Exploring Methods for Measuring Thermal Conductivity
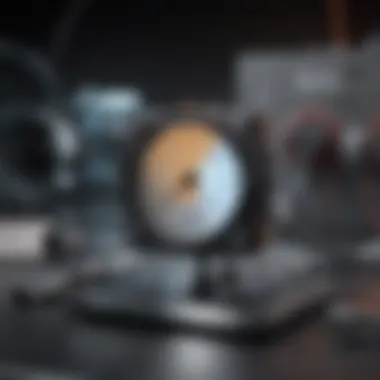
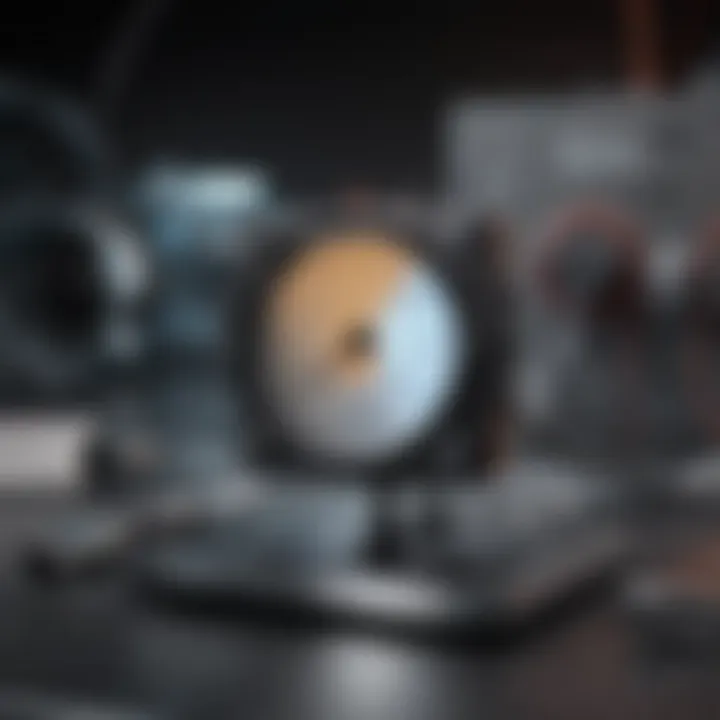
Intro
Understanding thermal conductivity measurement techniques is essential for exploring a wide array of scientific and engineering applications. From everyday materials found in homes to advanced composites in aerospace engineering, the thermal properties of substances significantly influence their function and performance. This article will unfold various methods of measuring thermal conductivity, highlighting their advantages and limitations, which will provide a holistic view to students, professionals, and educators alike.
Research Overview
Methodological Approaches
Measurement techniques for thermal conductivity can generally be divided into two distinct categories: direct and indirect methods. Each of these approaches comes with its own set of principles, challenges, and areas of application.
- Direct Methods: Often seen as the more straightforward techniques, direct methods measure thermal conductivity by applying a known heat flow to the material and observing the temperature change over time. Common examples include the steady-state method and transient techniques like the laser flash analysis. These approaches typically yield precise and immediate results, crucial for detailed material characterization.
- Indirect Methods: On the other hand, indirect approaches, such as differential scanning calorimetry and returned heat flux methods, estimate thermal conductivity based on thermal properties like heat capacity or thermal diffusivity. While these methods might not provide the same level of precision as direct techniques, they are often easier to implement and can be applied to a broader range of sample types.
Understanding these methods in-depth also involves recognizing the standard testing conditions under which these measurements occur, such as sample size, homogeneity, and environmental effects such as temperature and humidity.
Significance and Implications
The ability to accurately measure thermal conductivity is paramount across numerous fields:
- Material Science: In the development of new materials, thermal conductivity plays a pivotal role in determining thermal stability and energy efficiency.
- Construction Engineering: Building materials' thermal properties directly impact energy consumption in heating and cooling, making these measurements essential for sustainable design.
