Exploring the Impact of Phosphorylation Antibodies
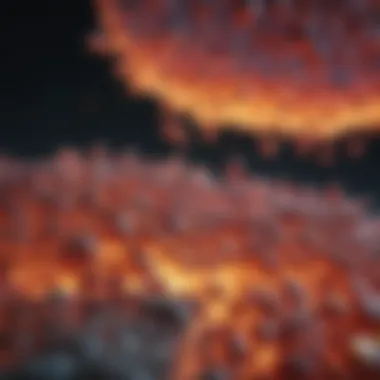
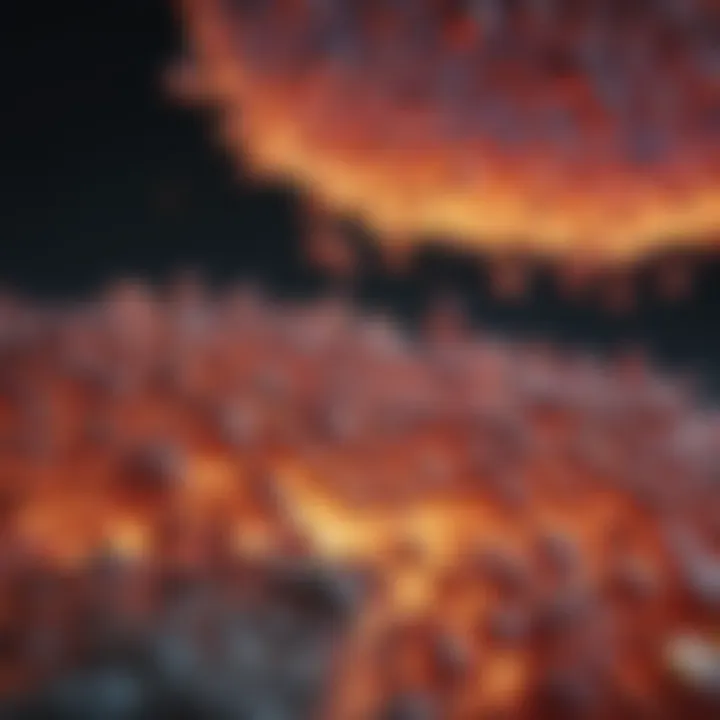
Intro
The increasingly intricate world of biological research continuously uncovers the vital role of phosphorylation antibodies. These antibodies are essential players in understanding how signaling pathways operate, thus contributing substantially to various fields such as cancer research and neuroscience. The beauty of phosphorylation lies in its dynamism, allowing proteins to be activated, deactivated, or modified in response to cellular signals—this process is nothing short of fascinating! By studying these antibodies, researchers can delve into the nuances of cellular communication and identify therapeutic targets.
As we embark on this exploration, it’s crucial to not only define what phosphorylation antibodies are but also to appreciate their significance within the greater context of biological research. This first section sets the stage, providing a comprehensive overview of research methodologies, implications, and both contemporary and future trends.
Preface to Phosphorylation Antibodies
The world of cellular biology brims with complexity, especially when one begins to unravel the intricacies of phosphorylation and the antibodies that interact with this crucial post-translational modification. Phosphorylation, the addition of a phosphate group to a protein, plays a pivotal role in regulating numerous cellular functions, from metabolism to signal transduction. This modification is not merely an accessory feature; it is often at the very heart of cellular communication, determining how cells respond to external stimuli. Thus, understanding phosphorylation antibodies is tantamount to grasping a fundamental aspect of how life operates at the molecular level.
Phosphorylation antibodies are tailored to recognize specific phosphorylated amino acids within proteins. Because phosphorylation can radically alter a protein's function, stability, or localization, the use of these antibodies in research and clinical settings has grown tremendously in importance. They are essential tools in deciphering the signaling pathways that govern a host of biological processes and diseases, especially cancer and neurodegenerative disorders.
The main benefits of employing phosphorylation antibodies in research are multifaceted:
- Specificity: These antibodies can differentiate between phosphorylated and non-phosphorylated states of proteins, allowing for precise analysis and characterization.
- Versatility: Useful across a variety of techniques, including Western blotting and immunohistochemistry, their applications span from basic research to clinical diagnostics.
- Insight Generation: By elucidating phosphorylation patterns, researchers glean insights into cellular mechanisms and disease states, making these tools invaluable in experimental biology.
However, employing phosphorylation antibodies is not without its challenges. Variations in antibody quality, potential cross-reactivity, and limitations in detection methodologies can hinder precise results. Understanding these challenges and effectively navigating them is crucial for researchers aiming to draw accurate conclusions from their experiments.
