Neon Transfection Protocol: An In-Depth Guide
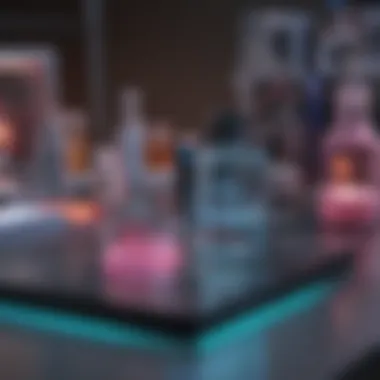
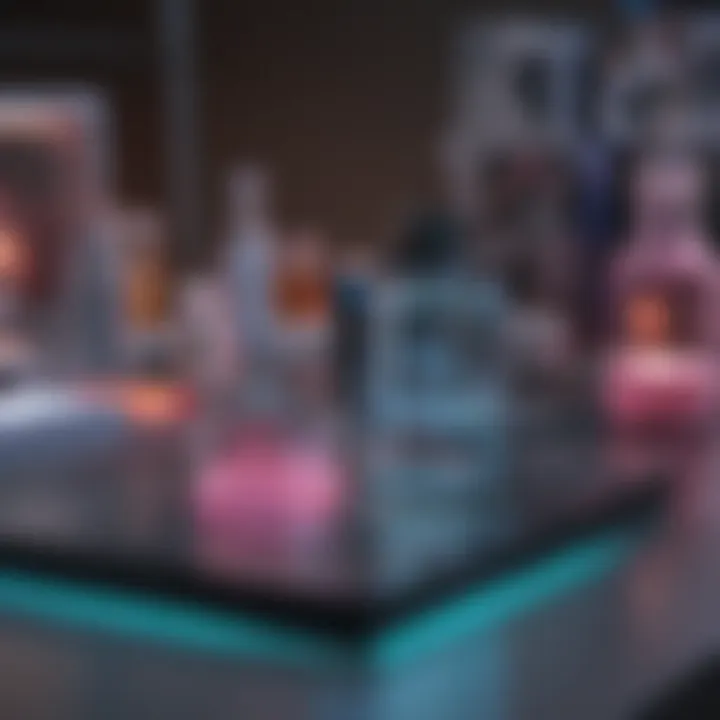
Intro
The neon transfection protocol stands as a fundamental technique in molecular biology, providing a reliable method for gene delivery into various cell types. Understanding this protocol is crucial for researchers aiming to manipulate genetic material for experimentation or therapeutic purposes. This article aims to delve into the nuanced steps involved in the neon transfection process, alongside the methodology, implications, and current trends in the field.
Essentially, the protocol harnesses electrical pulses to facilitate the uptake of plasmid DNA into cells. This process, known as electroporation, capitalizes on temporary permeabilization of the cellular membrane, allowing for the introduction of genetic material. As researchers explore this technology, they uncover its growing relevance across diverse applications in genetics and biotechnology.
Research Overview
Methodological Approaches
The neon transfection protocol incorporates specific methodological tactics designed to enhance transfection efficiency. Typically, the procedure begins with cell preparation, which involves selecting appropriate cell types and optimizing their confluency. Following this, one must mix the plasmid DNA with the cells in a specialized electroporation buffer.
The next step involves setting the parameters of the electroporator. Adjustments include pulse duration and voltage, both critical factors in determining the overall success rate of transfection. Upon completion of the electric pulse, cells require careful recovery in growth media, which helps them stabilize and express the introduced genes effectively.
Significance and Implications
The neon transfection protocol offers several significant advantages compared to traditional methods. It allows for a higher transfection efficiency, particularly in hard-to-transfect cell types. Moreover, its ability to introduce larger plasmid constructs makes it a powerful tool for complex gene studies. These characteristics open doors for research in gene therapy, cancer research, and genetic engineering, providing profound implications for potential medical advancements.
"Neon transfection represents a critical advancement in our ability to manipulate genetic material with precision in the laboratory setting."
The efficiency and expanded applications signify its growing importance in molecular biology. As researchers harness the power of this protocol, they contribute to advancements in genetics and a deeper understanding of cellular mechanisms.
Current Trends in Science
Innovative Techniques and Tools
Recent developments in neon transfection have seen a rise in techniques and tools that further streamline the process. Innovations such as high-throughput transfection systems enable the parallel processing of multiple cell samples. These advancements make it feasible to perform large-scale experiments with varying genetic constructs, expediting research outcomes.
Researchers are also integrating automation into the neon transfection workflow. By utilizing robotic systems, the precision and reproducibility of experiments improve significantly, reducing the likelihood of human error and bias.