- Electronics: As devices become smaller and more powerful, managing heat dissipation has become crucial. Accurate measurement methods facilitate the design of more efficient electronic components.
In summary, the methodologies adopted for thermal conductivity measurement influence not just the scientific understanding of materials, but also the practical applications in various industries.
Current Trends in Science
Innovative Techniques and Tools
The world of thermal conductivity measurement is not stagnant; new tools and innovative techniques are being developed continually. For instance, advancements in laser flash analysis have enhanced the precision of transient thermal conductivity measurements significantly. Equipment has also become more accessible, facilitating broader adoption of these measurement techniques outside specialized labs.
- High-Throughput Techniques: Automated systems enable researchers to conduct simultaneous tests on multiple samples, speeding up the characterization process without compromising on accuracy.
- Digital Measurement Integration: Software solutions that integrate with measurement tools allow for real-time data analysis, improving experimentation workflow and reliability.
Interdisciplinary Connections
Thermal conductivity is deeply intertwined with various scientific branches. Techniques developed in one field often find their way into others, enhancing research outputs across disciplines.
For instance, insights from nanotechnology have propelled advancements in measuring thermal properties of nanomaterials, paving the way for applications in energy conversion and storage. Likewise, understanding thermal properties in biological systems leads to advances in medical devices and treatments.
Foreword to Thermal Conductivity
Thermal conductivity is a significant property of materials that influences various scientific and industrial processes. Understanding this subject is not merely an academic exercise; it has a profound impact on fields as diverse as engineering, construction, and electronics. When we talk about thermal conductivity, we're addressing how well a material can conduct heat. This attribute plays a pivotal role in energy efficiency, safety, and sustainability across countless applications.
This section will guide readers through the essentials of thermal conductivity, laying a foundation for appreciation of its role in multiple contexts. It will help individuals grasp why accurate measurement of thermal conductivity is vitalânot just for material selection but also for the fine-tuning of many technical systems.
Definition and Significance
Defining thermal conductivity involves describing it as the quantity of heat that passes per unit of time through a unit area of a material when a temperature difference exists. It is a material's response to thermal gradient, and itâs measured in watts per meter kelvin (W/(m·K)). Understanding this definition is key. Thermal conductivity signifies how a material behaves under heat flux, which can greatly influence design decisions in construction, manufacturing, and even the design of everyday products.
The significance of thermal conductivity measurement cannot be overstated. For example, in the construction industry, accurate measurements help in choosing the right insulating materials which can lead to energy savings in buildings. Consider a straightforward scenario: if the walls of a house are made from a material with poor thermal conductivity, heat will escape quickly, increasing heating costs. On the flip side, materials with high thermal conductivity are used in heat sinks in electronics, ensuring that devices do not overheat and malfunction. Hence, knowledge of thermal conductivity directly correlates to operational efficiency and economic benefits.
Applications in Science and Industry
Thermal conductivity finds its way into numerous applications, stretching from everyday household items to advanced technological devices. Some significant applications include:
- Construction and Building Materials: Proper choices in insulation materials rely heavily on their thermal conductivity. This affects energy consumption, comfort levels, and utility bills.
- Electronics: Heat management is critical in electronic devices. Components such as heat sinks and thermal pads take advantage of materials with high thermal conductivity to dissipate heat efficiently.
- Automotive Industry: In vehicles, thermal conductivity directly relates to engine efficiency and passenger comfort. Materials used in engines must efficiently transfer heat to prevent overheating.
- Food Industry: Understanding how heat moves through various materials helps in cooking methodsâlike how quickly and evenly heat penetrates a pie or steak.
- Research and Development: In laboratories, scientists often investigate new materialsâ thermal properties as part of material science. Discovering materials with tailored thermal conductive properties can lead to innovations.
