Monochlorobimane: Comprehensive Analysis and Applications
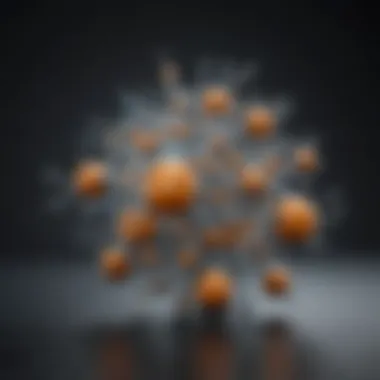
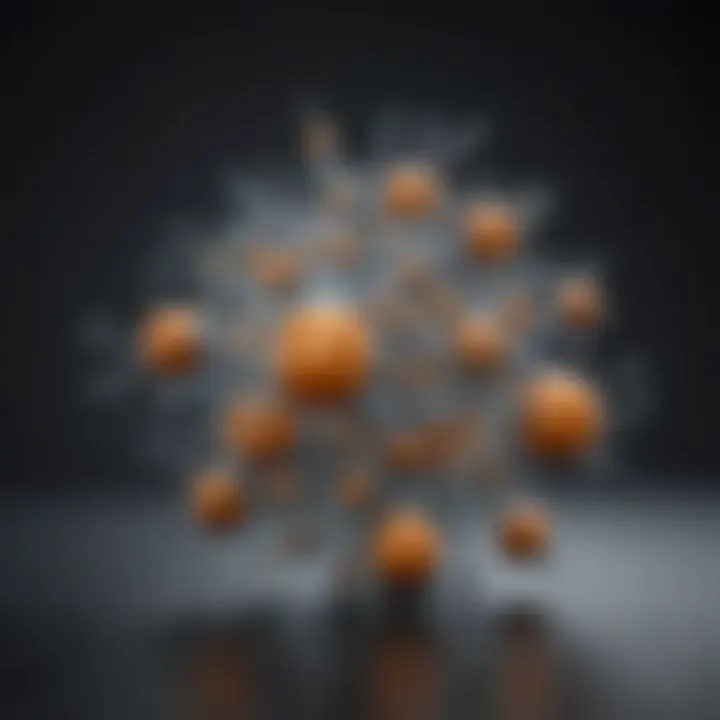
Intro
Monochlorobimane is a compound of significant importance in biochemical research. Its fluorescence and reactive properties make it a valuable tool for scientists. Understanding this compound can provide insight into its potential applications and implications for various biological systems. This section serves as an introduction into monochlorobimane, setting the stage for a deeper exploration into its synthesis, mechanisms, and applications.
Research Overview
Monochlorobimane has captured the attention of researchers due to its functionality. The compound is primarily recognized for its ability to monitor thiol status in living cells. This function allows for the examination of redox states and provides insights into several biological processes.
Methodological Approaches
Research involving monochlorobimane typically incorporates several methodological approaches. Common methods include:
- Fluorescence microscopy: This technique allows for the visualization of cellular processes in real time.
- High-performance liquid chromatography (HPLC): HPLC is used to separate and analyze compounds effectively.
- Mass spectrometry: This is vital for determining the structure of metabolites formed from monochlorobimane.
Using these techniques, researchers can gather data about the compound’s behavior in biological systems, enhancing our understanding of cellular dynamics.
Significance and Implications
The significance of monochlorobimane in research cannot be overstated. It has implications in various fields, including:
- Cell biology: By allowing the monitoring of thiols, chemicals like monochlorobimane enable insights into cellular redox environments.
- Drug discovery: It serves as a tool in the evaluation of compounds that affect cellular thiol levels.
- Therapeutic interventions: Research utilizing monochlorobimane can lead to better understanding of oxidative stress-related diseases.
Through these applications, monochlorobimane has established itself as an essential component in multiple avenues of scientific inquiry.
Current Trends in Science
The field is seeing innovative methods and connections arise as research progresses.
Innovative Techniques and Tools
In recent years, advancements in technology have broadened the application of monochlorobimane. Some notable trends include:
- Improved imaging techniques: Developments in fluorescence imaging have enhanced detection sensitivity.
- Automated analysis: New tools allow for high-throughput screening of compounds interacting with monochlorobimane.
These innovative methods are transforming the landscape of biochemical research.