Interdisciplinary Connections
The neon transfection protocol is increasingly finding applications beyond molecular biology, bridging fields such as pharmaceuticals, diagnostics, and regenerative medicine. In essence, the integration of neon transfection with other disciplines fosters collaborative efforts aimed at tackling complex biological questions. The outcomes of such interdisciplinary approaches may lead to enhanced therapeutic strategies and an expansion of our biological knowledge.
As we explore this protocol further, it becomes clear that the neon transfection technique is not only a methodological cornerstone but also a facilitator of innovation and advancement within the scientific community. The potential for future applications continues to be vast, underscoring the necessity for ongoing research and exploration within this domain.
Prologue to Neon Transfection
The field of molecular biology has made significant advancements due to the development of various techniques for gene delivery. Among these, Neon Transfection stands as a particularly effective method. This technique allows researchers to introduce nucleic acids into cells with high efficiency while maintaining cell viability. The importance of Neon Transfection lies in its applications across diverse areas such as gene therapy, genetic engineering, and protein production. Understanding its foundational principles, historical context, and practical applications is crucial for anyone involved in modern biological research.
Definition and Importance
Neon Transfection can be defined as a method that utilizes high-voltage pulses to facilitate the introduction of plasmid DNA or RNA into cells. This technique is notable because it combines the advantages of electroporation with the user-friendly nature of specific transfection systems. One key benefit of this method is its ability to transfect a wide range of cell types, including difficult-to-transfect primary cells. Furthermore, it minimizes the toxicity often associated with other transfection techniques, resulting in higher cell viability rates post-transfection.
The relevance of Neon Transfection is particularly evident in experimental setups requiring precise gene manipulation. For example, researchers investigating gene function, pathways, or therapeutic targets can employ Neon Transfection to introduce constructs effectively. The ability to achieve high transfection efficiency significantly enhances the quality and reliability of the results, making it a valuable tool in both academic and clinical laboratories.
Historical Background
The evolution of transfection methods has been shaped by the ongoing pursuit of enhancing gene delivery techniques. Traditional methods such as calcium phosphate transfection and lipid-based systems laid the groundwork for future innovations. However, these methods often posed limitations, particularly concerning efficiency and cell toxicity.
The introduction of electroporation marked a significant milestone in the transfection landscape. This technique allowed for the use of electric fields to facilitate the uptake of DNA into cells, greatly increasing transfection efficiency. Over time, improvements in electroporation technology led to the development of specialized devices like the Neon Transfection System. Launched by Thermo Fisher Scientific, this system has integrated user-friendly features with precise control over transfection parameters. Today, Neon Transfection is recognized as a leading method in molecular biology labs worldwide, paving the way for cutting-edge research and therapeutic developments.
Principles of Neon Transfection
Understanding the principles of neon transfection is essential for anyone seeking to implement this method effectively in research or practical applications. Neon transfection offers a unique mechanism for delivering nucleic acids into cells, which enhances genetic studies and therapeutic developments. The core principles encompass not just the technical aspects but also the considerations regarding efficiency and cell viability.
Mechanism of Action
The mechanism of action for neon transfection primarily relies on electroporation, where an electrical field is applied to drive DNA or RNA into target cells. When cells are subjected to an electric field, transient pores form in the cellular membrane. This allows nucleic acids to enter the cytoplasm effectively. The size of the pores and the overall success of transfection depend on the parameters set during the procedure, such as voltage and pulse duration. The method is notable because it minimizes the need for chemical agents that may affect cell health or be cytotoxic at high concentrations. This direct approach facilitates the introduction of various types of nucleic acids, making neon transfection versatile for different experimental setups.
Comparison with Other Transfection Methods
When considering transfection methods, it is critical to understand how neon technology stands in relation to others like chemical transfection, electroporation, and lipid-based systems.
Chemical Transfection
Chemical transfection involves the use of reagents to facilitate the entry of nucleic acids into cells. One significant aspect of chemical transfection is its reliance on cationic polymers or liposomes, which can form complexes with DNA. This method is popular due to its simplicity and relatively low cost. However, its main drawbacks include potential cytotoxicity and lower transfection rates in primary cells or certain cell lines. In summary, chemical transfection is useful for many applications, but it may not always provide optimal results compared to neon transfection.