To establish a solid foundation, we must first break down the key components that shape this field. This necessitates a closer examination of what phosphorylation entails and the overarching concept of antibodies, both of which we will explore in detail within the next sections.
Defining Phosphorylation
Phosphorylation occurs when a phosphate group, a chemical entity consisting of phosphorus and oxygen, is transferred from one molecule, often ATP, and attached to a protein. This seemingly minor alteration can have outsized consequences for a cell, leading to changes in activity, interactions, or cellular location of proteins. The enzymes that execute this transfer are called kinases, while the enzymes that reverse this process are known as phosphatases. The dynamic interplay between these two types of enzymes forms a regulatory network vital for various cellular functions. Notably, the specificity of kinase-substrate interactions often dictates the precision with which cellular responses are modulated.
Overview of Antibodies
Antibodies, in a nutshell, are proteins produced by the immune system in response to foreign bodies or antigens. They serve as the body's defense mechanism, binding to specific epitopes on the antigen to neutralize or eliminate them. In the context of biochemistry, researchers have harnessed these natural proteins for targeted applications in research and diagnostics. The antibodies that target phosphorylated proteins are distinguished by their unique capacity to bind precisely to the phosphorylated variants while avoiding the non-phosphorylated counterparts. This capability not only assists in tracking phosphorylation events but also in elucidating the broader implications these modifications have for cellular activity.
Mechanisms of Phosphorylation
Understanding the mechanisms of phosphorylation is crucial. It's like peeking into a hidden world where minute actions can result in colossal shifts within cellular processes. This section will dissect the fundamental acts of kinases and phosphatases, two players that are pivotal in phosphorylation and dephosphorylation events. By grasping these mechanisms, one can appreciate how they contribute to various biological pathways and cell signaling. Grasping the dynamics can allow researchers to harness these processes to inform therapeutic strategies, especially in areas like cancer treatment and neurodegenerative diseases.
Role of Kinases and Phosphatases
Kinases and phosphatases can be viewed as the architects and demolishers of phosphorylation events. Kinases are enzymes that add phosphate groups to proteins in a process known as phosphorylation. This reaction can initiate signaling cascades, including cell division, differentiation, or even apoptosis. To put it simply, when a kinase is activated, it adds a phosphate group, altering the target protein's function. There are various kinases – serine/threonine kinases and tyrosine kinases are among the most acknowledged. Each has its particular chance to regulate specific substrates and pathways.
"Kinases are the gatekeepers of phosphorylation, determining which proteins get tagged and when."
On the other hand, phosphatases perform the reverse action, removing phosphate groups. Their role might be understated when thinking about cellular signaling, but they are equally important. If kinases are likened to builders, then phosphatases are the demolition experts, carefully taking down constructs when they are no longer needed. The balance between these two enzyme classes is what ultimately governs the state of phosphorylation in a cell.
In this delicate dance, the interplay between kinases and phosphatases maintains homeostasis within cellular processes. Disruptions in this balance can lead to diseases—take cancer, for instance—wherekinase activity may be excessively high, or phosphatase activity might be unregulated. The importance of identifying and targeting these enzymes in therapy cannot be overstated.
Specificity of Phosphorylation Events
The specificity of phosphorylation events is akin to a finely-tuned orchestra, where each musician plays their part in harmony. In phosphorylation, specificity refers to the unique interactions between kinases, substrates, and their phosphorylation sites. This selective nature is crucial because it determines how precisely a signal is relayed and can drastically influence various cellular pathways.
Several factors contribute to this specificity:
- Amino Acid Context: The sequence surrounding the phosphorylation site often dictates whether a kinase will recognize and add a phosphate group. For instance, certain kinases may only target proteins that have specific amino acids adjacent to the phosphorylation site.
- Molecular Interactions: Kinases are not merely wandering about. They often interact with scaffold proteins that help them reach their substrates more efficiently. These scaffold proteins can restrict the substrate available for phosphorylation and provide a localized environment for signaling to occur.
- Post-Translational Modifications: Other modifications, such as methylation or ubiquitylation, can also alter the ability of kinases to phosphorylate. They can change the protein conformation, making it either more or less accessible to kinases.
This specificity ensures that the signaling pathways are activated correctly, which is vital for the appropriate cellular response. When mishaps occur at this level, it can lead to diseases or contribute to dysfunction in cellular communication. Therefore, understanding these localized mechanisms of action is key to manipulating cellular responses for therapeutic interventions once the scientific community’s focus gets set on such pathways.