Interdisciplinary Connections
Monochlorobimane exemplifies the connection between chemistry and biology. The compound not only serves as a crucial tool within biochemistry but also crosses into areas like:
- Pharmacology: Understanding cellular processes aids in drug development.
- Environmental science: Monitoring reactions in ecological systems can lead to insights into environmental stressors.
This intersection of disciplines fosters holistic approaches in research, allowing for comprehensive studies that can further elucidate complex biological phenomena.
Prolusion to Monochlorobimane
Monochlorobimane is a compound of great relevance in biochemical research, gaining significant attention due to its varied applications. This introduction aims to provide an understanding of why monochlorobimane is favored among researchers. Its utility spans across numerous fields, primarily in biological and biochemical contexts, making it essential in both academic and practical settings.
Monochlorobimane's key role lies in its ability to function as a fluorescent probe. This characteristic enables scientists to label proteins and visualize cellular processes with remarkable clarity. Moreover, its specific chemical properties allow for versatile applications, enriching experimental design and methodologies. Understanding the intricacies of this compound can inform better practices in labs and contribute to advancements in research.
In this section, we will explore the definition and chemical structure of monochlorobimane, followed by an overview of its historical background. These elements are crucial in establishing a solid foundation for appreciating the compound's significance in the broader context of biochemical research.
Definition and Chemical Structure
Monochlorobimane is a small molecule classified as a fluorophore. Chemically, it can be described as a derivative of bimane with a single chlorine substituent. The presence of the chlorine atom alters its photophysical properties, making it suitable for various applications in biological assays.
Its chemical formula is typically represented as C_9H_8ClN, signifying an aromatic compound that showcases an intriguing interaction between the chlorine atom and the rest of the molecular structure. This inclusion of chlorine enhances its reactivity and specificity—important characteristics for mechanisms in chemical biology.
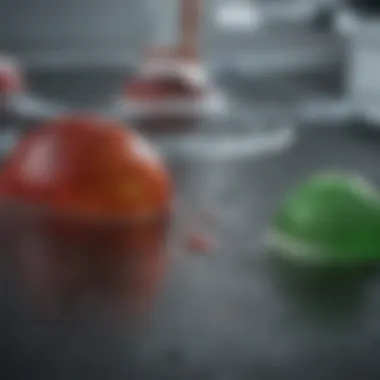
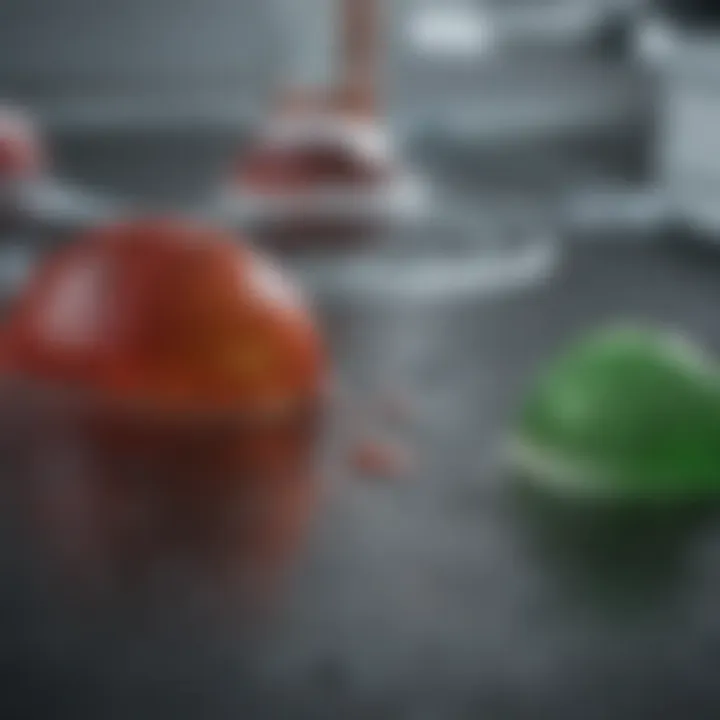
Historical Background
Historically, the developments surrounding monochlorobimane trace back to the growing need for effective fluorescent probes in biological research. The compound was first synthesized in the mid-20th century, with researchers aiming to create substances that could selectively bind to certain biomolecules. Throughout the years, scientists have optimized synthesis methods and characterized its properties, leading to increased interest in its applications.