Electroporation
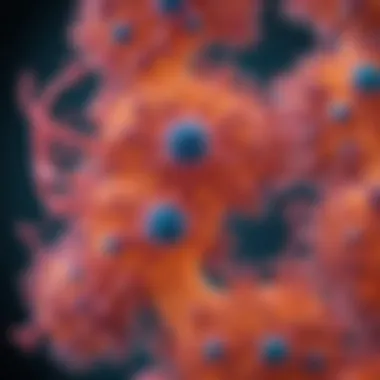
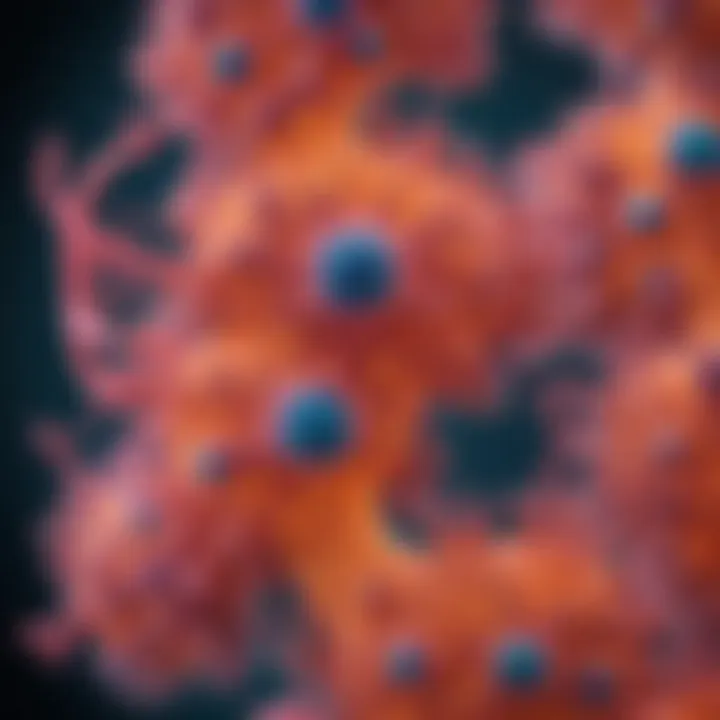
Electroporation, similar to the neon transfection technique, also uses an electric field to deliver nucleic acids. A key characteristic of electroporation is its adaptability to various cell types. It is highly effective for hard-to-transfect cell lines. However, the primary disadvantage can be the complexity of optimizing parameters, which can vary significantly across different cell types. Neon transfection simplifies this by providing standardized settings that are replicable, thus ensuring more consistent results.
Lipid-Based Systems
Lipid-based transfection systems employ lipid nanoparticles to encapsulate nucleic acids, facilitating their fusion with the cell membrane. A significant advantage of these systems is their low toxicity and ease of use. They are particularly beneficial for in vivo applications and have shown effectiveness in delivering mRNA. On the downside, lipid-based systems may not be as efficient as electroporation in delivering large plasmids or complex nucleic acid systems. Neon transfection provides a compelling alternative by delivering larger constructs with ease.
Neon transfection, positioned within the broader framework of transfection methodologies, offers unique advantages while addressing some common limitations associated with other methods. This understanding shapes better experimental designs and implementation in biological research.
Materials Required for Neon Transfection
Neon transfection represents a significant advancement in gene delivery techniques, making the selection of proper materials crucial to its success. Understanding these components can drastically affect experimental results and overall efficiency. This section will explore the essential materials needed for neon transfection and their relevance to the procedure.
Cell Culture Components
Cell culture serves as the foundation for effective transfection. Using high-quality cell culture components is vital to ensure cells remain healthy and proliferate properly. The primary components include:
- Culture Medium: This provides essential nutrients for cell growth. Using media such as DMEM or RPMI-1640 is common.
- Fetal Bovine Serum (FBS): Supplementing the medium with FBS improves cell viability and growth rates. Be sure to select serum from reputable sources to avoid contamination.
- Antibiotics: Adding penicillin-streptomycin can help prevent bacterial contamination. This is particularly important when working with sensitive transfection processes.
It is important to routinely check the pH and osmolality of your culture medium, as deviations can impair cell health. Proper storage and handling of these components cannot be overlooked; they should be stored correctly to maintain their sterility and efficacy.
DNA Preparation
The DNA used in the transfection process directly influences gene expression outcomes. Here are the key points to consider:
- Purity of DNA: High-purity plasmid DNA is important and should be purified using methods like phenol-chloroform extraction or commercially available kits. Contaminants can interfere with transfection efficiency.
- Concentration: The optimal DNA concentration typically ranges from 1 to 5 µg per 100,000 cells. Adjusting this concentration based on cell type is essential for maximizing uptake.