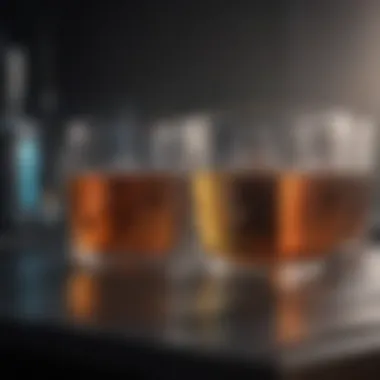
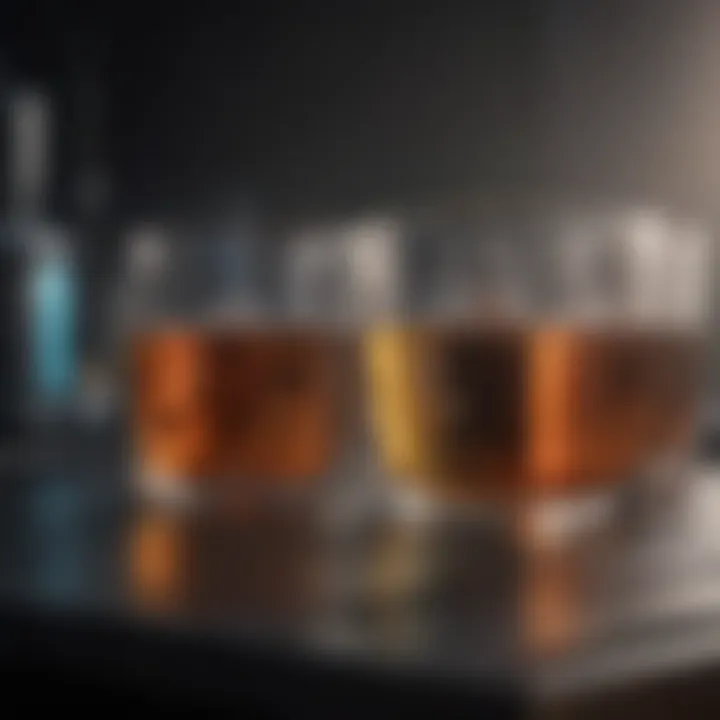
In summary, grasping the fundamentals of thermal conductivity is not just an academic pursuit for students or researchers; it is a crucial understanding for professionals across a spectrum of industries. As the demand for energy-efficient and high-performing materials grows, the importance of accurate thermal conductivity measurements will become even more pronounced.
Theoretical Foundations of Thermal Conductivity
Understanding the theoretical foundations of thermal conductivity is pivotal in grasping how heat transfers through materials. This foundational knowledge not only enriches our comprehension of thermal behavior but also shines a light on the practical implications of measuring thermal conductivity. The heat transfer mechanisms and material properties influencing conductivity are intricate subjects that define how different materials respond under thermal stress. Thus, for students, researchers, educators, and professionals alike, developing a robust grasp of these concepts is unsurpassed in its importance.
Heat Transfer Mechanisms
Heat transfer in materials encompasses three primary mechanisms: conduction, convection, and radiation. However, when it comes to thermal conductivity, conduction stands tall as the main player.
- Conduction occurs when heat transfers through direct contact between materials. Think of it as a relay race; the energy passes from one particle to the next until it reaches the other end.
- Convection involves the movement of fluids â either liquid or gas. Warm fluids rise while cooler ones sink, creating a cycle. It's like a dance between molecules where energy is efficiently circulated.
- Radiation is the transfer of heat in the form of electromagnetic waves. No contact is necessary; hence, it can occur in a vacuum. This is why the sun warms the Earth's surface without needing to touch it.
In the context of thermal conductivity, the focus remains squarely on conduction. The efficiency of heat transfer through a material during conduction is defined by its thermal conductivity, denoted by the symbol k. This parameter plays a crucial part in applications ranging from insulating materials to thermal management in electronics. To provide further clarity, high thermal conductivity materials, such as copper, swiftly conduct heat, while insulating materials like foam provide significant resistance to heat flow.
"The rate of heat conduction through a material is directly proportional to the temperature gradient across that material and its cross-sectional area, while being inversely proportional to its thickness."
Some factors that influence heat conduction include temperature, material composition, and structural integrity. As materials heat up, they tend to vibrate more vigorously, which can enhance the transfer of thermal energy through them.