Types of Phosphorylation Antibodies
Understanding the various types of phosphorylation antibodies is critical in the realm of biological research. Each type serves distinct purposes, addressing different needs depending on the specific assays or experimental requirements. This section explores the nuances of three primary categories: polyvalent antibodies, monoclonal antibodies, and polyclonal antibodies. A thorough comprehension of these distinctions allows researchers to make informed decisions about which type of antibody best suits their investigative needs.
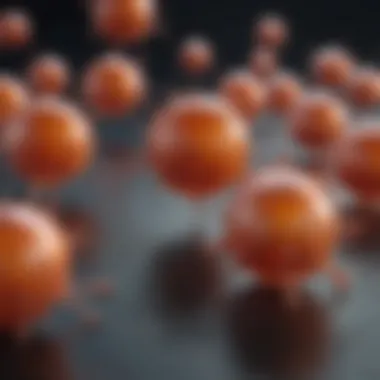
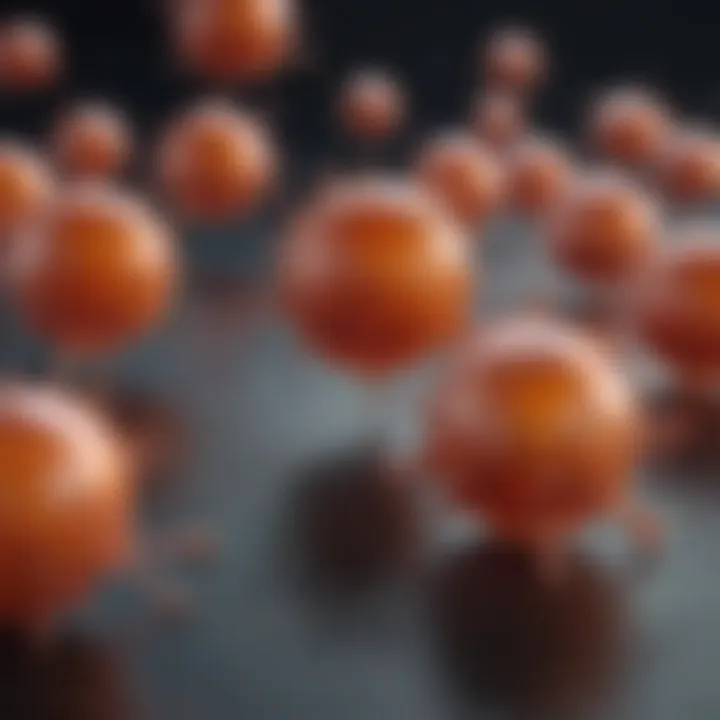
Polyvalent Antibodies
Polyvalent antibodies are those that can bind to multiple antigens or epitopes simultaneously. This characteristic makes them particularly useful in scenarios where diverse phosphorylation sites need to be probed. These antibodies can recognize a variety of protein conformations and modifications, making them versatile in applications such as protein interaction studies and signaling pathway analysis.
The benefits of using polyvalent antibodies include:
- Broad specificty: They increase the chances of detecting phosphorylated proteins in complex biological samples.
- Multiplexing capabilities: Researchers can investigate multiple targets in a single assay, saving time and resources.
However, there are challenges. The increased cross-reactivity can sometimes lead to false positives, complicating data interpretation. Thus, careful validation is critical when deploying these antibodies in experiments.
Monoclonal Antibodies
Monoclonal antibodies are produced from a single clone of immune cells, resulting in a homogeneous population that is engineered to detect a specific phosphorylation site. Their specificity is a double-edged sword; while it allows for high sensitivity in detecting particular phosphorylated residues, it also means that researchers must be judicious in their choice of antibody.
These antibodies present several advantages:
- High consistency: Each batch yields similar results, which can be crucial for reproducibility in scientific studies.
- Defined targets: Their finetuned specificity means they are especially valuable in applications demanding precise measurement, such as ELISA or flow cytometry.
Nonetheless, the downside is notable as well. These antibodies often require extensive validation; if researchers utilize a monoclonal antibody that does not effectively recognize the phosphorylated form of their target, it could derail experiments. Moreover, the production process can be relatively costly and time-consuming compared to other options.
Polyclonal Antibodies
Polyclonal antibodies arise from multiple B cell lineages, yielding a heterogeneous mix that can recognize many epitopes on the same protein target. This diversity means they are particularly robust against variations in peptide sequence or protein conformations, making them a popular choice in research settings.