- Formulation: Formulating DNA with appropriate buffers can help stabilize the plasmid and enhance transfection outcomes.
Striking a balance between purity and concentration can be the difference between success and failure in your transfection endeavors. Regular quality checks of your DNA preparations will ensure consistent results in your experiments.
Neon Transfection System Equipment
The success of the neon transfection protocol hinges on the proper use of specialized equipment. Some of the essential components include:
- Neon Transfection System: This device generates electrical pulses necessary for the electroporation process. It must be calibrated according to the specific requirements of your protocol.
- Electroporation Cuvettes: These are designed to hold cells and DNA, allowing the delivery of electrical pulses. Use cuvettes with the correct dimensions to ensure uniformity during electroporation.
- Cooling System: A cooling system is often needed to help maintain optimal temperatures during the transfection process.
Proper maintenance and handling of this equipment are crucial for performance. Regular checks and calibration of the Neon Transfection System ensure reliable results across repeated experiments.
Efficient preparation of materials directly correlates with the success rate of neon transfection. Failure to use proper components can lead to subpar outcomes.
Step-by-Step Neon Transfection Protocol
The Step-by-Step Neon Transfection Protocol is critical in achieving successful gene delivery in various biological applications. This technique provides a systematic approach that focuses on precision and repeatability, enhancing the reliability of results. Detailed attention to each phase of the protocol promotes transfection efficiency and ensures optimal cell performance. The benefits extend from improved gene transfer rates to maintaining cell viability, which are paramount for downstream applications.
Cell Preparation
Cell preparation is the first and pivotal stage of the neon transfection protocol. The aim is to handle the cells gently yet effectively to ensure they are in optimal condition for transfection. The type of cells used will dictate specific responsive behavior, so it is important to adhere to the following guidelines:
- Cell Density: Aim for a density of 70-90% confluency to foster optimal transfection rates. High-density cultures can compete for nutrients and space, potentially reducing overall productivity.
- Cell Type Considerations: Different cell types have varying sensitivities to transfection. While adherent cells might require detaching before transfection, suspension cells can often be directly transfected without this step. Assess the specific requirements for the cell line in use.
- Media Selection: Use complete media that supports cell growth. Avoid media containing antibiotics or supplements that might interfere with the transfection process. Freshly prepared media ensures cells are active and healthy.
- Incubation Conditions: Maintain appropriate temperature and CO₂ levels throughout the preparation process. Cells should be incubated under optimal environmental conditions in a CO₂ incubator.
Ensuring cell health at this stage is essential for successful molecular experimentation.
Electroporation Settings
Electroporation settings are vital in determining the efficiency of the transfection process. The parameters set during electroporation directly influence the cellular uptake of the genetic material. Adjustments should be made according to the following considerations:
- Voltage Settings: The voltage must be optimized based on cell type. Typically, voltages between 1,000 to 2,000 volts are employed.
- Pulse Duration: The duration of the electric pulse is crucial. It typically ranges from 20 to 50 milliseconds, with adjustments made based on the observed efficacy in preliminary trials.
- Number of Pulses: Employing a single pulse usually suffices, but multiple pulses (up to three) can improve uptake in some cases. Use caution to avoid causing excessive cell death.
- Buffer Conditions: The continuity of the electroporation buffer may affect cell compatibility. Use buffers that facilitate optimal conductivity and osmolarity.
It’s critical to document all settings throughout the experimentation phase, allowing for reproducibility in future experiments.
Post-Transfection Recovery
Post-transfection recovery is equally important. After the electroporation process, cells should not be subjected to immediate stress or harsh environments. This phase revolves around facilitating cell recovery and maximizing outcomes:
- Recovery Medium: Transfer cells to a suitable recovery medium post-transfection. This medium should support cell repair mechanisms while minimizing stress.
- Incubation Period: Allow cells to recover generally for 24 to 48 hours post-transfection, depending on the cell line. This time frame allows cells to express the transfected genes efficiently.
- Monitoring Cell Viability: Regularly assess cell viability via trypan blue exclusion or other relevant assays to determine the effect of transfection on cell health.
- Analyzing Results: Consider running preliminary assays post-recovery to gauge the transfection success rate. This can include methods like quantitative PCR or fluorescence microscopy to visualize expression.
"Attention to detail in each phase is key to successful gene delivery in transfection procedures."
Ensuring thoroughness in cell preparation, appropriate electroporation settings, and a careful recovery process significantly enhances the utility of this powerful tool in research and therapeutics.
