Multiplex Immunofluorescence: Techniques and Future Directions
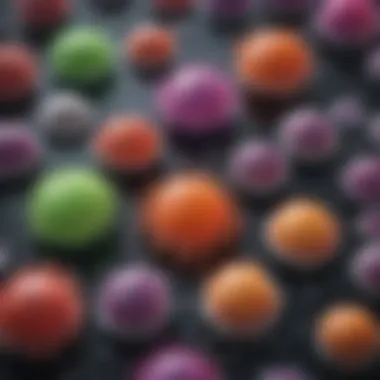
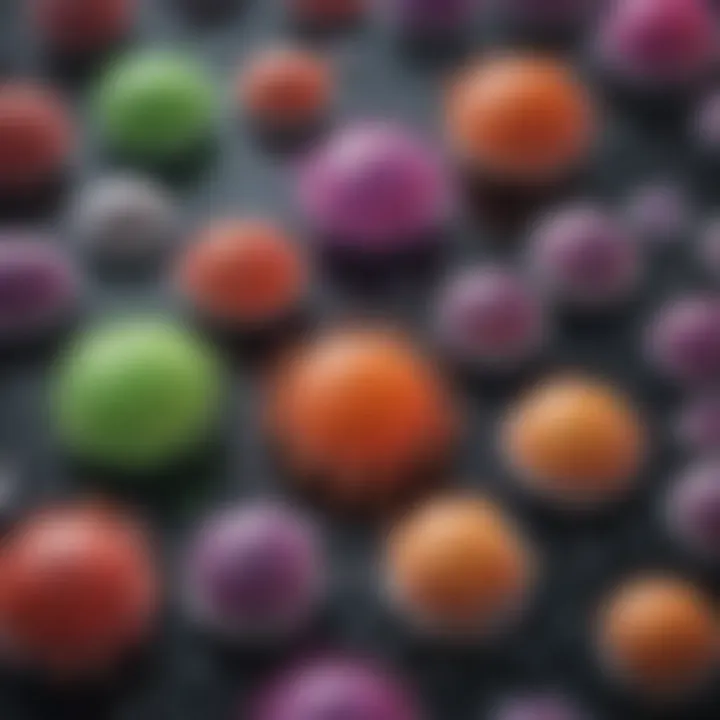
Intro
Multiplex immunofluorescence has emerged as a crucial technique in the domain of biological research and clinical diagnostics. This method allows scientists to visualize multiple targets within biological samples, facilitating a deeper understanding of cellular structures and functions. By employing various antibodies tagged with fluorescent dyes, researchers can identify and analyze specific proteins or antigens simultaneously. This capability is essential in diverse fields such as cancer research, neurobiology, and immunology, where complex interactions within cells and tissues demand a nuanced approach. This article will explore the foundational principles, applications, challenges, and future directions of multiplex immunofluorescence, aiming to provide a comprehensive overview of its significance in contemporary science.
Research Overview
Methodological Approaches
The methodology behind multiplex immunofluorescence relies on well-established principles of immunohistochemistry and fluorescence microscopy. Typical approaches involve the following key steps:
- **Sample Preparation: ** The biological samples, such as tissue sections or cell cultures, undergo fixation and permeabilization to preserve cellular integrity and enhance antibody accessibility.
- Antibody Labeling: Specific primary antibodies against target proteins are used. These antibodies can be directly labeled with different fluorophores or indirectly labeled using secondary antibodies.
- Imaging: Fluorescence microscopy techniques, such as confocal or multi-photon microscopy, enable the capture of images. Specialized software may be employed for image analysis and quantification.
While streamlined, multiplex immunofluorescence requires careful planning in terms of reagent selection and optimization of experimental conditions to avoid spectral overlap between fluorophores.
Significance and Implications
The ability to visualize multiple targets at once has significant implications for both research and clinical practice. For instance, in cancer diagnostics, multiplex immunofluorescence allows for the assessment of tumor markers alongside immune cell infiltration, providing insights into tumor microenvironments. Furthermore, this technique has the potential to advance personalized medicine by enabling clinicians to tailor therapies based on the distinct molecular profiles of individual patients.
Current Trends in Science
Innovative Techniques and Tools
The field of multiplex immunofluorescence is continually evolving with technological advancements. Recent innovations include:
- Spectral Imaging: This technique allows for the detection of more than four fluorescence signals simultaneously, increasing the number of targets analyzed per sample.