As techniques in cell imaging and protein labeling advanced, monochlorobimane emerged as an important tool in understanding cellular dynamics and biochemical interactions. Its efficacy in detecting thiols further established its relevance, allowing researchers to probe complex biological systems with greater specificity. The journey of monochlorobimane reflects a continuous relationship between chemistry and biology, highlighting its ongoing importance in scientific inquiry.
Synthesis of Monochlorobimane
The synthesis of monochlorobimane is a crucial aspect of its study, providing insight into both its production methodologies and the compound's applications in biological research. An understanding of synthesis not only enables the creation of this important compound but also illustrates the chemical principles underlying its properties and uses. Proper synthesis methods can significantly affect the purity, yield, and effectiveness of monochlorobimane in various applications. Therefore, discussing the synthesis helps clarify its significance from both theoretical and practical perspectives.
Chemical Reactions Involved
The synthesis of monochlorobimane typically involves chlorination of a suitable precursor. One common precursor is butylated hydroxytoluene. During this reaction, a chlorine atom replaces a hydrogen atom in the aromatic ring of the compound. This reaction is often facilitated through electrophilic aromatic substitution, in which the chlorine acts as an electrophile. Details of the chemical reactions include:
- Reactants: Typical starting materials include butylated hydroxytoluene and a chlorine source such as chlorine gas.
- Reaction Conditions: The reaction is usually conducted under controlled temperatures to maintain stability and improve yield.
- By-products: Side reactions may produce undesirable by-products that could impact the purity of monochlorobimane.
In essence, several steps ensure that the final product meets the specifications required for its intended research applications.
Industrial vs Laboratory Synthesis
The synthesis of monochlorobimane can occur in both industrial and laboratory settings, each offering distinct advantages and considerations.
- Industrial Synthesis:
- Laboratory Synthesis:
- Scalability: Industrial methods can produce monochlorobimane in large quantities, making it suitable for widespread application in various sectors, including pharmaceuticals.
- Cost-Effectiveness: The processes ideal for scale often reduce the overall cost per unit of the product, thereby making monochlorobimane more accessible for research and practical uses.
- Regulatory Compliance: Industrial synthesis must adhere to stricter regulations due to the potential environmental impact and the safety standards required for large-scale production.
- Control Over Variables: This allows researchers to modify reaction conditions, thus optimizing the yield and purity of the compound for specific experiments.
- Flexibility: Laboratory settings support the synthesis of derivatives or modified compounds, enabling innovative research directions.
- Cost: While cost per unit is higher, the smaller scale allows for extensive experimentation with methods and reactions without significant financial risk.
Choosing between industrial and laboratory synthesis depends largely on the intended use of the monochlorobimane, available facilities, and the desired purity of the final product. Each approach has its merits and can be selected based on the specific requirements of a given project.
Properties of Monochlorobimane
The properties of monochlorobimane are crucial to understanding its utility in scientific research. This compound exhibits unique physical and chemical features that make it a versatile tool in biochemical applications. The following sections detail these properties, offering insights into how they influence its functions in research environments.
Physical Properties
Monochlorobimane is characterized by several distinct physical properties that contribute to its applications in research. It is a colorless to yellowish crystalline solid at room temperature, typically more soluble in organic solvents like dimethyl sulfoxide (DMSO) than in water. The melting point of this compound is approximately 86°C. This solubility allows for flexible usage in various experimental setups, making it a preferred choice among researchers.
Additionally, the compound also has a relatively high boiling point, which suggests a stable thermal behavior. Its specific gravity is about 1.1, indicating that it is denser than water. These physical properties help researchers manipulate monochlorobimane effectively in lab settings, allowing for accurate measurements and applications.
Chemical Properties
The chemical properties of monochlorobimane significantly influence its reactivity and functionality in biological interactions. As a halogenated compound, monochlorobimane can undergo nucleophilic substitution reactions. This characteristic is vital for its role in labeling biomolecules. The chlorine atom in monochlorobimane acts as a leaving group, enabling the compound to react with thiol groups present in proteins and other biomolecules, forming stable thioether bonds.
In addition, monochlorobimane is relatively inert under standard laboratory conditions. It has a tendency to remain stable at neutral pH levels, which is beneficial when conducting biological assays involving living cells. Researchers must consider these properties while planning experiments to ensure that the compound retains its integrity throughout various procedures.