Optimizing Transfection Efficiency
Optimizing transfection efficiency is crucial for successful molecular biology experiments, particularly those involving gene delivery. High transfection efficiency leads to a greater number of cells expressing the target gene, which can significantly impact experimental outcomes. This section will delve into specific strategies and considerations that can enhance the success rate of neon transfection protocols.
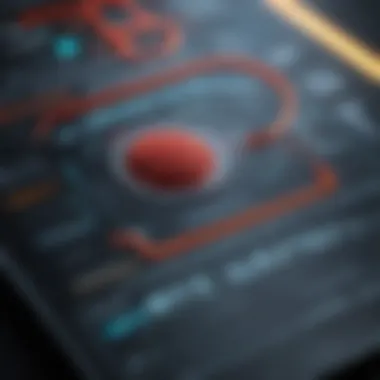
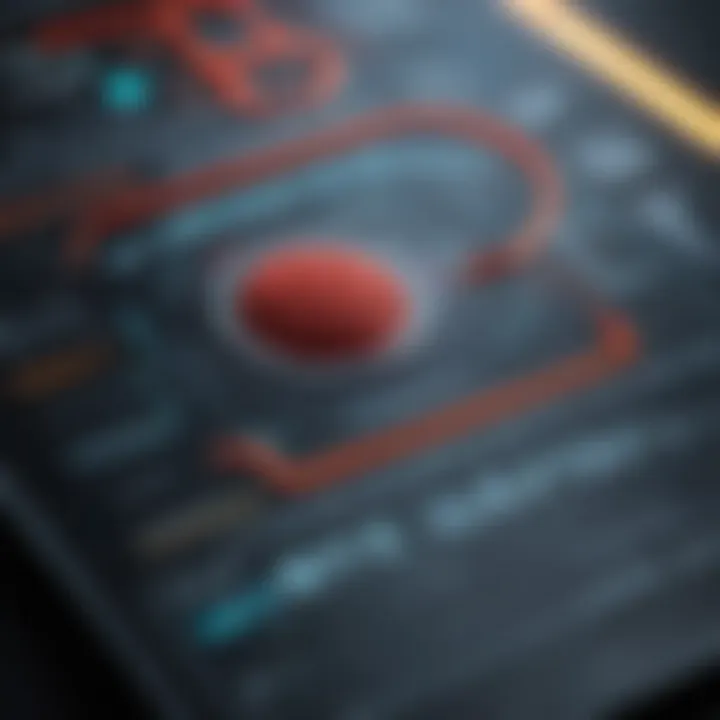
Parametric Adjustments
Parametric adjustments are key in fine-tuning the transfection process. The following aspects are important:
Voltage Settings
Voltage settings during neon transfection play a significant role in ensuring that the DNA enters the target cells effectively. The voltage has to be optimized based on the type of cells used and their membrane characteristics. One primary characteristic of voltage settings is their direct influence on the pore formation in the cell membrane, which is crucial for allowing DNA to enter. Higher voltages often result in increased transfection rates; however, they may also lead to higher cell death rates if not carefully managed. This is a beneficial choice in protocols where high transfection rates are needed but must balance cell viability.
A unique feature of voltage settings in neon transfection is the ability to adjust them according to cell type. For instance, some cells may require lower voltages to avoid toxicity, while others can withstand higher levels. The advantage is that researchers can tailor their approach, optimizing transfection further based on preliminary trials. However, the disadvantage is that it often requires extensive optimization, which can be time-consuming.
Pulse Duration
Pulse duration is another critical factor that affects the efficiency of the transfection process. This parameter determines how long the electric field is applied to the cells. Longer pulse durations can increase the likelihood of DNA entry; however, they also increase the risk of cell damage. Choosing the right pulse duration can help balance transfection efficiency and cell viability.
A notable aspect of pulse duration is its adjustment based on cell type and the overall experimental setup. This flexibility makes pulse duration a popular choice for optimizing transfection efficiency. A unique feature lies in the ability to apply multiple short pulses, which can improve DNA uptake while reducing cell damage compared to a single longer pulse. The advantage of this approach is that it may yield a higher percentage of viable, transfected cells, but the challenge is finding the ideal combination of pulse number and length that works best for specific experimental conditions.
Cell Density Considerations
Cell density is an important factor in optimizing transfection efficiency. It influences how many cells can be transfected simultaneously and the effectiveness of the transfection process. Too high or too low a density can negatively impact transfection efficiency. Finding the ideal cell density can ensure that cells are in the exponential growth phase and receptive to transfection.
