Next Generation Sequencing Platforms: An In-Depth Analysis
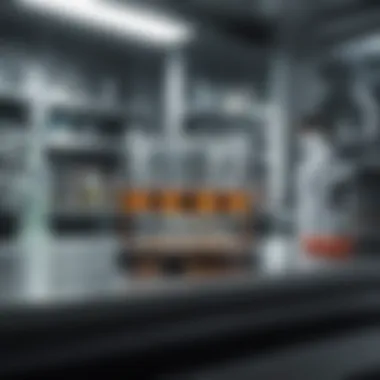
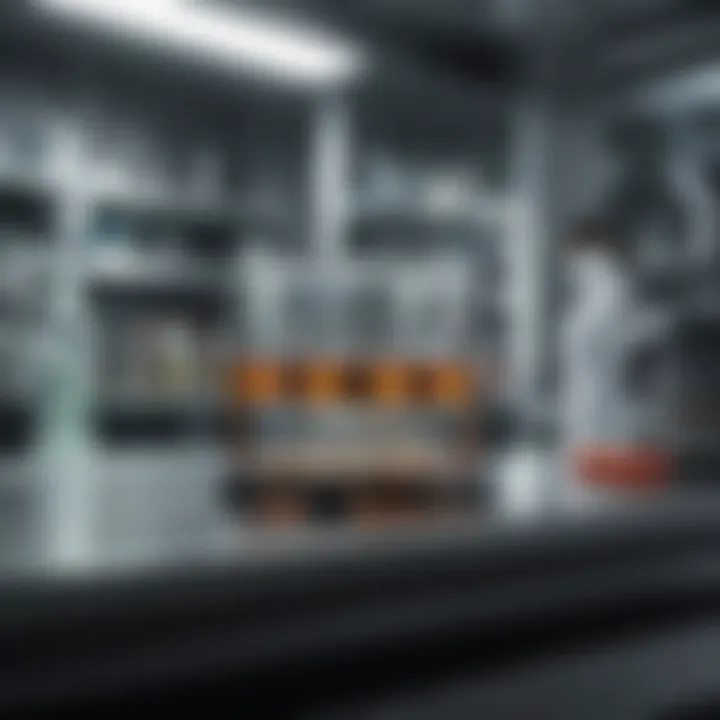
Intro
Next-generation sequencing (NGS) platforms have fundamentally changed the landscape of genomic research and clinical practices. These technologies allow scientists to sequence DNA and RNA much more quickly and cost-effectively than traditional methods. The advancements in NGS have led to far-reaching implications in various fields including molecular biology, medicine, and evolutionary studies.
As we dive deeper into the intricacies of NGS, it becomes important to understand its mechanisms, the innovations driving its growth, and the challenges it presents. This article will dissect various NGS platforms, providing a thorough analysis that caters to a wide array of readers, from novice students to seasoned professionals.
Research Overview
The exploration of NGS technologies follows specific methodological approaches that allow for empirical data collection and analysis. It is essential to grasp how these methods evolve with technological advancements.
Methodological Approaches
Different NGS platforms utilize distinct methodologies. The main approaches include:
- Sequencing by synthesis (SBS) used by Illumina platforms, which involves sequencing complementary DNA strands through similar synthesis processes.
- Ion semiconductor sequencing, implemented by Ion Torrent, which measures the release of hydrogen ions during the DNA polymerization process.
- Single-molecule real-time (SMRT) sequencing by Pacific Biosciences, which allows for the observation of single DNA molecules in real time and can produce longer read lengths.
These methodologies differ primarily in their sensitivity, accuracy, and throughput. NGS platforms collectively allow for multi-gene sequencing, targeted panels, and whole-genome sequencing, catering to a variety of research and clinical needs.
Significance and Implications
The implications of NGS technologies extend beyond sequencing alone. They foster applications in personalized medicine, genetic diagnostics, and infectious disease management, among other areas. The ability to analyze a vast amount of genomic data presents researchers with opportunities to discover genetic variations and understand their roles in disease pathogenesis.