Material Properties Affecting Conductivity
Material properties play a critical role in determining thermal conductivity. Several intrinsic characteristics can heavily influence how well a material conducts heat:
- Density: Generally, denser materials have more atoms packed closely together, which can enhance conductivity. Metals, being dense, often showcase high thermal conductivity.
- Specific Heat Capacity: Materials that require more energy to change their temperature will conduct heat more slowly. These attributes can create significant variations in thermal performance.
- Phase-State: The state of a materialâgas, liquid, or solidâimpacts its thermal conductivity. Solids tend to be better conductors than gases. For instance, although water is denser than air, steam still conducts heat much slower than solid metals due to its gaseous state.
- Moisture Content: In materials such as wood, higher moisture content can lead to improved thermal conductivity at the expense of structural integrity.
Identifying how these properties interplay enhances predictive models involving thermal conductivity. Engineers and researchers must consider these variable characteristics when selecting materials for specific applications, be it in construction, manufacturing, or electronics.
By integrating these theoretical underpinnings into practical situations, learners and professionals can significantly improve their understanding of thermal dynamics and the methodologies used to measure or evaluate thermal conductivity appropriately. In turn, this can lead to innovation in product development and scientific research in various fields.
Direct Measurement Techniques
Direct measurement techniques are pivotal when it comes to evaluating thermal conductivity because they provide actual readings rather than relying on theoretical estimates. The significance of these methods lies in their ability to yield precise measurements, which are crucial for applications across a spectrum of fields including materials science and engineering. By adopting such techniques, researchers and industry professionals can ascertain how different materials respond to heat, leading to better product development and optimization.
Guarded Hot Plate Method
Operational Principle
At the heart of the guarded hot plate method is its straightforward operational principle. The method employs a heated plate that is placed between two samples. It basically measures the heat flow through a material sample while controlling factors like temperature gradient. A defining characteristic is its ability to produce highly accurate results, even with diverse materials. This is what makes it a preferred choice in laboratory settings. However, its effectiveness can be limited when samples are heterogeneous or have irregular shapes, which could skew results.
Advantages
When talking about advantages, one must consider the high level of accuracy and repeatability that the guarded hot plate method offers. This meticulous technique allows for precise results, making it a solid option for both academic research and industrial applications. Its unique feature lies in the meticulous control of the test environment which minimizes external influences, thus providing reliable readings. However, users need to be aware that while the method excels in certain parameters, it may not be as suitable for rapid testing scenarios where time is of the essence.
Limitations
Despite its many strengths, this method is not without its limitations. One major drawback is the time-consuming nature of the procedure, often requiring a significant amount of time to reach thermal equilibrium. This characteristic makes it less practical for some quick applications. Additionally, the need for large sample sizes can limit its usability, especially in cases where material availability is restricted. While it shines in accuracy, cost and time factors must always be taken into account.
Heat Flow Meter Method
Operational Insights
The heat flow meter method operates on a principle that is relatively easy to grasp, focusing on the measurement of heat flow rate through a sample material. At its core, it assesses the energy that passes through a material when there is a known temperature difference across it. A key characteristic is its simplicity, making it user-friendly. As a common method in initial evaluations, it provides a quick approximation of thermal conductivity. Nonetheless, the results can sometimes vary if the material has uneven characteristics, which is a consideration for users seeking accuracy.