DNA Amount Optimization
The amount of DNA used during the transfection process can greatly impact efficiency. Using too little DNA may lead to low transfection rates, while excessive DNA can be toxic to cells. Therefore, optimizing DNA amount is essential for successful transfection. Researchers often perform a series of tests to identify the optimal quantity that results in effective transfection without compromising cell viability.
Understanding these adjustments provides a roadmap for improving transfection outcomes in experimental setups. By optimizing voltage settings, pulse duration, cell density, and DNA amount, researchers can significantly enhance the effectiveness of neon transfection.
Assessing Transfection Outcomes
Assessing the outcomes of transfection is crucial in understanding the efficiency and success of the neon transfection protocol. This assessment not only informs researchers about the uptake of foreign DNA but also guides subsequent experimental designs. The significance of this assessment stretches beyond mere data collection; it aids in optimizing protocols, troubleshooting issues, and validating experimental goals. An effective evaluation of transfection outcomes ultimately contributes to the reliability and reproducibility of the research findings.
Quantitative Methods
Flow Cytometry
Flow cytometry is a powerful tool that plays a key role in the quantification of transfected cells. It allows for rapid analysis of thousands of cells, providing data on the percentage of cells expressing the transgene. A main characteristic of flow cytometry is its ability to distinguish live, dead, and transfected cells through fluorescent markers. This feature makes flow cytometry a popular choice for researchers wanting precise and quantifiable results. It offers advantages such as high throughput and the capability to analyze multiple parameters simultaneously.
However, flow cytometry does have some disadvantages. The need for specialized equipment and training can hinder its accessibility for some laboratories. Additionally, the accuracy of results can be influenced by the quality of the fluorescent markers used. Despite these challenges, its unique capacity to provide quantitative data makes flow cytometry a critical method in assessing transfection outcomes.
Quantitative PCR
Quantitative PCR (qPCR) is another method utilized to measure gene expression levels post-transfection. Its primary strength lies in its sensitivity and specificity for detecting specific sequences of DNA, which allows for precise quantification of transfected DNA. This method is beneficial in confirming that the transfection has been successful at the molecular level. One unique feature of qPCR is its ability to monitor amplification of DNA in real-time, providing immediate feedback about the transfection efficiency.
Nevertheless, qPCR does have some limitations. It requires well-designed primers and control samples to ensure accurate quantification. Also, the process can be labor-intensive and may need more detailed analysis to interpret results accurately. However, the advantages of sensitivity and real-time monitoring often outweigh the disadvantages, making qPCR an invaluable tool in assessing transfection outcomes.
Qualitative Methods
Fluorescence Microscopy
Fluorescence microscopy allows researchers to visualize transfected cells and confirm the presence of the transgene. This method is notable for its ability to provide qualitative insights. By using fluorescent tags, specific proteins or nucleic acids within the cells can be illuminated, revealing the spatial distribution of transgene expression. This visual aspect of fluorescence microscopy makes it a popular choice in studies where the localization of the gene product is important.
The primary advantage of this method is its capability to offer direct visual confirmation of transfection. However, a notable limitation is that fluorescence microscopy typically requires a higher concentration of transfected material to achieve clear results. This demand may not always be achievable in all experimental setups, especially with low transfection efficiencies.
Western Blot Analysis
Western blot analysis serves as a method to detect specific proteins that result from successful transfection. This technique involves protein separation by gel electrophoresis followed by transfer to a membrane and detection using specific antibodies. A key characteristic of Western blot analysis is its ability to provide information about the size and expression levels of the targeted protein, making it a crucial method for verifying successful transgene expression.
Despite its strengths, Western blot analysis also presents challenges. It is generally time-consuming and requires a certain level of expertise for accurate interpretation. Additionally, the success of this method relies heavily on the quality of antibodies used for detection. Nonetheless, its robust ability to confirm the expression of the transgene at the protein level makes it an essential tool in assessing transfection outcomes.
Understanding the outcomes of transfection is not merely about gauging success; it is also a key element of refining the techniques applied in molecular biology.
Troubleshooting Common Issues
Troubleshooting in the context of neon transfection is critical for ensuring that experiments yield reliable and reproducible results. Even experienced researchers may encounter challenges during transfection, and understanding how to address these issues optimally can significantly influence the outcome of scientific studies. The effectiveness of gene delivery often hinges on timely problem-solving, making it essential to recognize and rectify common pitfalls. This section delves into prevalent issues faced during neon transfection, offering insight into their resolution to reinforce transfection success.
Low Transfection Efficiency
Low transfection efficiency is a frequent issue encountered during the neon transfection process. Achieving optimal efficiency is crucial, as it directly impacts the success of any downstream applications such as gene editing or protein expression. There are several factors that may contribute to low efficiency, including suboptimal electroporation settings, inappropriate DNA concentration, or poor cell condition prior to transfection.