Moreover, NGS plays a pivotal role in epidemiology, particularly in tracking outbreaks and understanding pathogen evolution. With ongoing developments, the relevance of NGS in research and clinical settings grows ever stronger.
"Next-generation sequencing platforms have enabled the analysis of millions of genetic variations across diverse populations, leading to enhanced insights into human health and disease."
Current Trends in Science
The scientific community continually strives for improvement and innovation. Current trends indicate a shift toward integration in genomic research and clinical applications.
Innovative Techniques and Tools
Recent advancements in bioinformatics and data management tools have enhanced the utility of NGS data, allowing researchers to cope with the extensive volumes of information generated. Techniques such as machine learning and artificial intelligence are now being applied to improve data interpretation and accelerate the identification of significant genomic variants.
Moreover, new tools that enable more efficient sample preparation and sequencing logistics are emerging. These innovations promise to further reduce costs and processing times, making NGS even more accessible.
Interdisciplinary Connections
Interdisciplinary collaboration has become crucial for the progression of NGS technologies. Fields such as computer science, statistics, and molecular biology are merging to enhance the effectiveness of genomic research. This collaboration enhances data analytics capabilities, ultimately leading to advancements in precision medicine and therapeutic strategies.
As the potential of NGS continues to unfold, it remains imperative to remain vigilant about its challenges, including ethical issues related to genomic data privacy, interpretation complexities, and the need for robust data management frameworks.
Prologue to Next Generation Sequencing
Next Generation Sequencing (NGS) has fundamentally changed the landscape of genomics, offering unprecedented capabilities for the analysis of genetic material. Its advent represents a major leap in our ability to decipher DNA and understand the complexities underlying genetic information.
NGS technologies provide researchers with the tools to sequence entire genomes quickly and at a fraction of the cost of traditional methods. They empower a wide range of applications, from basic research in genomics to clinical diagnostics, making them indispensable in today’s scientific arsenal. Understanding NGS is crucial for both researchers and practitioners who strive to make advancements in healthcare, genetics, and biological sciences.
Defining Next Generation Sequencing
Next Generation Sequencing refers to a collection of advanced sequencing technologies that allow for massive parallel sequencing of DNA. Unlike traditional Sanger sequencing, which sequences one DNA fragment at a time, NGS can sequence millions of fragments simultaneously. This technology works by generating millions of short DNA sequences, which are then assembled and analyzed using sophisticated bioinformatics tools.
NGS can target specific regions of interest or cover entire genomes, providing comprehensive insights into genetic variations and mutations.
Historical Context and Development
The journey towards NGS began with the Human Genome Project, which was completed in 2003 using traditional sequencing methods. As the demand for faster and cheaper sequencing grew, technological innovations emerged. The first commercially available NGS platform was launched in 2006. Over the years, significant improvements in read length, accuracy, and throughput have propelled NGS into the forefront of genomic research.
Advantages Over Classical Sequencing Methods
NGS offers several advantages over classical sequencing techniques:
- Speed: NGS can generate vast amounts of data in a fraction of the time, allowing for rapid results.
- Cost-Effectiveness: The cost per base sequenced has dramatically decreased, making genome sequencing accessible to more researchers and healthcare providers.
- Throughput: Its ability to sequence millions of fragments at once enables comprehensive analyses that classical methods cannot achieve.
- Versatility: NGS can be used for a wide array of applications, such as whole genome sequencing, targeted resequencing, and transcriptome analysis.
Understanding these advantages is essential for harnessing the full potential of NGS in various fields, including personalized medicine and genetic research.