Pros and Cons
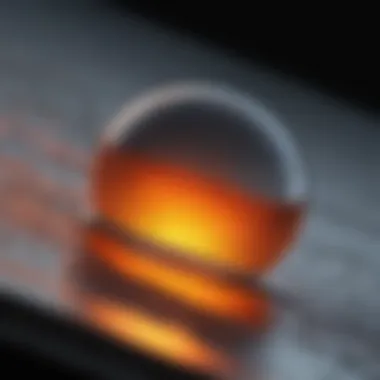
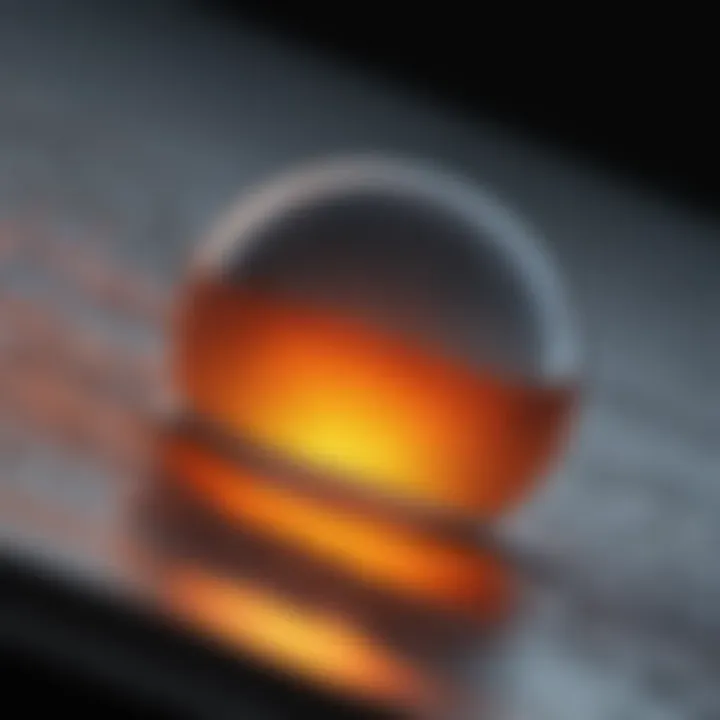
When weighing pros and cons, this method has the advantage of being relatively fast and can be carried out in a fraction of the time compared to other techniques. It is particularly useful for large-scale testing of insulation materials, allowing for streamlined assessments. The unique strength lies in its capacity to evaluate samples in their natural states.
Yet, the limitations canât be ignored. The method may introduce errors if the sample shapes deviate from the assumptions made for the analysis. Moreover, it might not account for thermal lag factors, leading to misinterpretations in certain circumstances. As such, a well-rounded view of its effectiveness will always require knowledge of its conditions and limitations.
Indirect Measurement Techniques
Indirect measurement techniques play a vital role in determining thermal conductivity, especially when dealing with materials that present challenges in direct measurement. These methods provide an effective alternative by using thermal response and transient conditions to gather data on thermal properties. The advantages of these techniques include their adaptability to a wider variety of materials and the possibility of minimizing sample size, leading to enhanced applicability in both research and industrial contexts.
Transient Plane Source Method
Basic Principles
The transient plane source method is based on the principle of heat diffusion from a heat source through a material. A sensor, often a thin disc, is placed against the material's surface. As it heats up, the heat travels through the material, and the temperature rise at the sensor is monitored over time. The key characteristic here is the ability to measure the thermal response quickly, which makes the technique appealing for various research and industrial applications. One unique feature is its applicability to both solid and fluid materials, making it a flexible option. However, one must consider that precise control of the environment is a necessity, which may limit field applications and increase complexities.
Applications
The transient plane source method is particularly beneficial in determining the thermal conductivity of polymers, ceramics, and composite materials. Its unique ability to provide quick results enhances its popularity in situations where timing is critical, such as in production lines or during rapid prototyping. While this method shines in terms of speed and usability, it might not always capture heterogeneity in material properties which could lead to a less comprehensive picture of the thermal conductivity across different sections of a material.
Challenges
Despite its advantages, the transient plane source method has its challenges. One significant issue lies in the sample thickness; for example, if the material is too thin, the heat may escape from the sides, resulting in inaccurate data. Furthermore, the thermal contact resistance between the sensor and the material surface has to be accounted for, as it can introduce measurement errors. Such challenges necessitate a thorough understanding of the method to utilize it effectively, reiterating the need for careful preparation and consideration in experimental design.