Spectral Characteristics
Spectral characteristics of monochlorobimane are integral in its identification and quantification during experiments. The compound exhibits prominent fluorescence under UV light, which is essential for its application in cellular imaging. The emission maxima typically occur around 485 nm when excited at 400 nm. This unique fluorescence allows for easy visualization and quantification of thiol-containing biomolecules within cells.
The absorption spectrum of monochlorobimane also reveals useful peaks that researchers can utilize in analytical techniques. These spectral data aid in developing specific protocols to optimize the detection of monochlorobimane when used in various assays. Understanding these characteristics enhances the efficacy of monochlorobimane in providing reliable results in biological systems.
The unique properties of monochlorobimane make it an invaluable compound in biochemical research, particularly for studies involving protein labeling and cellular imaging.
In summary, the physical, chemical, and spectral properties of monochlorobimane underline its importance in scientific research. Familiarity with these properties is essential for researchers to utilize this compound effectively in their experiments.
Uses in Biological Research
Monochlorobimane plays a vital role in biological research, particularly within the fields of biochemistry and molecular biology. This compound is notable for its ability to interact with various biological molecules, primarily proteins and thiol groups. As researchers continue to explore its functionalities, monochlorobimane's significance only grows. The following subsections provide insights into its specific applications in biological systems.
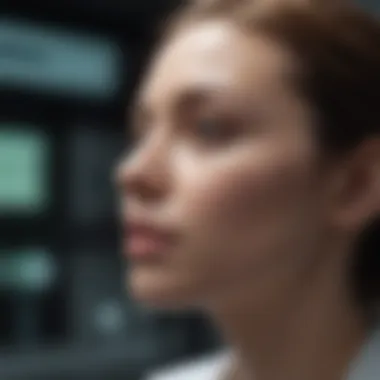
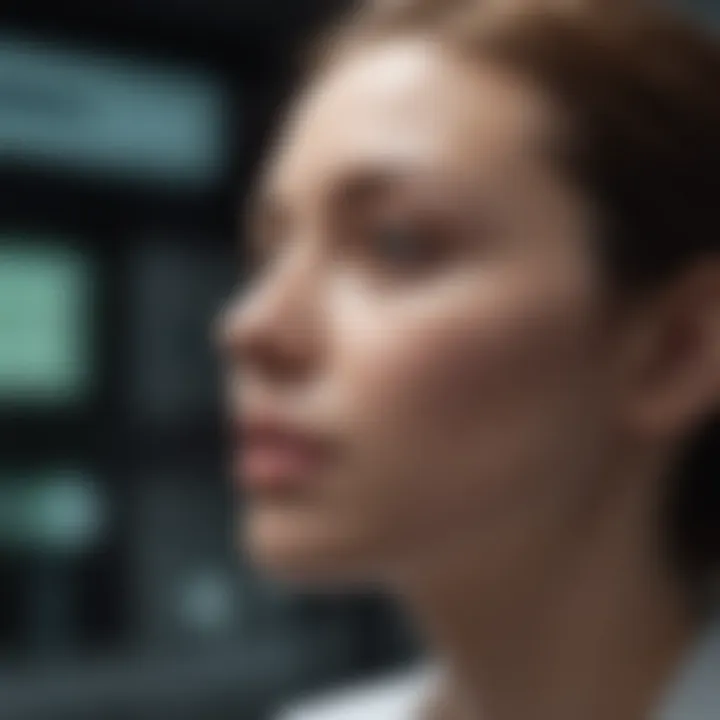
Role in Protein Labeling
One of the primary uses of monochlorobimane in biological research is its function in protein labeling. This process allows scientists to track and visualize proteins within cells. Monochlorobimane reacts with thiol groups located in the amino acids cysteine and glutathione. This reaction results in a fluorescent compound, enabling researchers to monitor protein dynamics in real-time. The advantages of using monochlorobimane over other labeling agents include its specificity for thiols and its relatively high quantum yield. As such, it provides clearer images with reduced background noise.
When employing monochlorobimane for protein labeling, several factors must be considered. Toxicity to cells, the stability of the fluorescent signal, and potential interference with protein function can all influence outcomes. Thus, experimental design must account for these elements to achieve reliable results. The labeled proteins can be analyzed via fluorescence microscopy or flow cytometry, making this labeling approach versatile for various research contexts.
