Exploring the Impact of Laser Technology Across Scientific Disciplines
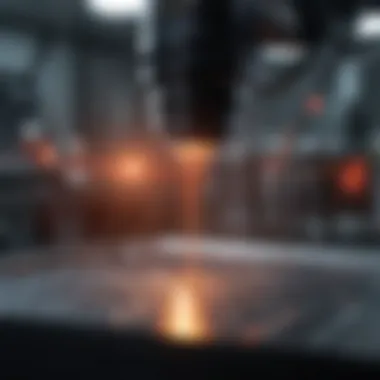
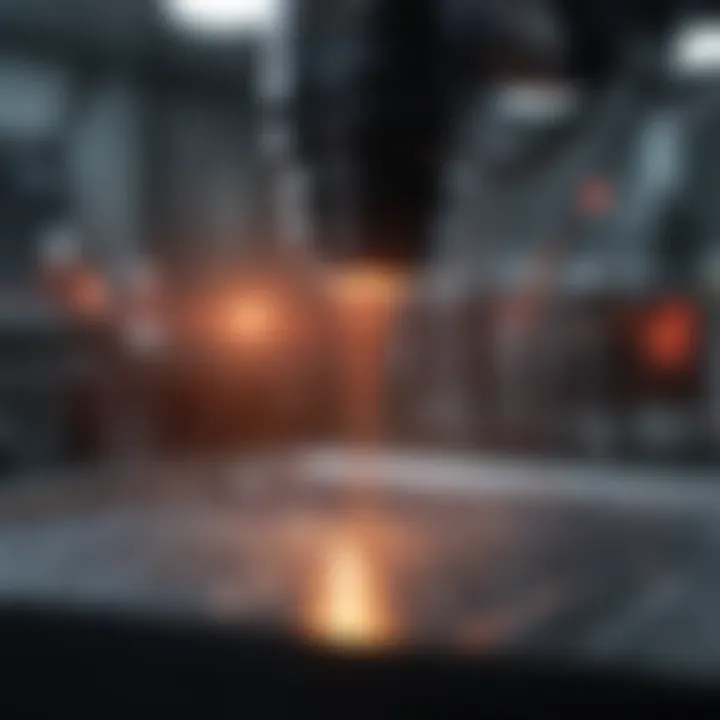
Intro
Laser technology has become a cornerstone in various scientific fields, transforming how research is conducted and the types of discoveries that can be made. This article explores the extensive applications of lasers in biology, chemistry, physics, and earth sciences. By delving into the principles that govern laser operation and tracing its historical development, we aim to show how this technology fundamentally alters methodologies and enhances precision in research.
In discussions of scientific innovation, comprehension of laser technology is not merely academic; it holds profound implications for practical applications. The integration of lasers into research practices reveals insights that accessible to both specialized and broader audiences, making this inquiry relevant and pressing in today’s scientific landscape.
Research Overview
Methodological Approaches
The utilization of laser technology in scientific research involves varied methodological approaches. These typically include:
- Spectroscopy: Lasers facilitate detailed analysis of material compositions through techniques like Raman spectroscopy and laser-induced breakdown spectroscopy.
- Imaging: In biology, lasers improve imaging techniques, enhancing contrast and resolution in microscopy applications.
- Measurements: In physics, lasers provide highly accurate distance and speed measurements, crucial for experiments requiring precision.
Each method showcased how lasers contribute uniquely to the scientific discourse across multiple disciplines, allowing researchers to test and confirm hypotheses with enhanced accuracy and reliability.
Significance and Implications
The implications of laser technology extend beyond individual disciplines; it fosters interdisciplinary cooperation. By developing tools that can adapt across various fields, lasers drive forward collaborative research that leads to comprehensive solutions to complex scientific problems. This shared advancement continues to motivate scientists to explore new areas where laser applications can yield significant benefits.
"Lasers have reshaped our understanding and approaches to measurement, analysis, and visualization in science. Their versatility is only matched by their power."
Current Trends in Science
Innovative Techniques and Tools
The emergence of new laser technologies has led to innovative techniques reshaping research landscapes. Some notable advancements include:
- Ultrafast Lasers: Allowing scientists to observe molecular processes that were previously too rapid to capture.
- Laser-Guided Surgery: Revolutionizing precision medicine by enhancing surgical techniques in medical practice.