Laser Flash Analysis
Methodology
Laser flash analysis is an advanced technique ideal for measuring thermal diffusivity, which can then be used to derive thermal conductivity. In this method, a short laser pulse heats one side of a sample, while the other side's temperature change is recorded. This technique stands out due to its high precision and rapid assessment capability. The methodologyâs unique feature is its ability to measure small samples quickly, making it particularly advantageous in research settings where material volumes are limited. The downside, however, is the complexity of equipment and data interpretation, which can require specialized knowledge.
Benefits
The benefits of laser flash analysis include its rapid measurement capabilities and ability to analyze various materials, from metals to ceramics. This method also provides a non-destructive means of testing, which is increasingly important in quality control and material evaluation contexts. However, the requirement for well-controlled laboratory conditions can be a drawback, limiting its use in field applications, and the analysis can become costly both in terms of equipment and training.
Disadvantages
While laser flash analysis is a powerful tool, it does come with disadvantages. One major limitation is its dependence on precisely calibrated equipment and ideal sample conditions, which might not always be feasible in practical scenarios. The method also struggles with extremely low or high thermal conductivities, where other techniques might offer better accuracy. Thus, while highly effective, the choice to utilize laser flash analysis must be made with a thorough consideration of its potential drawbacks in relation to desired outcomes.
Comparative Analysis of Methods
Comparative analysis of thermal conductivity measurement methods serves as a crucial pivot in our understanding of how different approaches can meet various needs in the domain of material science. When it comes to assessing thermal conductivity, no one-size-fits-all route exists. Each method collects its share of benefits and limitations, making it imperative to evaluate these processes in a comparative light.
By systematically contrasting diverse techniques, researchers and practitioners can tailor their approach depending on the specific materials involved, desired precision, budget constraints, and operational conditions. A detailed review also highlights gaps in the current methodologies and fosters innovation, ultimately pushing the boundaries of thermal conductivity measurement.
Accuracy and Precision
Accuracy in thermal conductivity measurement is vital, as any deviation can lead to miscalculations in design and performance of materials. Precision, defined as the consistency of repeated measurements, also plays a significant role; it helps assure that the results are reliable across different trials.
When exploring various methods, one finds that:
- Guarded Hot Plate Method is generally recognized for its high accuracy in steady-state conditions. However, it struggles under transient conditions.
- Laser Flash Analysis, on the other hand, can offer significant precision but might falter when dealing with very heterogeneous materials.
Emerging Technologies in Thermal Conductivity Measurement
Emerging technologies in the realm of thermal conductivity measurement are paramount to advancing material science and engineering practices. The importance of these innovations cannot be overstatedâthey not only enhance the precision and efficiency of measurements but also expand the scope of materials that can be explored. This section will delve into two key areas: micro and nanostructured materials, as well as innovative sensor technologies, each playing a crucial role in how we understand and manipulate thermal properties for various applications.