The benefits of polyclonal antibodies can include:
- Increased sensitivity: The ability to detect varying levels of phosphorylation due to their broader specificity is advantageous in many assays.
- Resilience to epitope changes: This can be a major plus in dynamic biological environments where proteins can undergo substantial structural changes.
However, one must tread carefully. The variability in antibody quality from different batches may lead to inconsistencies. Researchers should conduct comparative experiments to determine the suitability and effectiveness of the polyclonal antibody in their specific context.
In summary, choosing the right type of phosphorylation antibody can significantly affect experimental outcomes. Employing the appropriate antibody type requires a clear understanding of each option’s strengths and weaknesses, ultimately steering the research in the right direction. As the field continues evolving, comprehending these differences enables scientists to design more effective experiments and yield reproducible and reliable results.
Applications in Biological Research
When we consider the profound impact of phosphorylation antibodies, particularly in biological research, their role leans heavily on enhancing our grasp of intricate cellular processes. Phosphorylation is like a light switch; flipping it can turn various cellular functions on or off, influencing everything from cellular communication to metabolic activity. As researchers delve deeper into these mechanisms, they are discovering that inhibition or activation of phosphorylation can trigger significant variations in biological outcomes.
Role in Signal Transduction Pathways
Signal transduction pathways, the complex networks that cells use to respond to internal and external cues, are heavily reliant on phosphorylation. When a signal is received, kinases typically catalyze the addition of a phosphate group to specific proteins, which changes their shape and activity. This is crucial because it effectively acts like a relay race—each phosphorylated molecule passing the baton to the next one in line.
"Kinases and phosphatases function like traffic lights in cellular signaling, directing the flow of information by toggling protein activity on and off."
Examining these pathways often involves using phosphorylation antibodies to confirm whether specific proteins have been phosphorylated in response to a stimulus. For instance, researchers looking at insulin signaling pathways will check which proteins are phosphorylated after insulin binds to its receptor. This kind of keen observation allows scientists to map out these pathways more accurately, helping them unravel diseases characterized by disrupted signaling, such as diabetes or cancers.
Phosphorylation in Cell Cycle Regulation
Phosphorylation is also a heavyweight player in the regulation of the cell cycle—a tightly orchestrated series of events that lead to cell division. Cyclins and cyclin-dependent kinases (CDKs) are at the forefront of this drama, working together to ensure that each phase of the cell cycle is executed flawlessly. Imagine CDKs as construction foremen and cyclins as laborers who show up only when the job demands it. When the time comes, these foremen activate the laborers through phosphorylation, initiating processes like DNA replication.
Maintaining proper phosphorylation states during the cell cycle is vital. Misregulation can lead to uncontrolled cell growth, a hallmark of many cancers. Addressing this through the application of phosphorylation antibodies provides insights into cell cycle checkpoints, helping in the identification of new therapeutic targets that could potentially normalize the cycles of aberrant cells.
Use in Cancer Biomarker Discovery
The quest for cancer biomarkers takes researchers through a maze of complexity, and phosphorylation antibodies are invaluable in this pursuit. Certain oncogenes and tumor suppressor genes are directly implicated in altering phosphorylation states. As such, antibodies that specifically target phosphorylated forms of proteins help researchers pinpoint variations between normal and malignant cells.
For example, the p53 protein, known for its role as a tumor suppressor, can become phosphorylated in response to cellular stress. By utilizing antibodies against its phosphorylated state, scientists can assess cellular responses to therapeutic treatments, determine prognosis, and even evaluate the effectiveness of targeted therapies.
In this way, phosphorylation antibodies are not just lab tools; they are crucial lifelines guiding the research community towards effective cancer therapies and enabling more personalized treatment pathways.