- Laser Plasma Accelerators: Offering promising developments in particle physics, demonstrating the future of feasible particle acceleration.
These innovations are not just theoretical; they have practical implications in lab settings and beyond, stimulating advancements across disciplines.
Interdisciplinary Connections
The connections between various fields facilitated by laser technology cannot be overstated. For instance, the convergence of laser technology and nanotechnology has led to significant progress in material sciences, bioengineering, and even renewable energy sources. Collaboration between chemists and biologists using laser-based techniques has advanced understanding in drug delivery systems, where precision in targeting mechanisms is crucial.
In summary, laser technology's transformative effects have integrated deeply into scientific research methodologies. Its continual evolution and adaptability promise to significantly influence future scientific discoveries and practices.
Now, as we further explore specific case studies in the subsequent sections, we will witness firsthand how laser technology is fundamentally reshaping various scientific disciplines for both immediate and long-term advancements.
Prologue to Laser Technology
Laser technology has become a cornerstone in various scientific disciplines, including biology, chemistry, physics, and earth sciences. Understanding lasers goes beyond knowing they produce light; it encompasses their capabilities and applications that reshape how researchers approach scientific questions. The importance of this topic lies not only in the innovative methodologies developed but also in the profound impacts on practical applications.
One benefit of laser technology is its precision, allowing for enhanced measurement techniques and experimental designs. This precision is critical in areas such as medicine, where lasers can target tissues without damaging surrounding structures. Consideration of laser safety and handling is also paramount. Researchers must be aware of the risks associated, including eye injury from direct laser exposure. Therefore, this introduction sets the stage for better comprehension of how lasers operate and their historical context.
Furthermore, understanding the principles of laser operation is vital. The foundation of laser technology informs how it has evolved and continues to develop. This section will explore the definition of lasers, their characteristics, historical advancements, and the fundamental principles underlying their operation.
Definition and Characteristics of Lasers
A laser, or Light Amplification by Stimulated Emission of Radiation, is a device that generates an intense beam of coherent light. Unlike ordinary light sources, lasers emit light that is both monochromatic and directional. Monochromatic means that the light consists of a single wavelength, while directional indicates that the light is emitted in a narrow beam.
Key characteristics include:
- Coherence: The light waves emitted are in phase, which allows for high precision in applications like holography.
- Monochromaticity: This quality enables lasers to produce specific wavelengths, making them useful in spectroscopy.
- Directionality: Laser beams can travel long distances without scattering, useful for communication technology.
These characteristics set lasers apart from traditional light sources and underline their unique utility in various research fields.
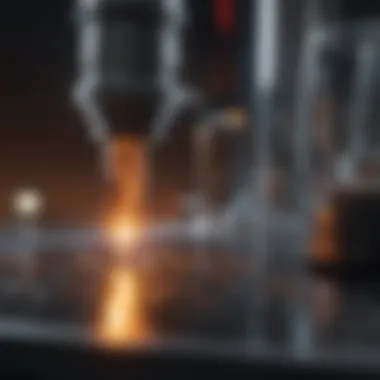
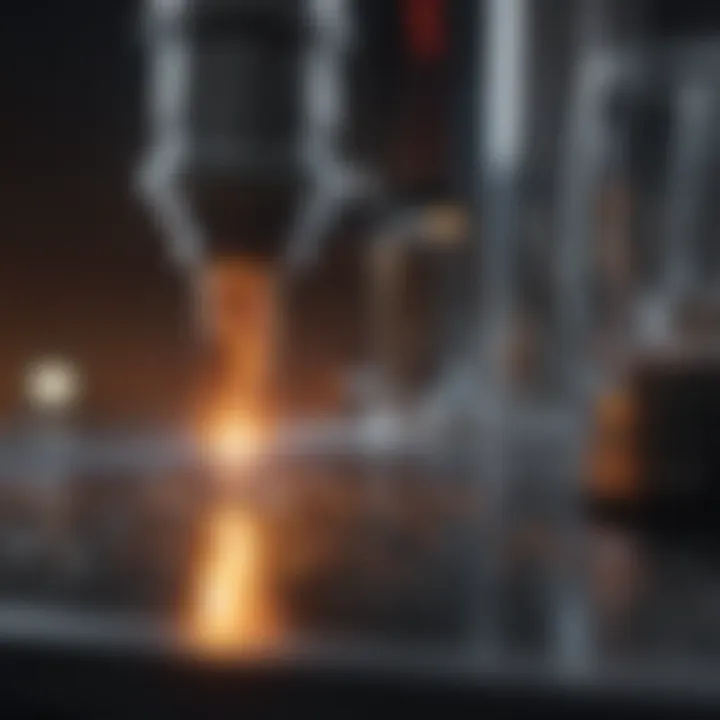
Historical Development of Laser Technology
The journey of laser technology began in the 20th century. The concept of stimulated emission was proposed by Albert Einstein in 1917 but took decades for practical application. The first working laser, the ruby laser, was created by Theodore Maiman in 1960. This marked a significant milestone, demonstrating the feasibility of generating coherent light.