- Automated Systems: High-throughput imaging systems expedite sample processing and analysis, making multiplex immunofluorescence more accessible for large-scale studies.
- Artificial Intelligence: Machine learning algorithms are being developed to enhance image analysis, aiding in the accurate interpretation of results from complex datasets.
Interdisciplinary Connections
Multiplex immunofluorescence does not exist in isolation but intersects with various disciplines such as genomics, bioinformatics, and pathology. Such interdisciplinary collaboration enriches the research landscape, enabling scientists to approach problems from multiple angles and integrate insights from diverse fields. The combination of multiplex immunofluorescence with genomic data, for instance, can uncover novel pathways and therapeutic targets.
In summary, multiplex immunofluorescence represents a significant advancement in biological visualization techniques, offering extensive applications and promising future directions.
Such advancements pave the way for enhanced understanding of complex biological processes and contribute to the evolution of medical practices in an era where precision medicine is increasingly vital.
Preamble to Multiplex Immunofluorescence
Multiplex immunofluorescence is increasingly recognized as an essential technique in both research and clinical diagnostics. It enables the visualization of multiple targets within a single biological sample, promoting a more detailed understanding of cellular structures and functions. This method accommodates the exploration of complex biological systems by allowing simultaneous detection of various proteins, which is crucial in fields like cellular biology and oncology.
The technique's significance lies in its capacity to provide unparalleled insights into cellular interactions. Given the intricate nature of biological processes, examining several targets concurrently can lead to breakthroughs in understanding disease mechanisms. Moreover, incorporating this method into routine diagnostic practices has the potential to enhance patient outcomes through personalized medicine approaches.
Researchers and clinicians need to consider several elements about multiplex immunofluorescence. This involves understanding the fundamental principles, appropriate selection of antibodies and imaging technologies, and interpreting complex data sets. Also, awareness of potential technical challenges and limitations is vital for effectively leveraging this technique in varied contexts.
Definition and Principle
Multiplex immunofluorescence refers to a methodology that leverages antibodies labeled with different fluorescent dyes to detect multiple proteins in a single tissue or cell sample. The principle is straightforward: specific antibodies bind to their respective antigens, and the attached fluorophores emit light at varying wavelengths when excited by a light source. This light is detected and converted into an image that showcases the spatial distribution of proteins within the sample.
A critical aspect of this technique is the careful selection of fluorophores. Each fluorescent dye must have distinct excitation and emission spectra to ensure clarity and prevent overlap in the detection signals. The successful application of multiplex immunofluorescence relies heavily on meticulous planning and execution of experimental protocols.
Historical Context
The evolution of multiplex immunofluorescence stems from advancements in microscopy and antibody technology. Initial fluorescence techniques primarily allowed for the examination of single targets, limiting the depth of analysis. Over time, researchers recognized the need for improved methodologies that could provide more comprehensive insights into the complex interactions that underlie various biological phenomena.
In the early 2000s, breakthroughs in fluorescent probe development and imaging systems led to the emergence of multiplex capabilities. Innovations included the introduction of new fluorophores, improved detection technologies, and sophisticated imaging software, which together enabled the simultaneous visualization of multiple proteins within a single sample. This progress has fueled significant research advancements across disciplines, particularly in understanding tumor microenvironments and cellular signaling pathways.