Applications in Cellular Imaging
Monochlorobimane has become an essential tool for cellular imaging. Its fluorescent properties facilitate the visualization of cellular structures, processes, and interactions. By employing microscopy techniques, researchers can observe real-time cellular activities, allowing for a deeper understanding of cellular mechanisms.
In particular, researchers utilize monochlorobimane to study cell signaling pathways and the effects of external stimuli on cellular behavior. This level of detail is crucial for elucidating complex biological processes and could reveal new therapeutic targets. Furthermore, the compound's effectiveness in live-cell imaging makes it a preferred choice over others that might only work in fixed samples.
The incorporation of monochlorobimane in cellular imaging fundamentally enhances research capabilities, providing insights that were previously challenging to obtain.
Detection of Thiols
The detection of thiols within biological systems is another important application of monochlorobimane. Thiol groups are critical for many biological functions, including enzyme activity and protein folding. Monitoring thiol levels in biological samples can yield significant insights into cellular health and disease states.
Monochlorobimane enables the selective detection of thiols due to its reactivity with these compounds. When monochlorobimane reacts with thiols, it forms a highly fluorescent product, making it easier to quantify and visualize thiols in various biological contexts. This capability is particularly useful in oxidative stress studies, where the balance of thiol levels can indicate the health of the cell.
Researchers can employ different techniques for thiol detection, such as fluorescence spectroscopy or imaging. Thus, monochlorobimane serves as a useful biomarker in studying redox biology and exploring the implications of oxidative stress in various diseases.
Methodologies for Analyzing Monochlorobimane
Analyzing monochlorobimane is critical for its effective application in biological research. Understanding the methodologies used for analysis allows researchers to accurately utilize this compound for various experiments. The main techniques include fluorescence and chromatographic methods. Each technique has distinct advantages and considerations that can influence experimental outcomes.
Fluorescence Techniques
Fluorescence techniques are widely used in the analysis of monochlorobimane. These methods are based on the principle that the compound can emit light when excited by a specific wavelength. This property allows for sensitive detection of monochlorobimane in various biological systems. The most common applications involve monitoring thiol levels in cells or tissues.
One popular method in this category is fluorescence microscopy. It enables the visualization of cellular processes at a high resolution. Researchers can use this technique to observe the localization of monochlorobimane within the cell, revealing dynamic cellular events in real-time.
In terms of setup, the equipment is essential for successful implementation of fluorescence techniques. A good-quality microscope equipped with suitable filters is necessary. Additionally, careful calibration is important to avoid common pitfalls, such as photobleaching, which can compromise data integrity.
Chromatographic Methods
Chromatographic methods provide another avenue for analyzing monochlorobimane. These techniques separate compounds based on their physical or chemical properties. High-Performance Liquid Chromatography (HPLC) stands out as an effective method for this analysis. In HPLC, monochlorobimane can be isolated and quantified with precision, allowing for the determination of concentration in complex mixtures.
Using chromatographic methods generally involves preparing samples meticulously to ensure reproducibility. The choice of solvents is critical, as the right mobile phase can significantly improve separation and resolution. This aspect is particularly important when working with biological samples that may have variable composition.
In summary, both fluorescence and chromatographic methods are key to the analysis of monochlorobimane. They provide researchers with alternatives to explore its functionalities and applications in biological contexts. The decision on which method to use often depends on the specific goals of the research and the resources available.
"The careful selection of analytical methods significantly influences the reliability of research findings in biochemistry."
By employing these methodologies, scientific inquiry can deepen its understanding of monochlorobimane’s role in biological systems.
Limitations and Challenges
Understanding the limitations and challenges surrounding monochlorobimane is crucial for both its application and the interpretation of results in biochemical research. While monochlorobimane offers significant capabilities in protein labeling and thiol detection, researchers must remain vigilant of the inherent issues that may arise. Addressing these challenges ensures optimal use of this compound and enhances the reliability of experimental outcomes.
Stability Issues
One of the primary challenges associated with monochlorobimane is its stability. This compound can be sensitive to environmental factors, such as temperature and pH, which can affect its reliability during experiments. The degradation of monochlorobimane can lead to inaccurate results, making proper storage and handling vital.
- Light Sensitivity: Monochlorobimane is photosensitive, meaning that exposure to light can alter its chemical structure and reduce its effectiveness. Researchers must minimize light exposure during storage and experimental procedures.