Subsequent developments included:
- Semiconductor Lasers: These became pivotal in telecommunications, enhancing data transfer rates.
- Fiber Lasers: Offering increased efficiency and versatility, fiber lasers have applications in materials processing and medical fields.
Each advancement has contributed to the broader applications of lasers today, impacting areas ranging from scientific research to everyday technology.
Fundamental Principles of Laser Operation
At its core, a laser operates by stimulating atoms to emit light. The fundamental processes involved include:
- Excitation: Atoms in a medium absorb energy, elevating their electrons to a higher energy state.
- Spontaneous Emission: An excited atom inevitably transitions back to its lower energy state, emitting a photon spontaneously.
- Stimulated Emission: If a photon of the right energy passes nearby, it can cause the excited atom to drop to a lower energy state, emitting another identical photon. This is what generates coherent light.
The combination of mirrors surrounding the gain medium amplifies the light produced by stimulated emission, resulting in the output beam. Understanding these principles is essential for researchers aiming to utilize lasers effectively across diverse fields.
Applications of Lasers in Biology
The significance of lasers within the biological sciences cannot be overstated. These technologies provide a range of tools that enhance the resolution and sensitivity in various experimental approaches. In fact, laser applications have led to precision that was previously unattainable, allowing researchers to explore biological phenomena at unprecedented levels. This section will explore critical elements such as laser-based techniques in molecular biology, their usage in medical procedures, and advancements in imaging technologies.
Laser-Based Techniques in Molecular Biology
Lasers are crucial in molecular biology, particularly in the study of biomolecular interactions and behaviors. One of the primary applications is laser-induced fluorescence. This method allows scientists to tag molecules with fluorescent markers and excite them with lasers. The emitted light can be detected, providing valuable data on the concentration and movement of biomolecules. This technique has made it easier to track changes in live cells, resulting in profound insights into cellular processes.
Another essential technique is laser capture microdissection. This method uses a focused laser beam to cut and isolate specific cells from a larger tissue sample. By selecting only the cells of interest, researchers can analyze genetic material, proteins, and other cellular components without contamination. This precision is valuable for studying diseases, including cancer, where specific cell types are pivotal. The ability to obtain pure samples from diverse tissues enhances the accuracy of subsequent analyses.
Use of Lasers in Medical Procedures
Medical laser applications have transformed patient care across various fields such as dermatology, ophthalmology, and surgery. Lasers are employed in procedures for their ability to perform targeted actions with minimal damage to surrounding tissues. For instance, lasers are used during LASIK eye surgery to reshape corneal tissue, improving vision outcomes with minimal recovery time.
In dermatology, lasers treat skin conditions effectively. They can remove tattoos, lesions, and hair through selective destruction of pigment while leaving surrounding skin unharmed. This precision is crucial for aesthetic applications, contributing to the cosmetic field’s growth.
Furthermore, lasers have a role in cancer treatment. Techniques such as photodynamic therapy employ lasers to activate photosensitizing agents that selectively destroy cancer cells. Such targeted approaches are part of a growing trend toward less invasive treatments.
Advancements in Laser Imaging Technologies
Advances in laser imaging technologies have revolutionized microscopy and diagnostic methods. Techniques like confocal microscopy utilize lasers to produce sharp images of biological samples in just multiple dimensions. This enhances the understanding of cellular structures in greater detail, leading to new discoveries in cellular function and disease mechanisms.