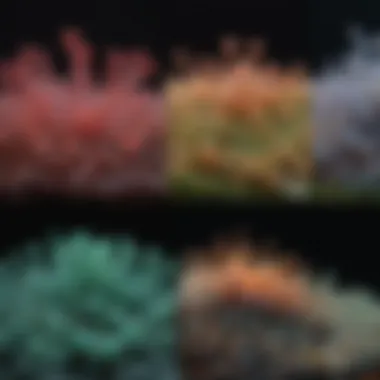
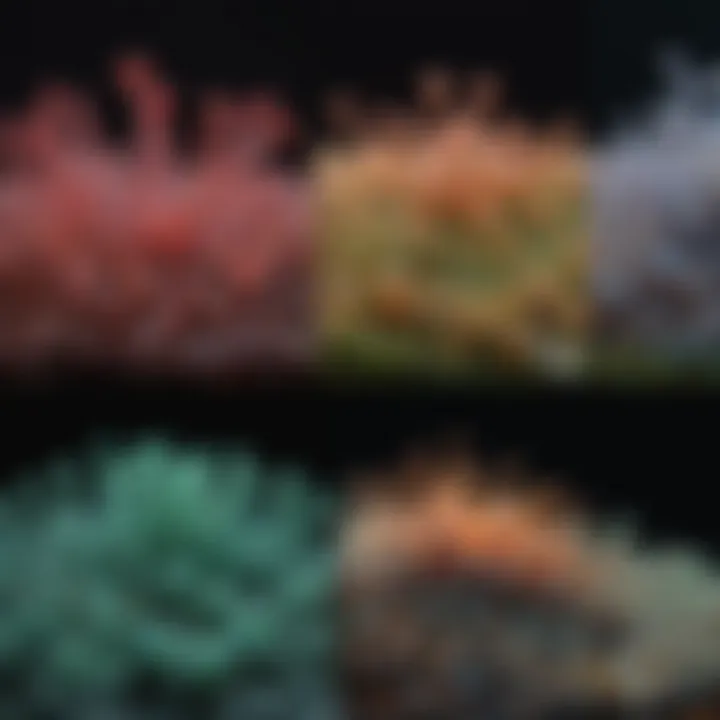
By examining the historical context of this field, one can appreciate the transformative impact of multiplex immunofluorescence in modern science.
Fundamentals of Immunofluorescence
Understanding the fundamentals of immunofluorescence is vital for mastering the multiplex approach. This section lays the groundwork by establishing key principles, essential for successful implementation of the technique. It encompasses important aspects like antibody labeling and the selection of fluorophores. The clarity in handling these components determines the reliability and accuracy of experimental results, making this understanding indispensable for researchers and educators in the field.
Basics of Antibody Labeling
Antibody labeling is a core element in immunofluorescence. It involves attaching a fluorescent dye to antibodies which recognize specific antigens in a sample. This process is crucial as it allows for the visualization of various target proteins within cells or tissues. There are two main types of labeling used. Direct labeling involves conjugating the fluorophore directly to the primary antibody. This method simplifies the procedure but may limit the range of available fluorophores. Indirect labeling, on the other hand, uses a fluorophore-conjugated secondary antibody that binds to the primary antibody. This increases amplification and can provide better signal strength.
- Considerations in Antibody Labeling:
- Specificity: Ensure that the antibodies chosen do not cross-react with other proteins.
- Fluorophore compatibility: The selected fluorophore should match the imaging system used, to avoid signal loss.
- Preservation: Handle labeled antibodies carefully to prevent degradation of the fluorophore.
By mastering these factors in antibody labeling, researchers can enhance the quality of their visual data significantly.
Fluorophores: Selection and Properties
Fluorophores are essential for producing a fluorescent signal in immunofluorescence. They absorb light at specific wavelengths and emit light at longer wavelengths. The choice of fluorophore is critical. Factors like brightness, photostability, and spectral properties need to be evaluated.
Some commonly used fluorophores include:
- Fluorescein isothiocyanate (FITC): Bright with a peak emission at 520 nm.
- Texas Red: Provides strong signal with emission around 615 nm, ideal for applications requiring longer wavelengths.
- Alexa Fluor dyes: Known for their high photostability and brightness across a range of wavelengths.
When selecting fluorophores, consider:
- Spectral overlap: Ensure that the emission spectra do not interfere with each other in multiplex experiments.
- Reactiveness: Assess the stability and reactivity of fluorophores to minimize background signals.
- Brightness: Opt for dyes that can provide a clear signal relative to the level of target proteins present in the sample.
To achieve optimal results in multiplex immunofluorescence, careful attention to the selection of fluorophores and their properties is crucial. The right choices will ensure enhanced visualization and data accuracy.
Techniques in Multiplex Immunofluorescence
The techniques utilized in multiplex immunofluorescence are fundamental to its success and relevance in both research and clinical settings. Effective execution of these techniques significantly enhances the ability to simultaneously visualize multiple antigenic targets within a single sample. This multiplexing capability is particularly beneficial as it allows researchers to gather more comprehensive data from a limited amount of tissue or cell sample. Understanding these techniques is crucial, as they determine the quality and interpretability of the results derived from multiplex assays.
Sample Preparation Methods
Sample preparation is critical in ensuring the integrity and quality of the immunofluorescence process. The quality of the tissue or cell sample directly affects the outcome of the experiments. Different types of preparation methods exist, each choice affecting resolution and signal performance.