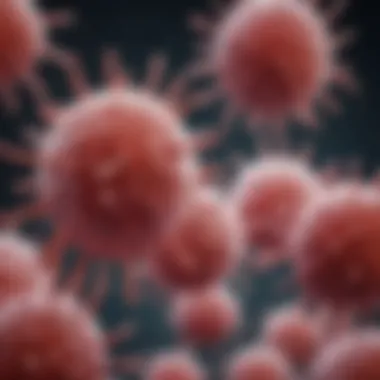
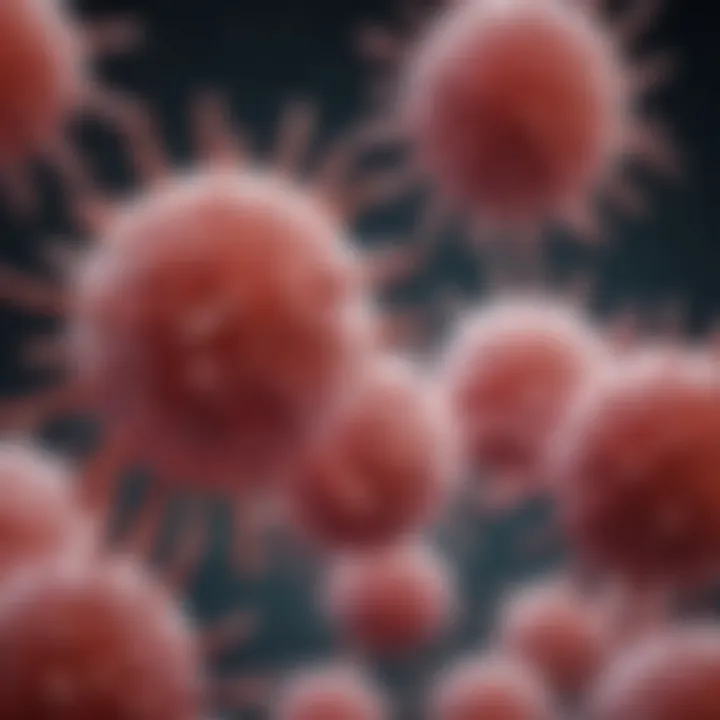
As we move through this exciting area of biological research, it becomes clear that phosphorylation antibodies serve diverse and essential functions that tie into the very fabric of cell biology, disease understanding, and therapeutic development.
Detection Techniques
Detection techniques for phosphorylation antibodies play a crucial role in both experimental design and the interpretation of biological data. These methods enable researchers to identify and quantify phosphorylated proteins, offering insights into various pathways, cellular functions, and potential disease states. Understanding these techniques can aid in choosing the most suitable approach based on research goals and the nature of biological samples.
Western Blotting
Western blotting is a widely utilized technique in molecular biology for detecting specific proteins in a sample. The procedure starts with electrophoresis, where proteins are separated by molecular weight. The proteins then get transferred to a membrane, and the detection begins. Here’s how it generally works:
- Sample Preparation: Proteins from cells or tissues are extracted using lysis buffers that often contain phosphatase inhibitors to prevent dephosphorylation.
- Gel Electrophoresis: Samples are loaded onto a gel matrix, which separates proteins based on size during an electric current.
- Transfer to Membrane: Once separated, proteins are transferred onto a blotting membrane, usually made of nitrocellulose or PVDF.
- Antibody Incubation: The membrane is incubated with blocking agents to prevent non-specific binding. Primary antibodies specific to the phosphorylated form of the target protein are then applied, followed by secondary antibodies linked to a detection system.
- Visualization: Detection is performed through chemiluminescence or colorimetric methods, allowing researchers to visualize unique bands corresponding to phosphorylated proteins.
This technique is celebrated for its ability to provide qualitative and semi-quantitative data on protein expression levels, making it invaluable in various studies, including cancer research and signaling pathways.
Immunofluorescence
Immunofluorescence involves the use of fluorescently labeled antibodies to detect specific proteins within fixed cells or tissue sections. This approach offers the visual localization of target proteins, which is significant in understanding their functional roles in cellular context. The steps typically include:
- Sample Fixation: Cells or tissue samples are first fixed to preserve structure and stop cellular events.
- Permeabilization: The cells are treated with detergents to allow antibodies to penetrate cell membranes.
- Blocking: Non-specific sites are blocked using serum from the same species that the secondary antibody was raised in.
- Primary Antibody Application: The sample is incubated with a primary antibody specific for the phosphorylated target protein.
- Secondary Antibody Addition: A secondary antibody conjugated to a fluorescent dye is applied, which binds to the primary antibody.
- Microscopy: Visualization occurs using a fluorescence microscope, allowing researchers to observe not only the presence but also the subcellular localization of phosphorylated proteins.
Immunofluorescence provides real-time insights into the spatial dynamics of phosphorylation events within living cells.
Enzyme-Linked Immunosorbent Assay (ELISA)
Enzyme-Linked Immunosorbent Assay, or ELISA, is essential for quantifying proteins in various samples, such as blood, serum, or cell lysates. This technique employs enzyme-linked antibodies that produce a measurable signal once substrate is added. The process usually unfolds as follows:
- Coating the Plates: A specific amount of the capture antibody is coated onto the wells of a microtiter plate.