Another important advancement is the use of lasers in optical coherence tomography. This non-invasive imaging technique captures high-resolution images of tissues, proving invaluable in ophthalmology and cardiology. It allows clinicians to visualize internal structures without the need for invasive procedures, ultimately improving patient outcomes.
These innovations show how vital laser technology is to the evolving landscape of biological research and medical applications.
In summary, the applications of lasers in biology provide substantial benefits across molecular biology, medical procedures, and imaging technologies. They enhance precision, improve patient care, and enable groundbreaking discoveries. As technologies advance further, the influence of lasers in biology is likely to expand even more.
Lasers in Chemistry Research
The integration of laser technology into chemistry research has revolutionized the field, facilitating numerous groundbreaking studies and applications. Lasers enable precise measurements and allow for manipulation at the molecular level, which can yield significant insights into chemical processes. Their applications span various domains, from fundamental research to environmental protection, making them essential tools in modern chemistry.
Laser Spectroscopy: Principles and Applications
Laser spectroscopy involves the use of lasers to analyze the properties of substances through interactions between light and matter. This technique can identify chemical compounds and assess their concentrations with remarkable sensitivity and specificity.
One core principle of laser spectroscopy is the interaction of laser light with the electronic or vibrational states of molecules. In doing so, one can differentiate between various chemical species and even isotopes. Applications range from studying reaction dynamics to characterizing materials. Some commonly used laser spectroscopy techniques include:
- Raman Spectroscopy: This technique provides information about molecular vibrations and is widely used in characterizing materials.
- UV-Vis Spectroscopy: Employing ultraviolet and visible light, it allows for the analysis of electronic transitions.
- Fluorescence Spectroscopy: It detects light emitted by substances after being excited by a laser source.
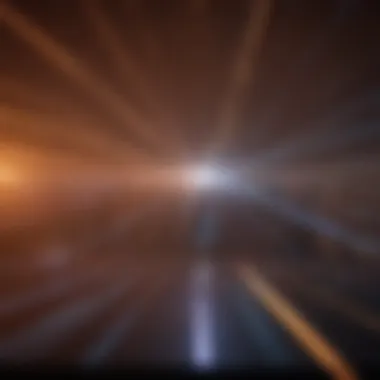
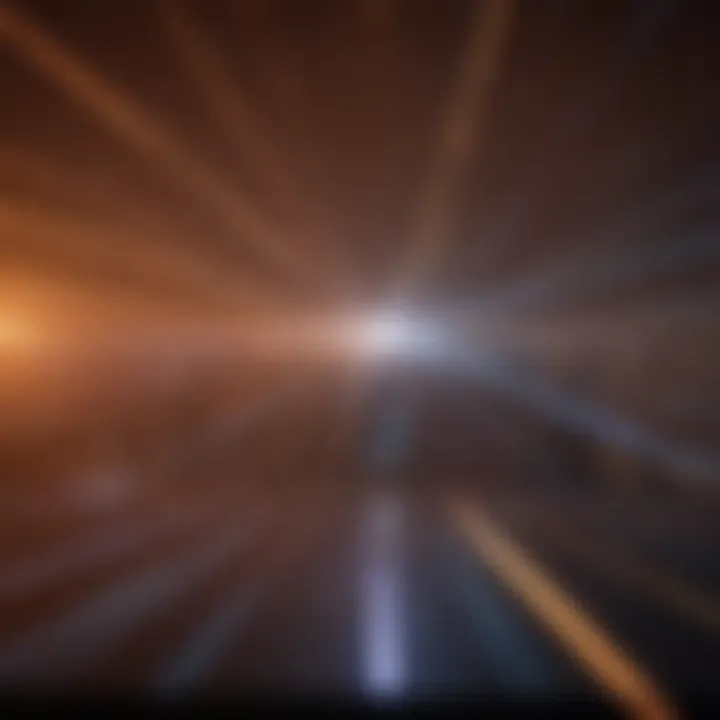
The sensitivity of laser spectroscopy leads to advancements in drug development, environmental monitoring, and quality control in chemical manufacturing.
Role of Lasers in Environmental Chemistry
Lasers play a vital role in environmental chemistry by facilitating the measurement of pollutants and monitoring environmental changes. Technologies like LIDAR (Light Detection and Ranging) use lasers to obtain precise measurements of atmospheric gases, aerosols, and land surfaces. This capability is crucial for analyzing the composition and quality of air, water, and soil.