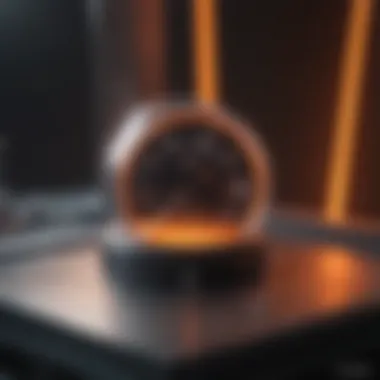
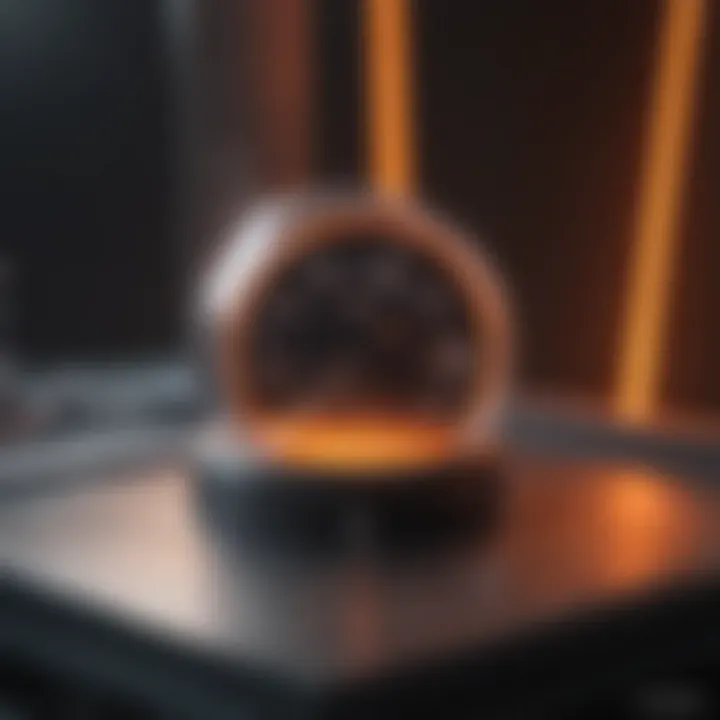
Micro and Nanostructured Materials
Micro and nanostructured materials have revolutionized many fields by exhibiting unique thermal properties compared to their bulk counterparts. At the nanoscale, materials can behave differently because of their increased surface area to volume ratio and quantum effects. This allows for fascinating thermal phenomena, like increased thermal resistance or even changes in heat conduction mechanisms.
The techniques to measure thermal conductivity in these materials often use advancements that help tackle the challenges presented by their tiny sizes. For instance, laser flash analysis can be adapted for thin films and nanoparticles, providing a clearer picture of heat transfer at smaller scales. Additionally, microfabricated sensors can deliver more localized measurements, crucial for understanding the thermal characteristics of materials that are often hidden in bulk forms.
"With the continued miniaturization of components in electronics, understanding thermal conductivity at the nano level is not just beneficial but essential."
Technologies like atomic force microscopy (AFM) with thermal probes are also gaining traction. These tools enable scientists to investigate thermal properties with unprecedented resolution, permitting researchers to explore potential applications in thermoelectric materials, where efficient heat-to-electricity conversion is necessary.
Innovative Sensor Technologies
Innovative sensor technologies are at the forefront of improving our ability to measure thermal conductivity. Recent advancements have seen the development of smart thermal sensors that integrate multiple functionalities. These sensors can provide real-time data, offering insights on how materials behave under various thermal conditionsâwhether they be high, low, static, or dynamic environments.
One example is the integration of fiber optic sensors, which utilize the change in light properties to gauge temperature and subsequently, thermal properties of materials. By embedding these sensors directly into materials during fabrication, researchers gain the ability to monitor changes in thermal conductivity in real-time, providing feedback on structural integrity or performance.
Moreover, wireless sensor networks are also making waves, allowing for extensive data collection without the physical cable constraints. This capability is particularly useful in remote sensing applications or in harsh environments where traditional measurement approaches might falter.
As we forge ahead, these technologies promise to unveil oddities and intricacies of thermal behavior across a range of applications, from aerospace engineering to renewable energy.
In summation, advancements in micro and nanostructured materials and sensor technologies are shaping the future landscape of thermal conductivity measurement. With their ability to capture data with unmatched precision and adaptability, these technologies stand to redefine our understanding of thermal characteristics in avant-garde materials.
Future Directions in Research
As we propel further into the 21st century, the quest for more efficient thermal conductivity measurement methods becomes paramount. In the ever-evolving landscape of materials science and engineering, the focus shifts towards improving accuracy and applicability of the methods we currently have. This section examines the importance of future directions in research regarding thermal conductivity measurements, delving into how these advancements can benefit industry and academia alike.
Integration of Measurement Techniques
Integrating various thermal conductivity measurement techniques can pave the way for more comprehensive insights into material properties. Using a multi-faceted approach can bridge the gaps that exist within individual techniques, thus enhancing both reliability and depth of analysis. Here are some key points to consider:
- Enhanced Accuracy: By synthesizing data from multiple methods, researchers can cross-verify measurements, leading to increased reliability. For instance, combining transient plane source and laser flash analysis could refine results for materials that exhibit complex thermal behaviors.