- Sample Addition: Samples containing the target protein are added, allowing binding to the coated antibodies.
- Blocking: Non-bound sites are blocked to prevent background noise, enhancing specificity.
- Detection Antibody: A secondary enzyme-linked antibody is added, targeting the phosphorylated protein of interest.
- Substrate Reaction: After thorough washing, a substrate is introduced. The enzymatic reaction produces a color change, which can be quantitatively measured by a spectrophotometer.
ELISA is notably praised for its sensitivity and capacity for high-throughput analysis, making it an excellent choice for screening and quantification of phosphorylated proteins involved in various diseases.
In summary, selecting the appropriate detection technique affects data integrity and interpretative accuracy in biological research. Each method has its strengths, and understanding these differences is key to advancing knowledge in the field of phosphorylation antibodies.
Challenges in the Use of Phosphorylation Antibodies
Phosphorylation antibodies are vital tools in the realm of biological research. Yet, their application is not without significant challenges. Understanding these hurdles is crucial for researchers aiming to yield accurate and replicable results. The issues range from cross-reactivity complications to quality inconsistencies, all of which demand careful attention.
Cross-Reactivity Issues
Cross-reactivity is one of the primary concerns when working with phosphorylation antibodies. This problem arises when an antibody binds not only to its intended target but also to other proteins or modified residues, leading to erroneous conclusions. For instance, if an antibody designed for phosphorylated Akt binds to a similar structure in another protein, the resulting data can mislead researchers regarding the signaling pathways.
To address this, researchers must critically evaluate antibody specificity before use, often by referencing data sheets or published studies. However, it’s essential to recognize that even well-characterized antibodies may still exhibit unexpected interactions under certain experimental conditions. Hence, implementing rigorous controls and standards is necessary to ensure that findings accurately reflect biological realities.
Variability in Antibody Quality
Antibody quality can vary widely across different manufacturers and even batches. This inconsistency poses a challenge when reproducibility is paramount in research. For instance, an antibody with a robust performance in one experiment may produce disparate results in subsequent studies. Variability is often due to factors such as differences in production methods, purification processes, or even storage conditions.
When researchers encounter unreliable antibodies, they can face setbacks in research timelines and unexpected increases in costs. Thus, a best practice includes opting for antibodies that have been thoroughly validated across multiple studies and conditions, ensuring a higher confidence level in their results. Even so, researchers must remain vigilant, keeping a close watch on how each batch performs in their specific context.
Limitations in Detection Techniques
Detection of phosphorylated proteins is another crucial factor affecting the efficacy of phosphorylation antibodies. Various methods exist—such as Western blotting and immunohistochemistry—but each technique comes with its own limitations. Sensitivity and specificity can vary greatly; for example, while Western blotting is a go-to method, it may not be sensitive enough to detect low-abundance phosphorylated proteins without extensive sample preparation.
Moreover, some assays might not preserve the phosphorylation state during sample preparation, leading to false negatives. As such, it’s vital for researchers to choose their detection methods judiciously and remain aware of their potential pitfalls. Iterative testing and validation alongside thorough documentation can mitigate these issues, allowing for more reliable outcomes in phosphorylation research.
Understanding these challenges is not merely academic; they are essential to achieving reliable data that can drive forward our knowledge in molecular biology.