Moreover, laser techniques contribute to:
- Remote Sensing: Allowing scientists to assess large areas without direct contact, these methods aid in monitoring deforestation, urbanization, and climatic changes.
- Chemical Detection: Lasers are used in devices that can detect trace levels of contaminants, ensuring public safety and environmental health.
By enhancing our understanding of chemical processes occurring in the environment, lasers enable better management and protection of natural resources.
Laser-Induced Reactions and Their Implications
Laser-induced reactions represent a fascinating area of research in chemistry. The focused energy from lasers can initiate chemical reactions under controlled conditions, leading to outcomes not attainable through traditional methods. This precision allows for exploration into various reaction pathways, stabilization of transient species, and even the synthesis of novel compounds.
Some implications of these reactions include:
- Enhanced Reaction Rates: By focusing energy directly on the reactants, lasers can increase reaction rates significantly.
- Selective Bond Cleavage: Lasers offer the ability to selectively break bonds in complex molecules, allowing chemists to devise new synthetic routes.
- Study of Fundamental Reaction Mechanisms: Understanding how molecules behave under laser excitation can provide insights into fundamental chemical processes.
The Role of Lasers in Physics
Lasers play a crucial role in the field of physics, serving as vital tools for both fundamental research and practical applications. Their ability to produce coherent light, which can be precisely controlled in terms of intensity, frequency, and polarization, makes lasers essential for exploring various physical phenomena. As researchers delve into the quantum realm, lasers facilitate experimentation that has pushed the boundaries of our understanding. The benefits of using lasers in physics are manifold; they can provide high-resolution measurements, allow precise manipulations of particles, and enable the observation of phenomena that were previously inaccessible.
Laser Technology in Fundamental Physics Research
Laser technology underpins a significant portion of fundamental research in physics. High-power lasers are used in experiments to study atomic and molecular interactions. For instance, lasers can be employed to cool atoms to near absolute zero, allowing scientists to observe unique states of matter, such as Bose-Einstein condensates. This state exhibits quantum effects on a macroscopic scale, presenting profound implications for the field of condensed matter physics.
Moreover, lasers facilitate advanced spectroscopic techniques, enabling researchers to analyze the energy levels of atoms and molecules with remarkable precision. This capability has implications not only for basic research but also for the development of new materials and technologies.
Applications in Quantum Physics
In quantum physics, lasers serve as a bridge between theory and experimental validation. They enable the manipulation of quantum states and allow researchers to investigate phenomena like quantum entanglement and quantum computing. For example, trapped ion quantum computers often utilize lasers to control the state of ions with high precision, which is critical for processing information.
Furthermore, the study of quantum optics—an area that investigates the quantum behavior of light—relies heavily on lasers. Techniques such as quantum key distribution for secure communication systems take advantage of laser properties, illustrating their essential role in both theoretical and applied quantum studies.
Innovations in Laser Sources and Their Impact
The evolution of laser technology has led to the development of various innovative laser sources. Solid-state lasers, fiber lasers, and semiconductor lasers have emerged, each offering distinct advantages. For instance, fiber lasers are known for their high efficiency and compact size, making them suitable for a wide range of applications, from telecommunications to industrial cutting processes.
Furthermore, advancements in ultrafast laser technology enable researchers to generate pulses of light lasting just a few femtoseconds. This innovation allows for the observation of rapid processes, such as chemical reactions or electron dynamics, in real time. By capturing these fleeting events, scientists can gain insights that enhance our understanding of physical laws.
In summary, lasers have transformed the landscape of physics research. Their precision, versatility, and ability to control light behavior position them as indispensable tools across various sub-disciplines of physics. As technology continues to advance, the potential for lasers to uncover new phenomena remains vast, promising to elevate both fundamental research and practical applications to new heights.
"Lasers unlock a realm of precision and control in physics that was once unimaginable, shaping the future of research and discovery."
Laser Applications in Earth Sciences
Laser technology plays a critical role in advancing our understanding of Earth Sciences. The precision and efficiency of lasers enable accurate measurements and observations that are vital in various subfields such as geology, meteorology, and environmental science. The benefits of laser applications in Earth Sciences include enhanced data collection, improved mapping techniques, and innovative monitoring systems that cater to the demands of modern scientific inquiry.