Common approaches include formalin-fixed, paraffin-embedded (FFPE) samples, which are widely used due to their preservation of morphology and antigenicity. However, challenges arise as formalin fixation can mask antigen sites. Therefore, unmasking techniques, such as heat-induced epitope retrieval, become essential. Alternatively, fresh frozen sections can provide better antigen preservation, making them suitable for specific assays.
Proper handling during sample preparation is also paramount. Avoiding cross-contamination ensures the specificity and accuracy of results. It is also beneficial to optimize the stage of sample processing, including fixation time, permeabilization, and blocking, as these processes directly influence signal strength and background noise in the assays.
Imaging Protocols
Imaging is a crucial step in the multiplex immunofluorescence workflow. It entails the acquisition of images that capture the fluorescent signals from the labeled targets in the sample. The choice of imaging protocol can affect resolution and the overall quality of the data collected.
Fluorescence microscopy remains the primary imaging tool in multiplex assays. Different platforms exist, ranging from confocal microscopy to widefield and super-resolution systems. Each has its strengths in capturing high-resolution images from complex samples. For instance, confocal microscopy allows for the optical sectioning of samples, providing cleaner backgrounds and enhanced contrast. However, careful attention should be paid to the settings, such as illumination intensity and exposure time, to avoid photobleaching.
The calibration of imaging systems is also crucial. Systematic adjustments help maintain uniformity across samples and experiments. Regular maintenance and proper handling ensure optimal performance, reflecting in the clarity and quality of acquired images. Incorporating automated imaging systems further enhances reproducibility and accuracy in high-throughput studies.
Data Acquisition Techniques
The analysis of fluorescence data involves sophisticated data acquisition techniques. These techniques ensure the signal captured from the samples is processed correctly to yield meaningful results. Unique challenges arise in the context of multiplex assays given the simultaneous detection of multiple targets, requiring meticulous data interpretation.
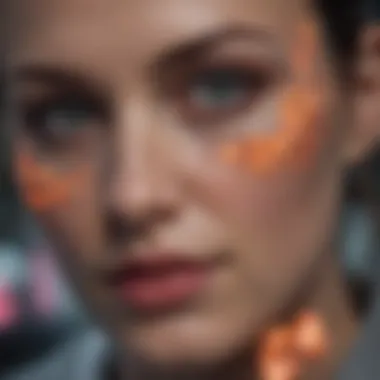
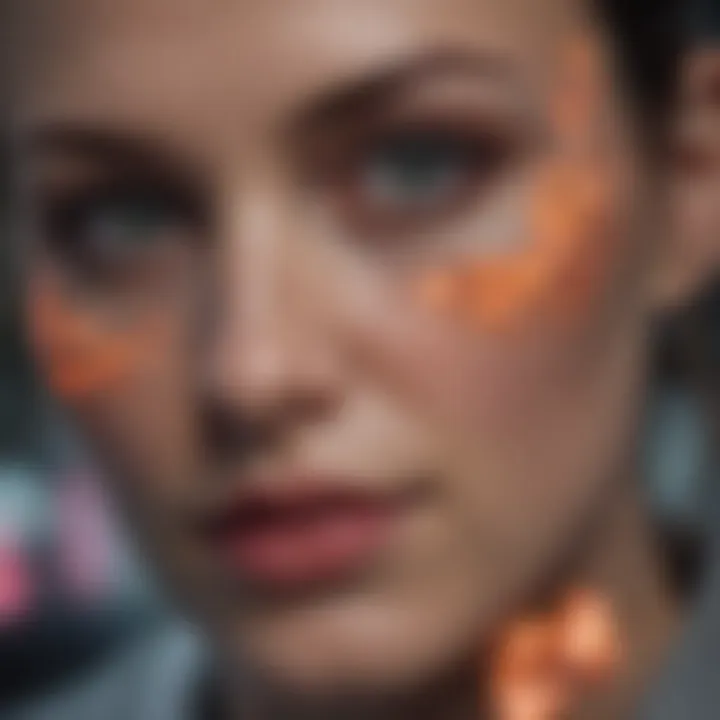
Common data acquisition methods include spectral imaging and multi-channel detection. These techniques enable the discernment of overlapping signals from different fluorophores. Implementing appropriate software aids in spectral unmixing, a process that distinguishes between overlapping fluorescence emissions. The choice of software can influence the efficiency of data processing and analysis, thus affecting the conclusions drawn from the experiments.