Types of Next Generation Sequencing Platforms
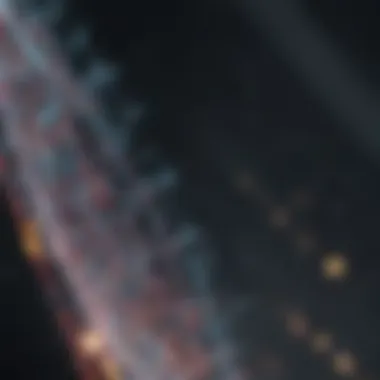
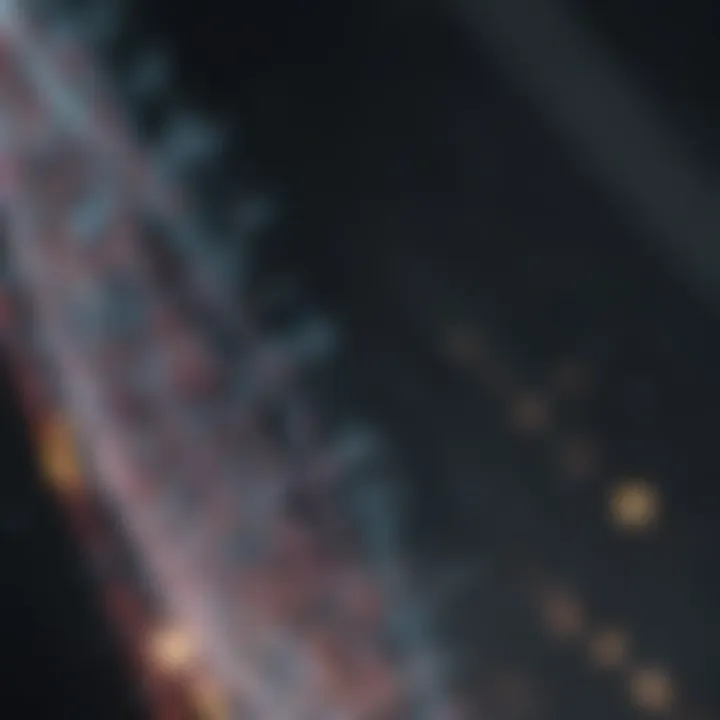
Understanding the types of next generation sequencing platforms is essential for researchers and professionals in the field of genomics. These platforms vary in their methodology, throughput, accuracy, and cost, which all play a critical role in selecting the right technology for a specific application. Their diversity also reflects the rapid advancements in sequencing technology, pushing the boundaries of what is possible in genomic research and clinical diagnostics.
Different types of NGS platforms serve unique purposes, whether it’s for large-scale genomic projects or smaller, focused studies. Each platform has distinct advantages that must be considered in the context of the research questions at hand. This section will provide an insightful analysis of five prominent types of NGS platforms, each with a unique approach to genomic sequencing.
Sequencing by Synthesis
Sequencing by synthesis is one of the most widely used NGS methods. This technique generates DNA sequences by determining the order of nucleotides in a DNA strand. It works by adding labeled nucleotides one at a time to a growing DNA strand. Each base incorporated releases a distinct fluorescent signal, allowing for real-time tracking of the sequence. The advantages of sequencing by synthesis include high accuracy and the ability to produce millions of sequences simultaneously. Platforms like Illumina are major players in this space due to their efficiency and reliability.
Sequencing by Ligation
In sequencing by ligation, the process hinges on the use of hybridization and ligation steps. Primers labeled with fluorescent dyes are ligated to the DNA template. This method can generate long reads and is adept at accurately determining sequences with complex regions. The main advantage of sequencing by ligation is its flexibility and potential for high throughput. The SOLiD technology is a notable example that operates on this principle, providing an alternative to traditional sequencing strategies.
Ion Torrent Technology
Ion Torrent technology leverages a different approach than traditional sequencing methods. It uses semiconductors to detect changes in pH that occur when nucleotides are incorporated into a DNA strand. This allows for rapid sequencing without the need for optical reading. The main benefits of Ion Torrent include cost-effectiveness and a compact design, making it suitable for smaller labs. Although it may not achieve the same accuracy as sequencing by synthesis, it offers fast turnaround times, which can be critical in time-sensitive research.
Single-Molecule Real-Time Sequencing
Single-molecule real-time sequencing (SMRT) stands out by directly observing the synthesis of DNA. This method allows for much longer read lengths compared to conventional methods. By capturing all four nucleotides in real time, the technique emphasizes clarity in distinguishing between similar sequences. The PacBio platform is a leading example of this technology. Its ability to reveal structural variations and epigenetic modifications sets it apart, making it valuable for comprehensiveness in genomic studies.
