. For instance, if an antibody meant for one type of keratin inadvertently binds to another, the resulting fluorescence might suggest protein presence where there is none. This not only complicates interpretation but also risks misinforming subsequent research outcomes.
To minimize these misinterpretations, it is vital to thoroughly validate antibodies before usage. Checking the manufacturer's datasheet for specificity claims, exploring peer-reviewed studies that employ the same antibodies, and conducting preliminary tests can provide insights into potential pitfalls. It's a painstaking process but one that pays off in clarity.
Fluorophore Stability
Another aspect that looms large in immunofluorescence is fluorophore stability. These fluorescent dyes are sensitive to environmental factors such as light, temperature, and even the pH of the sample preparation. A fluorophore that photographs beautifully in optimal conditions might degrade rapidly, turning your brilliant Saturday night plans at the microscope into a disappointing series of dim or shadowy images. Therefore, researchers must handle and store these probes with care.
Additionally, choosing the right fluorophore is critical. While certain dyes may provide vibrant signals, their susceptibility to photobleaching can lead to fading during extended imaging sessions. For example, some engineered fluorophores show resilience against bleaching, enabling better results in long-term studies. Careful selection based on experimental design is crucial for successful outcomes.
Sample Preparation Issues
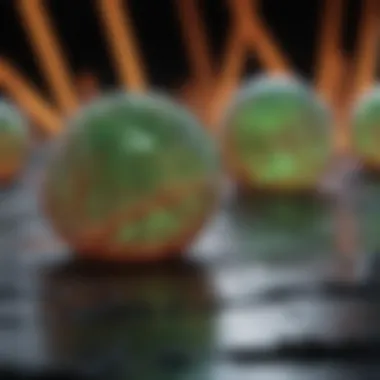
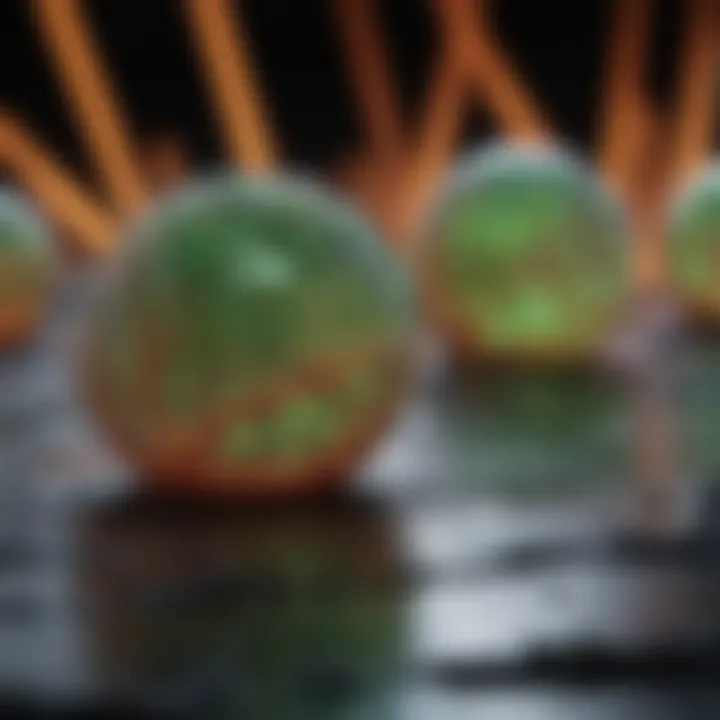
Last, but definitely not least, are the sample preparation issues associated with immunofluorescence. The success of this technique begins long before one even touches the microscope. Proper tissue fixation, for example, is essential. Insufficient fixation may compromise the integrity of the proteins and lead to unreliable results. If a tissue sample is not permeabilized correctly, antibodies may have difficulty entering the cells to bind their targets, further complicating matters.
Furthermore, sample characteristics—such as thickness, type of cells or tissues, and even their inherent autofluorescence—can affect the quality of imaging. It’s not uncommon for researchers to find themselves facing unexpected noise in their images due to high autofluorescence from certain tissues, thereby clouding the true signal. This necessitates an understanding of how to properly handle and prepare numerous sample types for effective results.
"Addressing these challenges head-on is key to maximizing the potential of immunofluorescence staining.”
In summary, while immunofluorescence staining is a powerful tool in the scientist's arsenal, challenges like antibody specificity, fluorophore stability, and sample preparation must be navigated with vigilance. Recognizing and addressing these hurdles will ultimately enhance the reliability of results and pave the way for significant advancements in the field of cellular biology.
Future Directions in Immunofluorescence
The field of immunofluorescence staining is really at an exciting crossroads right now. As researchers are constantly pushing the limits of what’s possible, there’s a need to look ahead at how this method can evolve. The future directions not only promise enhanced accuracy and efficiency but also open the door to conclusions that could change our understanding of biology and diseases. High-throughput capabilities, integration with genomic techniques, and advancements in fluorescent probes stand out as key areas that can dramatically shift the landscape of cellular visualization.