Applications in Cellular Biology
Multiplex immunofluorescence represents a pivotal advancement in the realm of cellular biology. This technique affords researchers the ability to observe and analyze multiple target proteins simultaneously within a single tissue section or cell. As a result, it enhances the understanding of complex cellular interactions and biological processes, which is crucial for elucidating the underlying mechanisms of various diseases. The utility of this method extends across several domains such as developmental biology, neuroscience, and cancer research, contributing significantly to advancements in these fields.
Tissue Microenvironment Studies
The tissue microenvironment plays a significant role in cellular behavior, influencing processes such as development, tissue repair, and disease progression. Multiplex immunofluorescence allows scientists to visualize different cell types and their spatial relationships within the tissue context. By simultaneously tagging multiple markers, researchers can investigate the interactions between various cell populations in their native conditions.
This approach provides a detailed view of cellular landscapes, particularly in heterogeneous tissues, where not all cells express the same markers. Key studies harnessing this method have revealed critical insights into tumor microenvironments, facilitating a deeper understanding of how cancer cells interact with surrounding stromal and immune cells. Such insights can lead to the identification of novel therapeutic targets.
"Understanding the tissue microenvironment is crucial for the development of effective therapies. Multiplex immunofluorescence offers a comprehensive view of these complex interactions."
The consideration of multiple factors simultaneously enables a more holistic view of biological systems. In the context of regenerative medicine, this technique aids in deciphering how stem cells or progenitor cells communicate with their microenvironments to promote tissue regeneration.
Cell Signaling Pathways
Cell signaling pathways govern various cellular functions, from metabolism to proliferation and apoptosis. Analyzing these intricate pathways requires precise and simultaneous detection of multiple signaling proteins. Multiplex immunofluorescence is integral in this regard, as it provides a platform to observe how different signaling molecules interact and regulate each other's activity.
For example, researchers can study the interplay between growth factors, receptors, and downstream effectors within a single experiment, thereby gaining insight into the mechanisms that drive diseases like cancer and neurodegeneration. By mapping these interactions spatially and temporally, scientists can better understand how signaling pathways are altered in diseased states compared to normal conditions.
Furthermore, this approach aids in identifying biomarkers for diseases and their progression, which is paramount for personalized medicine. The depth of information obtained through multiplex immunofluorescence in cell signaling studies can refine therapeutic strategies aimed at restoring or manipulating cellular functions.
In summary, applications in cellular biology highlight the versatility and power of multiplex immunofluorescence. From analyzing tissue microenvironments to dissecting complex signaling pathways, this technique has become an invaluable tool for researchers. Its capacity to provide a multifaceted view of biological phenomena positions it as a cornerstone in advancing our understanding of cellular processes.
Clinical Diagnostics and Research
Multiplex immunofluorescence plays a crucial role in both clinical diagnostics and research settings. By enabling the simultaneous detection of various biomarkers within a single sample, this technique delivers extensive information that is invaluable for understanding complex biological systems and disease processes. The ability to visualize multiple targets concurrently allows for a more comprehensive analysis of cellular environments. This is particularly beneficial in clinical settings, where timely and accurate diagnoses can make a significant difference in patient outcomes.
Role in Oncology
In oncology, multiplex immunofluorescence has transformed the way tumors are analyzed. This technique allows researchers and clinicians to evaluate the expression of different proteins involved in tumor progression, immune response, and metastasis. Different markers can indicate specific tumor types or the likelihood of response to treatments.
- Personalized Medicine: By identifying multiple targets, clinicians can customize treatment plans tailored to the individual tumor profile. This approach enhances the effectiveness of therapies and reduces unnecessary side effects.
- Tumor Microenvironment: Understanding the interactions between tumor cells and surrounding tissue is essential. Through multiplexing, researchers can visualize the tumor microenvironment, providing insights into how immune cells infiltrate tumors and affect their behavior.
Thus, multiplex immunofluorescence is an essential tool in research and therapeutic protocols for cancer treatment, contributing significantly to advancements in precision medicine.
Autoimmune Diseases Analysis
Multiplex immunofluorescence is also instrumental in the study of autoimmune diseases. It permits the evaluation of multiple autoantibodies and the assessment of immune cell subsets involved in disease pathology.
- Comprehensive Profiling: Utilizing this technique, researchers can generate detailed profiles of autoantibody levels in diseases such as lupus and rheumatoid arthritis. This data can improve the understanding of disease mechanisms.