Other Emerging Technologies
The landscape of next generation sequencing is continuously evolving with the advent of new technologies. Companies are exploring various innovative approaches, including nanopore sequencing and CRISPR-based methods. Nanopore sequencing allows DNA strands to pass through a nanoscale protein pore. This can yield rapid sequencing results and is advantageous for portable applications. CRISPR-based methods aim at targeted sequencing, enabling specific regions of DNA to be analyzed with high precision. These emerging technologies promise to enhance sequencing capabilities and address specific research needs.
In summary, each type of next generation sequencing platform holds its own advantages and limitations. Decision-making regarding which platform to use is driven by factors such as accuracy requirements, read lengths, project scale, and budget considerations. Understanding these various platforms will better equip researchers and clinicians to make informed choices that align with their sequencing goals.
Operational Mechanisms of NGS
Understanding the operational mechanisms of next-generation sequencing (NGS) is crucial in appreciating its transformative impact on genomics. This segment discusses the essential processes that make NGS effective and efficient. The focus lies on three core areas: library preparation, amplification techniques, and sequencing processes. Together, these elements work in a synchronized manner to generate high-quality sequencing data, which can then be analyzed for various research and clinical applications.
Library Preparation
Library preparation is the initial step in NGS. It involves the creation of a sequencing library from the target DNA or RNA sample. The quality of this library significantly influences the accuracy and reliability of the sequencing results. During this step, nucleic acids are fragmented to manageable sizes and are then ligated with specific adapters. These adapters serve multiple purposes: they facilitate the binding of the library to the sequencing flow cell, allow for the identification of the fragments later, and enable the amplification during the sequencing process.
Efficient library preparation is vital for maximizing sequencing output. If the library is not prepared correctly, it can lead to poor coverage and increased systematic errors. Consequently, researchers often utilize various kits and methods tailored for specific applications to ensure optimal results, such as those offered by Illumina or Thermo Fisher Scientific.
Amplification Techniques
Following library preparation, amplification techniques are employed to generate sufficient quantities of sequences for accurate detection. There are several methods available, with polymerase chain reaction (PCR) being one of the most common. PCR amplifies the DNA fragments in a targeted manner, resulting in millions of copies of the desired sequences.
The importance of amplification lies in its ability to enhance the sensitivity of the sequencing process. Low-abundance sequences can be effectively increased to levels that are detectable by the sequencer. However, it is crucial to minimize bias introduced during this phase. Over-amplification or biased amplification could distort the relative abundance of certain sequences, which may lead to interpretational errors. Assessing the amplification efficiency is thus essential to maintain the fidelity of results.
Sequencing Processes
The actual sequencing processes are where the critical operations of NGS take place. Different platforms employ varying methodologies, including sequencing by synthesis, ligation, and other technologies. Generally, the sequencer reads the DNA fragments by either emitting a fluorescent signal or detecting specific chemical reactions as nucleotides are incorporated into the growing DNA strand.
What sets NGS apart from traditional sequencing is its ability to generate millions of sequences simultaneously, known as massively parallel sequencing. This greatly reduces the time and cost associated with sequencing compared to the earlier methods. However, it is important to note that the data generated are vast and require robust bioinformatics tools for analysis.
The transformative nature of NGS lies not only in its speed but also in its capacity to elucidate complex biological questions by providing unprecedented amounts of data.
The operational mechanisms of NGS—from library preparation, amplification to sequencing—form the backbone of its capabilities. A clear understanding of these processes highlights their importance, challenges, and implications for the field of genomics.
Applications of Next Generation Sequencing
Next Generation Sequencing (NGS) has revolutionized our understanding of genomics and molecular biology. Its applications are vast and varied, making it a critical tool for researchers, clinicians, and educators. The ability to sequence large volumes of DNA quickly and cost-effectively opens up a multitude of avenues for exploration and development in various fields.
One of the major benefits of NGS applications is its precision. The technology allows for detailed genetic analysis, leading to discoveries that were previously unattainable through traditional methods. The impact of NGS on advancing knowledge in genomics, transcriptomics, and clinical research cannot be overstated. This section explores several key applications, highlighting their significance and contributions to the field.