Advancements in Fluorescent Probes
New fluorescence probes are emerging, significantly improving the sensitivity and specificity of immunofluorescence staining. These advanced probes often boast brighter emissions, higher photostability, and longer wavelengths, which help in minimizing overlap in multicolor applications. A particularly promising development is the use of nanoparticles that can bind to multiple targets, allowing for a multifaceted view of cellular components in a single experiment.
It’s not just about being flashy (literally) — the development of fluorescent probes aimed at specific cellular structures or behaviors means researchers can unravel complex biological pathways with greater insight. The durability of these new stains ensures that slides maintain their quality even through prolonged imaging sessions.
Integration with Other Techniques
Integrating immunofluorescence with other cutting-edge technologies can greatly expand its utility and applicability.
Combined Staining with Genomic Techniques
One of the standout features of combining immunofluorescence with genomic techniques is the ability to correlate protein expression with genetic data. This dual approach allows scientists to visualize how specific proteins relate to gene expression in various conditions. Using methods like RNA in situ hybridization in conjunction with immunofluorescence enables a rich interrogation of cellular functions, particularly in complex frameworks like tumors where cellular heterogeneity is pronounced.
The benefit of this combined staining method is profound; it creates a multifaceted picture that traditional methods can’t match. However, it can be trickier to establish proper protocols, meaning practical limitations might temper its immediate adoption.
Synergistic Use with Electron Microscopy
Integrating immunofluorescence with electron microscopy is another game changer. It provides a way to further enhance the resolution of biological images beyond the capabilities of conventional fluorescence microscopy. The high-resolution details revealed through electron microscopy can validate and complement the fluorescent results. This complementary technique not only improves spatial resolution but can also allow researchers to assess the localization of proteins at nearly atomic resolution.
While electron microscopy adds significant value, it does come with logistical hurdles, such as the need for complex sample preparation and the potential for longer processing times. Moreover, balancing the two requires a thoughtful approach to ensure that antibody labeling doesn’t interfere with electron-dense contrast agents.
Automation and High-throughput Screening
As with many areas in research, automation is a key focus for the future of immunofluorescence. High-throughput systems are simplifying the process, enabling researchers to perform numerous experiments in one go. This not only saves time but also reduces variability between experiments, thus improving reliability.
With advances in imaging technology and analysis software, it’s becoming easier to process and interpret large datasets resulting from high-throughput approaches. Researchers can now generate insights at a scale that wasn’t feasible before.
Finale
In the realm of cellular biology, immunofluorescence staining represents a cornerstone technique, contributing significantly to the understanding of various biological processes. This method not only provides a window into the cellular architecture but it also allows researchers to pinpoint the precise localization of proteins, which is invaluable in elucidating cellular functions and behaviors.
Summary of Key Insights
Throughout this article, we've navigated through the intricate layers of immunofluorescence staining, uncovering its principles and methodologies. The main points include:
- The fundamental mechanics behind fluorescent labeling and the diversity of fluorophores used.
- The distinction between direct and indirect staining approaches and their respective advantages.
- The various microscopy techniques that enhance visualization, including wide-field, confocal, and super-resolution microscopy.
- Key applications of this technique spanning cellular pathology, neuroscience, cancer research, and developmental biology.
- Challenges such as antibody specificity, stability of fluorophores, and issues in sample preparation.
- Future directions indicating advancements in fluorescent probes, integration with genomic techniques, and automation in high-throughput screening.
By highlighting these aspects, we reaffirm the significance of immunofluorescence staining as a pivotal tool in modern biological research.
The Role of Immunofluorescence in Scientific Advancement
Immunofluorescence staining has not only enhanced our comprehension of cellular mechanisms but has also catalyzed advancements across various scientific realms. The ability to visualize cellular components in situ has proven essential in clinical diagnostics, leading to early detection of diseases such as cancer and autoimmune disorders. Furthermore, its role in fundamental research offers insights that drive innovations in therapeutic interventions and drug development.
An important note is that as the field progresses, the continual optimization of techniques and technologies will undoubtedly lead to new discoveries. The blending of immunofluorescence with other methodologies, such as genomics and electron microscopy, will further enhance the resolution and depth of our analyses, painting an even clearer picture of biological phenomena.
As we gaze into the future, it's clear that immunofluorescence will remain at the forefront of cellular biology, providing powerful avenues for exploration and understanding.
"The key to unlocking the mysteries of life lies in the details that immunofluorescence staining reveals."
In sum, immunofluorescence is not merely a technique but a gateway to scientific inquiry that continues to push the boundaries of what we know about life at the cellular level.