- Biomarker Discovery: The identification of novel biomarkers is critical. Multiplex immunofluorescence helps in revealing specific markers that correlate with disease activity or progression, which may lead to better targeted therapies.
The use of multiplex immunofluorescence facilitates a holistic understanding of autoimmune conditions, providing insights that are often missed when evaluating singular biomarkers.
In summary, multiplex immunofluorescence holds immense potential in clinical diagnostics and research. Its applications in oncology and autoimmune disease analysis demonstrate its importance in enhancing our understanding of complex biological interactions and improving patient care.
Technical Challenges and Limitations
In the field of multiplex immunofluorescence, understanding the technical challenges and limitations is crucial for researchers. As this technique becomes more prevalent in biological and biomedical research, recognizing these hurdles can enhance the accuracy and reliability of the results obtained. Addressing these issues ensures that multiplex immunofluorescence can be utilized effectively, ultimately broadening its applications in various domains such as clinical diagnostics and experimental biology.
Cross-Reactivity Issues
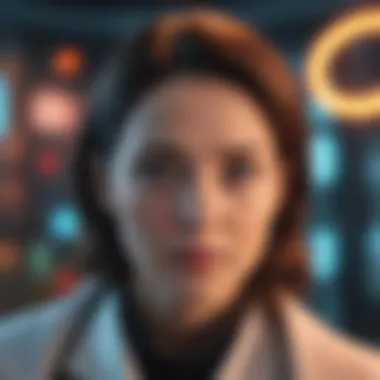
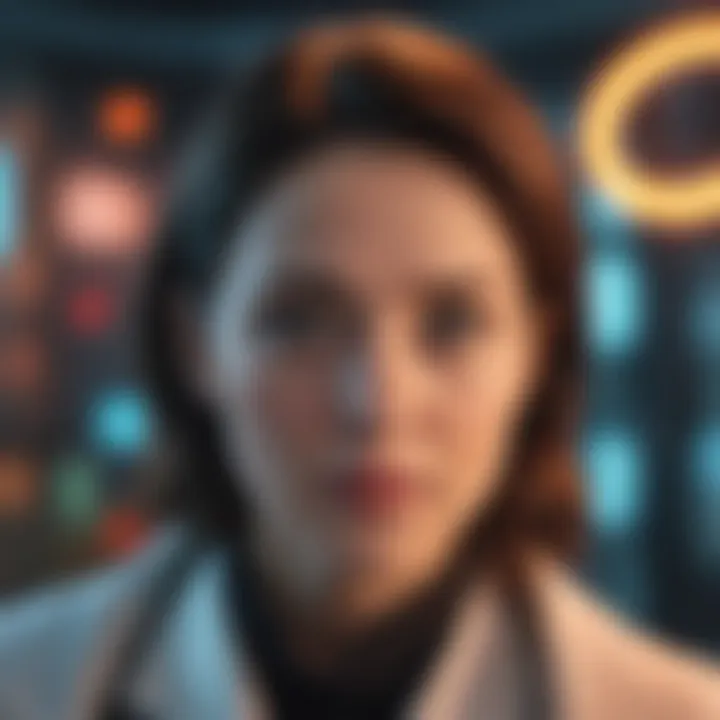
One of the prominent technical challenges in multiplex immunofluorescence is cross-reactivity. This occurs when antibodies bind to unintended targets, leading to false-positive signals. Cross-reactivity can significantly skew results, especially when analyzing complex tissues where multiple antigens are present.
Researchers must carefully select antibodies that show high specificity for their targets. It is also essential to run appropriate controls to mitigate the impact of cross-reactivity. This can include the use of isotype controls and verifying binding specificity through techniques such as western blotting or ELISA. A thorough understanding of the antibodies used in multiplexing is critical.
Ultimately, minimizing cross-reactivity improves the signal-to-noise ratio, allowing for more precise interpretation of results. It further aids in decoding cellular environments accurately, which is vital for advancing research and diagnostic applications.
Signal Overlap and Interpretation
Signal overlap is another technical limitation that adds complexity to multiplex immunofluorescence. This issue arises when two or more fluorescent signals coincide, leading to difficulties in distinguishing individual signals. With increased numbers of targets in a sample, managing the spectral properties of the fluorophores becomes increasingly challenging.
Researchers must carefully select fluorophores with distinctly different emission spectra. This strategy is essential to avoid confusion when analyzing images. Advanced imaging software can help in quantifying and deconvoluting overlapping signals, but this often requires a steep learning curve.