- Temporal Stability: The compound's reactivity can diminish over time, especially if not stored correctly. This reduction in stability can impact experimental timing, where precise reactions are crucial.
To mitigate these issues, scientists often recommend regular calibration and careful monitoring of experimental conditions. Using appropriate controls can also enhance reliability.
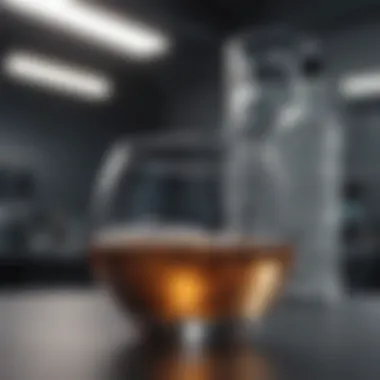
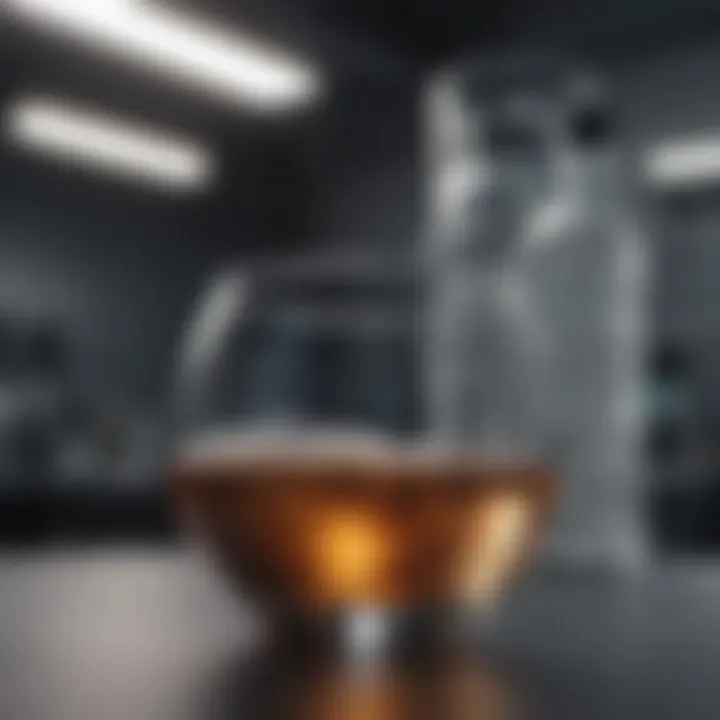
Specificity in Biological Systems
Another challenge lies in the specificity of monochlorobimane within biological systems. While it is a powerful tool for detecting thiols, there are limitations regarding its selectivity.
- Cross-Reactivity: Monochlorobimane can bind to various biomolecules, leading to potential interference in assays. Such cross-reactivity may yield misleading results when analyzing specific proteins or thiol concentrations.
- Complex Biological Environments: Cells and tissues are complex; presence of various thiols and other molecules can complicate results. This reality necessitates a thorough understanding of the biological context in which monochlorobimane is applied.
Ultimately, overcoming these specificity challenges requires careful experimental design, including controls that help account for possible interactions. By doing so, researchers can bolster the accuracy of their findings.
The awareness of monochlorobimane's limitations will enable researchers to fine-tune their methodologies to achieve more precise and reproducible results.
Emerging Applications
The study of monochlorobimane is evolving, with new applications emerging in various fields, particularly in biological sciences. This section aims to highlight the important developments in the area of emerging applications, contributing to a deeper understanding of monochlorobimane’s versatility and potential impact.
Novel Research Directions
Recent studies have focused on exploring how monochlorobimane can enhance our understanding of cellular processes. The compound's ability to selectively react with thiols offers significant insights into redox biology. One key area of investigation is its use in studies concerning oxidative stress, a condition that occurs when there is an imbalance between reactive oxygen species and antioxidants.
Moreover, researchers are exploring the utility of monochlorobimane in tracking protein dynamics. By utilizing its fluorescence properties, scientists can visualize and quantify the movement of proteins within cells. This advancement could significantly improve the understanding of diseases characterized by protein misfolding or aggregation, such as Alzheimer's disease.
"Monochlorobimane's role in thiol detection marks a paradigm shift in redox biology, unlocking new pathways for research that were previously inaccessible."