- Wider Applicability: Different materials might respond uniquely to measurement methods. When researchers integrate techniques, they gain the ability to cater to a broader spectrum of materials and conditions. This can be particularly useful in specialized industrial applications, such as aerospace or semiconductor fields, where precision is critical.
- Innovation: The amalgamation of various methodologies can inspire innovation, perhaps leading to new measurement techniques that take the best elements from existing ones. Think of this as modern-day alchemyâtransforming base measurement methods into something more valuable.
Interdisciplinary Applications
The interplay between thermal conductivity measurement techniques and various scientific disciplines illuminates a path to collaborative innovation. Each discipline brings a unique perspective, opening the door to joint advancements in understanding and application. Here are a few noteworthy aspects:
- Material Sciences and Nanotechnology: As we design materials at the nanoscale, understanding how thermal conductivity behaves becomes more complex. Collaborations between material scientists and nanotechnologists can stimulate the development of precise methods tailored for innovative nanostructured materials.
- Environmental Science: Thermal conductivity is a crucial parameter in studying heat transfer in soils and materials. Combining insights from geophysics with traditional thermal measurement techniques can enhance models predicting thermal behavior in ecological contexts, such as climate change effects on permafrost.
- Electronics and Semiconductor Industry: The push for more efficient electronic devices necessitates a deep understanding of thermal management. Researchers in electronics can collaborate with thermal engineers to optimize measurement methods that ensure device reliability and performance.
In summary, as research dives deeper into the nuances of thermal conductivity, leveraging multi-faceted techniques and fostering interdisciplinary collaborations can yield groundbreaking advancements. Such efforts will not only drive academic inquiry but also hold profound implications for various industries facing the challenges of next generation materials.
Finale
In the realm of material science and engineering, comprehending thermal conductivity measurement methods stands as a cornerstone for innovation and application. This article has laid bare the landscape of techniques available for assessing thermal conductivity, bridging theory with practical implications. Understanding these methodologies is not merely an academic exercise; it has far-reaching consequences across various industries such as construction, aerospace, and electronics.
Summary of Key Insights
A quick recap highlights several fundamental insights:
- Direct vs. Indirect Methods: The differentiation between direct measurement techniques, like the Guarded Hot Plate method, and indirect methods, such as Laser Flash Analysis, exemplifies the diverse approaches available. Each comes with its own set of advantages and challenges, making the choice context-dependent.
- Material Variability: Various factors affect thermal conductivity, including the microstructure and composition of materials. This variability necessitates a tailored approach when selecting a measurement method to provide accurate results.
- Emerging Technologies: The incorporation of micro and nanostructured materials is reshaping the landscape. Innovative sensor technologies are paving the way for more precise and rapid measurements, allowing for better performance evaluation in real-time applications.
"Understanding the right method for measuring thermal conductivity can lead to significant advancements in material performance and application outcomes."
Implications for Future Research and Development
The implications of thermal conductivity measurement extend beyond simple data collection; they open doors to future innovations in material design and application. Here are a few key areas for future exploration:
- Integration of Techniques: Research focusing on hybrid methods could combine the strengths of both direct and indirect approaches for even greater accuracy in various environments.
- Interdisciplinary Approaches: Collaborations across disciplines, such as nanotechnology and materials science, could yield breakthroughs in measuring techniques, especially for advanced materials that often defy conventional measurement methods.
- Sustainability Concerns: As industries strive for more sustainable practices, assessing thermal properties becomes increasingly crucial. Future research should emphasize methods that facilitate the use of sustainable materials in construction and manufacturing.
In essence, the investigation into thermal conductivity measurement methods not only enhances our scientific knowledge but also drives real-world applications that can significantly impact industries and daily life. The discussion surrounding future research underscores the need for ongoing exploration and adaptation to the ever-evolving landscape of material technology.