The ability to accurately interpret overlapping signals is vital for drawing reliable conclusions from multiplex immunofluorescence experiments. It is important for scientists to remain informed about the latest software advancements and techniques that aid in overcoming this hurdle.
Understanding and addressing signal overlap can enhance quantitative data analysis and lead to better insights into cellular mechanisms. A well-structured experimental design that considers these limitations will yield more robust and interpretable data, thus impacting research outcomes positively.
Future Directions in Multiplex Immunofluorescence
Future directions in multiplex immunofluorescence represent an essential aspect of ongoing research and application in this field. The technique has experienced significant advancements, yet there remains considerable room for development. Understanding these future trends is crucial for researchers aiming to enhance their methodologies and integrate new technologies.
This section delves into two primary areas of advancement: technological improvements and integration with other techniques. Each of these components plays a vital role in expanding the utility of multiplex immunofluorescence for scientific inquiry and clinical applications.
Technological Advancements
The landscape of multiplex immunofluorescence is shaped by continuous technological advancements. New tools and techniques enhance the resolution and specificity of this method. For instance, improvements in fluorophore chemistry allow for brighter and more stable dyes, which is beneficial for detecting targets with low expression levels. Additionally, advancements in imaging systems have enabled higher throughput and better sensitivity.
Some notable technological innovations include:
- Ultrasensitive detectors: These allow for the capture of faint signals, improving sensitivity in detection.
- Automated imaging systems: Automation speeds up the process of acquiring images from multiple samples, increasing efficiency.
- High-dimensional data analysis: Emerging software tools enable researchers to analyze complex datasets more effectively, providing deeper insights into biological processes.
By continuing to prioritize these advancements, researchers can explore previously unattainable aspects of cellular biology.
Integration with Other Techniques
Incorporating multiplex immunofluorescence with other techniques can significantly enhance its effectiveness. The merging of different methodologies creates more comprehensive analytical frameworks. This fusion allows for a multi-faceted view of biological phenomena, integrating multiple dimensions of data.
Key integrations may include:
- Single-cell RNA sequencing: This can complement multiplex immunofluorescence data, providing insights into gene expression at the single-cell level while visualizing protein localization.
- Mass cytometry: Combining these two methods provides both protein and cellular signaling data, adding layers of comprehension to cellular behavior.
- In vivo imaging technologies: This enhances the understanding of biological processes in their physiological context, facilitating translation from bench to bedside.
Such integrations promise to propel future applications in personalized medicine by enabling researchers to uncover detailed mechanisms of diseases and tailor therapeutic strategies accordingly.
"The future of multiplex immunofluorescence lies not just in improved techniques, but also in its integration with complementary methodologies to provide a holistic understanding of biological systems."
The End
The conclusion serves as a crucial segment of the article, encapsulating the central themes related to multiplex immunofluorescence. It emphasizes the significant role this technique plays in advancing both fundamental and applied biological research. Multiplex immunofluorescence enables scientists to observe intricate interactions within cellular contexts, thus enhancing our understanding of complex biological systems and diseases.
Summarizing Key Insights
Multiplex immunofluorescence represents a leap forward in the study of cellular biology. Key insights include:
- Simultaneous Target Visualization: This method allows for multiple targets to be visualized concurrently, reducing variability and improving the quality of data obtained.
- Enhanced Understanding of Cellular Interactions: By employing this technique, researchers gain insights into cell signaling pathways and tissue microenvironment dynamics, contributing to a deeper understanding of cellular behaviors and disease progression.
- Identification of Biomarkers: The ability to detect several markers within a single sample streamlines biomarker discovery, crucial for both diagnostics and therapeutic development.
Implications for Future Research
Looking ahead, the impact of multiplex immunofluorescence on future research is profound. It opens pathways to:
- Personalized Medicine: As this technology evolves, its integration with patient samples could lead to personalized treatment plans based on specific tumor profiling.
- Combination with Emerging Technologies: The synergy between multiplex immunofluorescence and other techniques, such as single-cell sequencing, could provide unprecedented insights into cellular heterogeneity.
- Innovative Research Applications: Expanding the range of detectable targets makes multiplex immunofluorescence a promising technique for studying complex diseases, such as neurodegeneration and various cancers.
In summary, as researchers continue to refine multiplex immunofluorescence techniques and address existing limitations, the future of this technology in scientific inquiry looks promising. The continued exploration of its potentials can lead to transformative advancements in cellular and molecular biology.