Remote Sensing Technologies Using Lasers
Remote sensing technologies powered by lasers offer a powerful means of collecting data from the Earth's surface and atmosphere without physical interaction. One prominent example of this technology is Lidar, which uses laser pulses to measure distances from aerial or satellite platforms. This method generates high-resolution topographic maps by precisely calculating the time it takes for laser light to return after hitting the ground.
The applications of Lidar are varied and far-reaching. For instance, it is employed to create detailed forest maps to assess biomass and monitor deforestation rates. Furthermore, Lidar's ability to penetrate vegetation allows researchers to study the underlying soil characteristics, contributing significantly to land use planning and management decisions.
Laser-based Geophysical Measurements
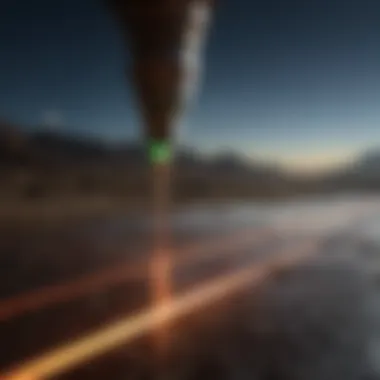
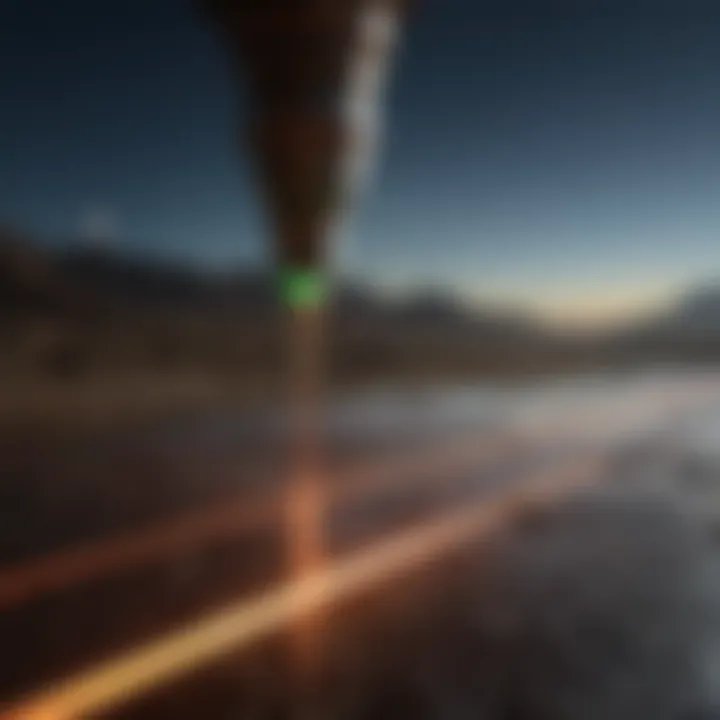
Laser-based geophysical measurements are pivotal in understanding geological formations and phenomena. Techniques such as laser-induced breakdown spectroscopy (LIBS) are utilized to analyze the composition of soil and rock samples. This method primarily involves focusing laser beams onto a material to generate a plasma, which emits light that is analyzed to determine elemental composition.
This approach is advantageous because it is rapid and provides real-time data. It facilitates detailed examination of mineral deposits, helping geologists and engineers make informed decisions about resource extraction. Additionally, laser range finding is commonly used to measure ground deformation and subsidence, providing essential data for earthquake studies and volcanic activity assessments.
Impact on Climate Studies and Monitoring
The integration of laser technology into climate studies significantly enhances our ability to monitor changes in the environment. By utilizing laser remote sensing, researchers can track greenhouse gas concentrations in the atmosphere, yielding insights into climate change dynamics. For instance, satellite-based laser systems like the Orbiting Carbon Observatory are capable of measuring carbon dioxide levels with high precision.
Moreover, lasers play a role in monitoring ice sheet dynamics and sea level rise, essential for understanding global warming impacts. The ability to make continuous and precise measurements at various locations contributes to more reliable climate models, informing policymakers and the public.
"The precision and efficiency of laser technology significantly improve our understanding and monitoring of complex environmental changes."