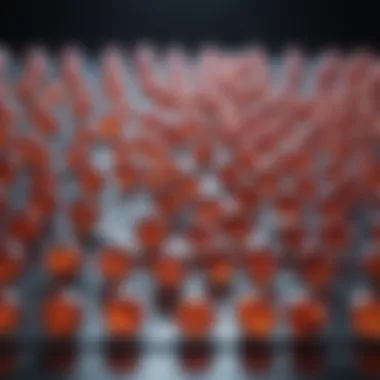
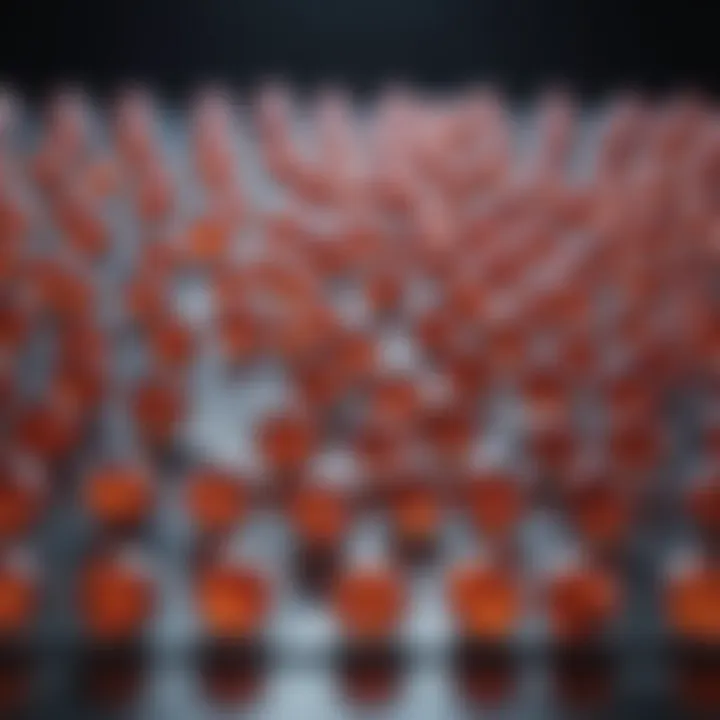
In summary, while phosphorylation antibodies empower scientific discovery, the challenges they pose cannot be overlooked. Addressing cross-reactivity issues, ensuring antibody quality, and navigating detection limitations are pivotal steps in optimizing their use in research.
Case Studies in Research
In the domain of phosphorylation antibodies, case studies serve as vital signposts, showcasing real-world applications and the consequent ramifications on various scientific fields. Such case studies do not merely illustrate the theoretical significance of phosphorylation; they offer a tangible lens through which we can view the complexities of biological systems. By exploring these specific instances, we glean insights into how these antibodies facilitate research, drive innovation, and reveal underlying mechanisms in diseases and biological processes.
Advancements in Cancer Research
The use of phosphorylation antibodies has brought remarkable advancements in oncology research. Cancer cells often exhibit abnormal phosphorylation patterns which can serve as biomarkers. For instance, the study of the HER2 protein, a well-known oncoprotein, illustrates how phosphorylation antibodies can help in understanding its role in tumor proliferation.
Research has revealed that phosphorylated HER2 correlates with aggressive tumor behavior. Using phosphorylation antibodies directed against specific HER2 phospho-epitopes allows researchers to detect and quantify these modifications with precision. This not only aids in diagnosis but also helps in monitoring treatment responses. With the advent of such targeted therapies, the potential to personalize cancer treatment has become increasingly feasible.
"Advancements in understanding cancer biology through phosphorylation antibodies are redefining personalized medicine."
Moreover, in the context of targeted therapy development, a study involving the targeting of AKT, another phosphorylation pathway, has shown notable improvements in therapeutic strategies for certain breast cancer subtypes. Researchers utilized monoclonal antibodies that specifically recognized phosphorylated AKT, allowing them to demonstrate the pathway’s role in tumorigenesis and survival in cancer cells.
This journey of discovery does not end with therapeutic implications; it extends into prognostic evaluations as well. Phosphorylation antibodies have opened doors to new horizons in prognostic stratification where identifying patients at risk of poor outcomes becomes achievable through specific phosphorylation markers.
Investigating Neurodegenerative Diseases
Phosphorylation also plays a pivotal role in the realm of neurodegenerative diseases, with case studies shedding light on the mechanisms responsible for such disorders. Alzheimer’s disease is perhaps the most studied in this context, with tau protein hyperphosphorylation being a hallmark feature.
In recent research, the development of phosphorylation antibodies targeting hyperphosphorylated tau provides a robust tool for understanding the intricate pathways that lead to neurodegeneration. By examining the presence and concentration of these phosphorylated forms in cerebrospinal fluid or in neuroimaging, researchers have developed diagnostic tests that can better predict the onset of Alzheimer’s before clinical symptoms manifest.
Studies have illustrated how these investigative tools do not only clarify the pathological landscape but also reveal potential therapeutic targets. For example, a recent study observed that inhibiting specific kinases responsible for tau phosphorylation could potentially reverse some effects of Alzheimer’s pathology. This kind of research underscores the importance of targeted approaches in therapeutics, all thanks to the insights provided through phosphorylation antibodies.
In summary, the case studies in cancer and neurodegenerative diseases exemplify the vast potential of phosphorylation antibodies. The profound implications of these findings not only push the boundaries of research but also hold promise for future therapeutic applications. As researchers continue to unravel these complex biological processes, phosphorus and its regulatory mechanisms remain at the forefront of scientific inquiry.