To improve transfection efficiency:
- Optimize Electroporation Settings: Adjust voltage and pulse duration according to the specific cell type used. Certain cell lines may require different settings for optimal performance.
- Review DNA Quality: Ensure that the DNA is of high purity and concentration. Contaminated or degraded DNA can lead to reduced transfection rates.
- Assess Cell Density: Transfecting cells at the right density is important. Too few cells can reduce the efficiency of the process, while too many can lead to cell stress.

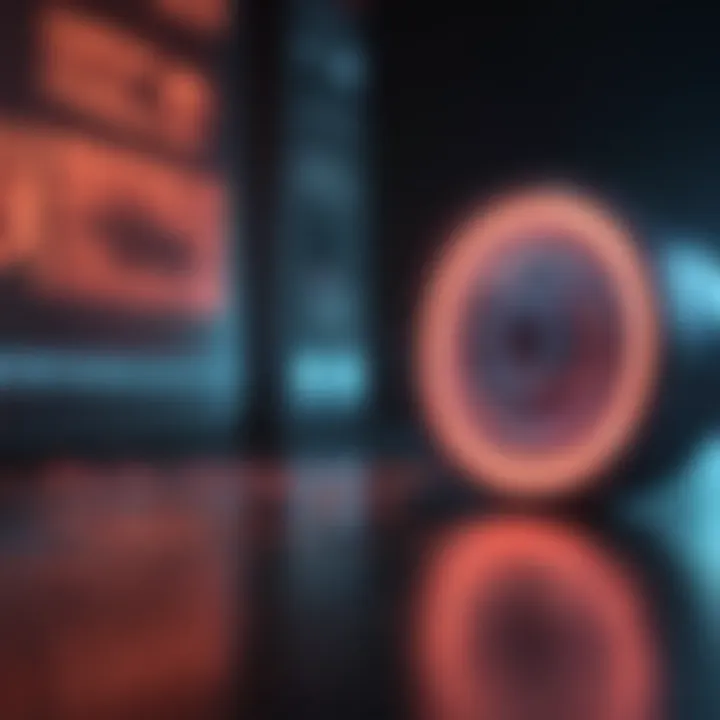
By systematically evaluating these factors, researchers can enhance transfection outcomes.
Cell Viability Concerns
Cell viability is an essential aspect to monitor following neon transfection. While the primary goal is gene delivery, preserving cell health is equally important for obtaining reliable results in subsequent experiments. High voltage settings or prolonged pulse durations may lead to increased cell mortality rates, often making it difficult to interpret results accurately. Therefore, maintaining a balance between achieving effective transfection and preserving cell viability is crucial.
To address cell viability issues:
- Modify Pulse Parameters: Integrate gentler electroporation conditions, particularly when working with sensitive cell types. This adjustment can help to minimize cellular stress.
- Use Recovery Medium: After transfection, cells should be placed in a recovery medium that promotes healing and regrowth. This medium is often devoid of antibiotics which can further stress cells.
- Regular Monitoring: Observe cells for several hours post-transfection to assess cell health. Any signs of significant cell death should prompt a review of transfection parameters.
These strategies aid in maintaining cell health, facilitating subsequent analysis.
Contamination Risks
Contamination can pose significant threats to the success of any experimental protocol, and neon transfection is no exception. Introducing foreign organisms or substances during the process can invalidate results and skew data interpretation. Contamination can occur at multiple stages, including during cell culture preparation, DNA handling, or even the electroporation step.
To mitigate contamination risks:
- Maintain Sterility: Utilize sterile techniques throughout the process. Regular use of disinfectants on surfaces and equipment is essential to reduce microbial presence.
- Careful DNA Handling: Use high-quality, uncontaminated reagents when preparing DNA. Additionally, proper storage conditions should be maintained.
- Check Environmental Conditions: Ensure that the laboratory environment, including incubators and cold storage, is routinely monitored for contamination and adjusted as necessary.
Proactively addressing these aspects can substantially decrease contamination chances, ensuring the reliability of transfection results.
Key Points to Remember:
- Low transfection efficiency can arise from multiple factors, requiring thorough investigation.
- Maintaining cell viability is paramount for obtaining valid experimental outcomes.
- Contamination, if neglected, can jeopardize experimental findings.
The significance of troubleshooting common issues cannot be overstated. Addressing these challenges adequately allows researchers to optimize their experiments, ensuring that they can leverage the full potential of neon transfection protocols.