Future Directions in Laser Technology
The continuous advancement in laser technology significantly influences various scientific disciplines. This section explores the upcoming trends, emerging technologies, and collaborative efforts shaping the future of lasers. Understanding these future directions is crucial, as they hold the potential to enhance research capabilities, improve precision, and open new avenues for discovery. The ongoing integration of laser technology into different fields highlights its versatility and indispensable role in modern science.
Emerging Laser Technologies and Their Potential
Emerging laser technologies are transforming how researchers approach problems across different scientific fields. Innovations such as ultrafast lasers, which can produce extremely short pulses of light, are enabling scientists to study processes at unprecedented temporal resolution. For example, researchers can observe chemical reactions in real time, leading to a better understanding of molecular dynamics.
Another promising development is the progress in laser systems that operate at different wavelengths. These systems find applications in various areas such as targeted medical treatments using laser phototherapy, and precision agriculture utilizing laser-based imaging techniques for crop analysis.
The ability to manipulate light at different frequencies can lead to novel applications, enhancing the usability of laser technology in diverse scientific research.
- High-energy lasers for defense and industrial applications.
- Green lasers that minimize environmental impact.
- Fiber lasers for efficient and compact solutions in manufacturing.
Interdisciplinary Applications of Laser Research
As laser technology advances, its interdisciplinary applications continue to grow. Collaboration between biology, chemistry, physics, engineering, and environmental science is becoming more common. For example, laser-based methods in biology are not limited to medical diagnostics; they are also transforming ecological studies. Researchers are now able to map populations of marine organisms using laser scanning techniques, drastically improving data accuracy.
Furthermore, the interplay of lasers with artificial intelligence opens new possibilities. Combining machine learning with laser imaging techniques can lead to real-time data analysis from experiments. This facilitates not only faster results but also deeper insights into complex systems, making interdisciplinary research more efficient and impactful.
Some applications include:
- Laser-guided surgery combining precision tools with real-time imaging.
- Chemical analysis using laser-induced breakdown spectroscopy in field settings.
- Remote sensing for monitoring environmental changes and resource management.
Challenges and Opportunities Ahead
Despite the undeniable progress, challenges persist in the expansion of laser technology. Researchers face the task of developing cost-effective laser systems that can be widely adopted across diverse fields. The initial investment in sophisticated laser technologies may deter smaller institutions or individual researchers from utilizing these advancements.
Moreover, as the field expands, data management and analysis become increasingly complex. Handling large datasets generated from laser experiments requires innovative computational solutions, which remain underdeveloped in some areas.
However, these challenges also bring opportunities for growth. The demand for more innovative laser applications prompts further research and development. Innovative funding solutions and collaborative projects between academia and industry could foster advancements. Additionally, enhancing educational programs focusing on laser technology can equip future scientists with necessary skills.
"The potential of laser technology is profound, but realizing it requires collective effort across different scientific domains."
In summary, navigating the challenges ahead presents an opportunity for scientists to implement cutting-edge solutions that not only advance their respective fields but also broaden the horizons of laser technology as a whole.
Culmination
The exploration of laser technology within various scientific domains reveals its profound relevance and transformative power. Understanding the impact of lasers is essential for grasping the landscape of modern research and innovations. This article has journeyed through numerous applications, showcasing not only how lasers are fundamental tools in laboratory settings but also how they have expanded the horizons of what is possible in scientific inquiry.
Summary of Key Points
In this discussion, we have highlighted several key aspects of laser technology:
- Definition and Historical Context: We began by outlining what lasers are and traced their development from theoretical concepts to practical applications.
- Interdisciplinary Applications: The versatility of lasers was examined across multiple disciplines, emphasizing their role in biology, chemistry, physics, and earth sciences.
- Future Directions: Emerging technologies and the potential for interdisciplinary research have been positioned as crucial areas for further exploration.
Each point underscores the importance of lasers in enhancing precision and efficiency in scientific endeavors.
The Continuing Importance of Laser Technology
Looking ahead, the continual evolution of laser technology holds significant promise. As lasers become more advanced, their applications will likely expand further. In medical fields, for example, new laser techniques are in development for more effective and less invasive treatments. Environmental scientists are leveraging lasers for more accurate climate models.
Moreover, the intersection of laser research with artificial intelligence and materials science presents exciting opportunities. Collaborations across disciplines will fuel innovation and streamline research processes.
"The integration of laser technology in research not only enhances current methodologies but also sets the groundwork for future discoveries."