Future Directions in Phosphorylation Antibody Research
The exploration of phosphorylation antibodies holds significant promise for the advancement of biological sciences. This section will delve into prospective developments shaping this field, particularly in how they can enhance research methodologies and clinical applications. As scientists continuously seek to undo the knots of biological complexity, understanding phosphorylation and its associated antibodies becomes ever crucial. Innovations in this area could lead to breakthroughs not only in basic research but also in therapeutic contexts, thereby underscoring the relevance of this topic.
Emerging Technologies
Adopting fresh technologies can profoundly impact how we harness phosphorylation antibodies. Here are some of the notable innovations:
- Nanotechnology in Antibody Development: The Miniaturization of antibody microarrays with nanostructures could enable the simultaneous detection of multiple phosphorylation events. This method would allow researchers to paint a clearer picture of cellular signaling pathways.
- CRISPR-Cas9 Applications: The utilization of CRISPR systems can help modify antibodies for increased specificity towards phosphorylated epitopes. By targeting specific gene functions, researchers can ease the identification of phosphorylation events in real-time.
- Machine Learning Algorithms: The integration of AI tools in analyzing data stemming from phosphorylation studies can optimize the interpretation of results. They can uncover patterns and predict outcomes that were previously undetectable by traditional methods.
- Microfluidics: Advanced microfluidics technology can simplify the processes of antibody binding and detection. As such, it offers faster, cheaper, and more efficient ways to analyze complex samples—including those from difficult tissues or small quantities of cellular materials.
Incorporating these advancements into the toolkit of researchers can lead to unprecedented insights into the biochemical pathways that govern life.
Potential Clinical Applications
The horizon for clinical applications of phosphorylation antibodies continues to expand, offering exciting opportunities for tackling various health conditions. Here are potential areas where they might play a crucial role:
- Precision Medicine in Oncology: Customizing treatments based on the phosphorylation status of proteins in tumors could shift the entire landscape of cancer therapy. This would allow for tailored therapies that increase effectiveness while minimizing adverse effects.
- Neurodegenerative Disease Interventions: Abnormal phosphorylation patterns often correlate with diseases such as Alzheimer’s. Antibodies targeting these aberrant states could serve not only as diagnostic tools but also as therapeutic agents, potentially slowing disease progression.
- Biomarker Discovery in Autoimmune Disorders: Identifying phosphorylation changes can help develop biomarkers critical for early detection and monitoring the progression of autoimmune diseases, thereby improving patient outcomes.
- Regenerative Medicine Applications: Understanding the phosphorylation landscape could enhance tissue regeneration strategies, for example in stem cell therapy, by ensuring the right signals are activated during cellular differentiation.
These developments hold the potential to reshape not just how we diagnose and treat diseases, but also how we understand the very mechanisms at play within living organisms.
The End
In this article, we have explored the intricate landscape of phosphorylation antibodies, underscoring their critical role in both basic and applied scientific research. The importance of this topic extends beyond mere comprehension of biochemical processes; it encompasses the advancement of medical diagnostics and therapeutic strategies.
Summary of Key Points:
- Phosphorylation plays a pivotal role in regulatory pathways that control cellular functions.
- Various types of phosphorylation antibodies — including polyvalent, monoclonal, and polyclonal — have distinct applications and implications.
- Detection techniques such as Western blotting and immunofluorescence allow researchers to visually and quantitatively assess phosphorylation, yielding invaluable insights.
- Despite challenges like cross-reactivity and variability in antibody quality, innovative approaches in antibody research are paving the way for enhanced specificity and utility in scientific inquiries.
Impact on Scientific Research:
The impact of phosphorylation antibodies on scientific research is both profound and multifaceted. These antibodies are not only tools for detection but also serve as bridges to understanding complex cellular signaling networks. For instance, in cancer research, elucidating phosphorylation patterns can reveal pathways that are aberrant in tumors, guiding treatment strategies.
Moreover, in the realm of neuroscience, phosphorylation antibodies are crucial for studying neurodegenerative diseases, as they allow researchers to visualize changes in protein activity related to cellular health. The continuous evolution of technologies will likely enhance the specificity and efficiency of these antibodies, making them even more integral in therapeutic development.
"Phosphorylation antibodies open doors to previously uncharted areas of biological research, continuously influencing our approach to understanding life at the molecular level."
As we reflect on the future of this field, the prospects are bright. The ongoing development and optimization of these tools promise to dramatically reshape our understanding of cellular mechanisms and foster breakthroughs in areas like personalized medicine and regenerative therapies.