Applications of Neon Transfection
Neon transfection is a highly versatile technique that plays a crucial role in various fields of molecular biology. Its applications range from basic research to advanced therapeutic strategies. Understanding where and how this method is applied allows researchers to harness its power effectively. Below, we explore some prominent applications of neon transfection in greater detail.
Gene Editing and Knockouts
Gene editing has become a core area in molecular biology, and neon transfection greatly facilitates this process. It enables the introduction of plasmid DNA or RNA into cellular models, making it possible for scientists to modify genes effectively. With tools like CRISPR-Cas9, researchers can create precise gene knockouts, allowing the investigation of gene functions or disease models.
One significant benefit is the efficiency and reliability that the neon transfection protocol provides. By optimizing cellular conditions and using the right voltage settings, researchers can achieve high transfection rates. This results in a higher percentage of successfully edited cells, which is of utmost importance in genetic studies.
Moreover, neon transfection allows for the simultaneous editing of multiple genes, proving invaluable in complex genetic studies. It has also been used in therapeutic strategies aimed at correcting genetic disorders, presenting a promising avenue for future clinical applications.
Protein Expression Studies
Neon transfection is pivotal for protein expression studies. By delivering DNA that encodes a specific protein into cells, researchers can analyze the effects and functions of that protein in real time. This process is essential for understanding the complexity of protein interactions and cellular responses.
The accuracy of the protocol allows for controlled expression levels, which is key for reproducibility in experiments. Scientists can create stable cell lines that express a particular protein, enabling longitudinal studies and therapeutic applications. Recognizing the structural nuances of the expressed protein can also lead to insights into its role in various biological pathways.
In addition, the technique aids in the production of recombinant proteins, which can be used in drug development and vaccine production. By establishing optimal conditions, researchers can scale up production, making this application crucial for both academic and industrial endeavors.
Drug Development Research
The role of neon transfection in drug development research cannot be overstated. It allows for rapid assessment of the effects of potential therapeutics at the cellular level. By transfecting cells with constructs related to drug targets, researchers can evaluate how these drugs modulate specific pathways.
This method accelerates the preclinical testing phase, allowing scientists to elucidate interactions between new drugs and their biological targets. It also enables the identification of biomarkers associated with drug responses, contributing to personalized medicine approaches.
As researchers work towards developing more effective therapeutics, neon transfection offers a robust platform for screening and validation of drug candidates, streamlining the pathway from discovery to clinical application.
Noteworthy: The applications of neon transfection extend beyond these examples, influencing various domains such as vaccine research and regenerative medicine.
Future Directions in Transfection Technology
The landscape of molecular biology is ever-evolving, particularly in the domain of gene delivery methods. Future directions in transfection technology hold critical implications for both research and therapeutic applications. As scientists explore innovative solutions, advancements in transfection techniques can streamline experiments, enhance efficiency, and broaden the scope of molecular diagnostics and treatments. This exploration emphasizes the need for continuous improvement and adaptation in protocols to meet diverse experimental demands.
Emerging Techniques
Recent advancements in transfection technology suggest several promising directions. Some notable emerging techniques include:
- Nanoparticle-based Delivery: Utilization of nanoparticles can improve the specificity and efficiency of gene delivery. Their small size allows for better cellular uptake and protection of nucleic acids from degradation.
- CRISPR-Cas Systems: While primarily known for gene editing, CRISPR technology also influences transfection. By refining delivery methods, researchers can achieve precise alterations in gene expression with minimal off-target effects.
- Targeted Delivery Systems: Development of ligands that direct delivery systems to specific cell types is an area of growing interest. This specificity minimizes unintended consequences and enhances therapeutic efficacy.
These emerging methods reflect a trend towards more sophisticated, less invasive, and more targeted transfection approaches.
Ethical Considerations
As the capabilities of transfection technologies expand, ethical considerations become increasingly salient. Key issues to address include:
- Biosafety and Regulations: New techniques must comply with evolving biosafety standards. Regulatory frameworks must adapt to the introduction of novel technologies to ensure public safety and responsible research practices.
- Potential for Gene Editing Misuse: As technologies like CRISPR become accessible, there is a need for guidelines to prevent misuse. The implications of gene editing on future generations and ecosystems raise significant ethical questions.
- Informed Consent: For clinical applications of gene delivery methods, obtaining informed consent from patients is crucial. This entails transparent communication regarding potential risks and benefits associated with novel therapies.
"The power of transfection technology is immense, but with great power comes great responsibility. Ethical considerations must guide the development and application of these techniques."