Another interesting direction is its evolving role in biosensing applications. The development of biosensors that use monochlorobimane could transform diagnostic approaches, enabling real-time monitoring of physiological changes in living organisms.
Potential in Therapeutic Development
Monochlorobimane's emerging applications are not limited to basic research; they also extend into therapeutic contexts. Its biochemical properties suggest potential applications as a drug carrier, particularly in targeted therapy where minimizing side effects is critical. By conjugating monochlorobimane with drug molecules, it may enhance the specificity of Drug Delivery Systems, thereby allowing more effective treatment options.
In addition, the applicability of monochlorobimane in redox modulation presents an exciting opportunity for drug development. Compounds that can restore redox balance may lead to novel treatments for diseases linked to oxidative damage. Targeted therapies that leverage monochlorobimane can be particularly vital in managing cancer, where oxidative stress plays a substantial role in tumor progression and resistance to treatments.
Furthermore, the exploration of monochlorobimane in nanomedicine is gaining traction. Researchers are investigating its compatibility with nanoparticles for drug delivery, which can significantly enhance drug solubility and bioavailability. This dual application, both as a fluorescent probe and as a component of drug delivery systems, highlights the compound's multifaceted nature in therapeutic development.
As the body of research grows, so does the potential impact of monochlorobimane in both therapeutic and diagnostic areas. The insights gained from studies will likely inform future iterations of biomedical solutions, making monochlorobimane a compound to watch in the coming years.
Future Prospects in Monochlorobimane Research
Monochlorobimane holds great promise for the future in scientific research. Its utility in various biological applications provides a foundation for further exploration. As researchers look for innovative tools in the study of cellular processes, monochlorobimane continues to attract attention. There are several key considerations in this field that may enhance its application.
Integrative Approaches
Integrative approaches are vital in maximizing the potential of monochlorobimane. Scientists are now focusing on combining monochlorobimane with other fluorescent probes. This enhances the specificity and sensitivity of detection methods. For instance, utilizing monochlorobimane alongside other dyeing agents can facilitate multi-color imaging. This is particularly helpful in understanding complex biological systems. By integrating monochlorobimane into existing methodologies, researchers can gain deeper insights into cellular mechanisms.
The following factors are crucial in integrative approaches:
- Collaboration across disciplines: Multidisciplinary teams can utilize a range of expertise to optimize the use of monochlorobimane.
- Adaptable protocols: Procedures must evolve to incorporate new findings and techniques without sacrificing reliability.
- Cross-validation of results: Combining different imaging techniques can lead to more robust conclusions from the data.
Technological Advances
Technological advances will shape the trajectory of monochlorobimane research substantially. Improvements in imaging technologies, for example, can reveal new dimensions of cellular activities. Advanced fluorescence microscopy techniques, such as super-resolution microscopy, enable researchers to observe phenomena at molecular levels with increased precision. As these technologies develop, the inherent characteristics of monochlorobimane can be explored in novel ways.
Additionally, the rise of AI and machine learning tools presents opportunities for analysis of large datasets generated by monochlorobimane studies. Researchers can leverage these tools to identify patterns or anomalies within complex biological data. These technologies allow for processing massive amounts of information efficiently.
In summary, future prospects in monochlorobimane research hinge on several elements: integrating approaches across disciplines, embracing technological advancements, and constantly evolving methodologies. The potential applications are vast, signaling an exciting future for this compound in the scientific community.
"Future research in monochlorobimane is not just about its current applications but also exploring its broader capabilities in new domains."
Developments in both integrative strategies and technology will undoubtedly provide new avenues for exploration in the realm of monochlorobimane.
Finale
The conclusion of this article serves as a synthesis of the content discussed throughout. It is vital not just as a summary but also as a reflection on the significance of monochlorobimane within biochemical research. The physical and chemical properties of monochlorobimane make it a versatile tool in various applications, including protein labeling and cellular imaging. Recognizing these features helps researchers understand its utility in specific experimental designs.
Summary of Key Findings
- Monochlorobimane shows unique reactivity towards thiols, making it a valuable resource in biological studies.
- Synthesis methods range from industrial techniques to laboratory-based approaches, allowing for flexibility in research environments.
- Applications extend beyond simple labeling; they include critical roles in imaging and detecting biological molecules.
- There are challenges in stability and specificity, which warrant careful attention in experimental setups.
- Emerging studies suggest that novel uses for monochlorobimane could redefine its relevance in major research fields.