Genomic Research
Genomic research has greatly benefited from NGS technologies. The ability to sequence entire genomes at a low cost enables researchers to conduct large-scale studies. This has significant implications for evolutionary biology, genetics, and population studies.
- Discovery of Genetic Variants: NGS aids in identifying single nucleotide polymorphisms (SNPs) and structural variants within populations.
- Comparative Genomics: Researchers can compare genomes across species, providing insights into evolutionary relationships.
- Functional Genomics: Understanding gene function and regulation has been enhanced, giving researchers tools to investigate gene-environment interactions.
The depth of information available through NGS allows for powerful analyses that advance both basic and applied research.
Transcriptomics
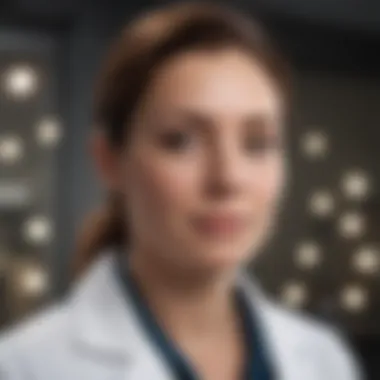
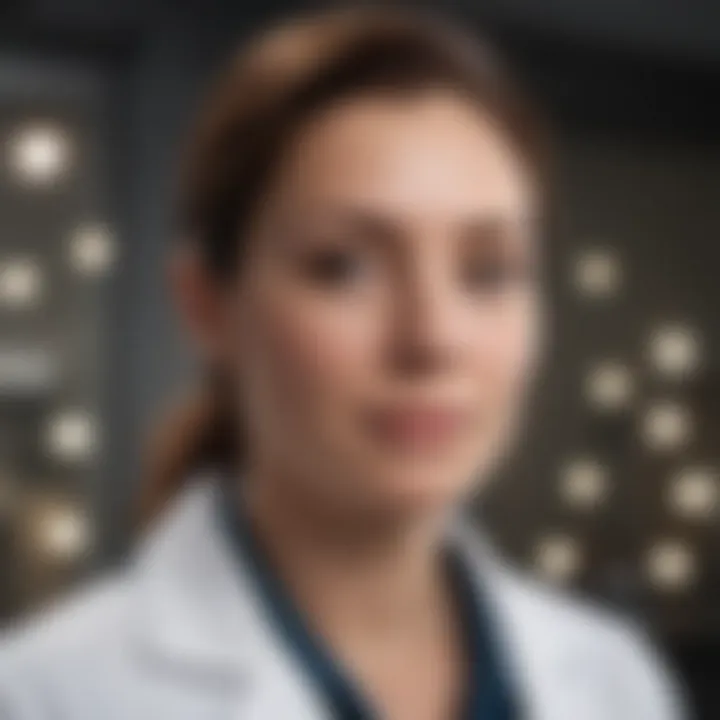
Transcriptomics focuses on the complete set of RNA transcripts produced by the genome. NGS techniques, such as RNA-Seq, have transformed this field by providing a comprehensive approach to understanding gene expression.
- Gene Expression Profiling: NGS enables the quantification of gene expression levels across different conditions or treatments.
- Alternative Splicing Analysis: Researchers can investigate the different isoforms of mRNA generated from a single gene, leading to insights into post-transcriptional regulation.
- Identification of Non-Coding RNAs: The discovery of various non-coding RNAs has been facilitated by NGS, revealing their roles in gene regulation and other cellular processes.
The insights gained from transcriptomic studies are essential for understanding cellular function and the underlying mechanisms of diseases.
Metagenomics
Metagenomics expands the analysis of genetic material from environmental samples, encompassing the diverse microbial communities present. NGS technologies have greatly improved the efficiency of metagenomic studies. It allows for the direct analysis of DNA from environments without needing to culture organisms.
- Microbiome Research: NGS has become the backbone of microbiome studies, enabling the exploration of microbial diversity and function in various ecosystems, including human health.
- Bioremediation: Understanding microbial communities involved in bioremediation processes has been facilitated by NGS, informing strategies for environmental cleanup.
- Pathogen Detection: Rapid identification of pathogens in clinical settings can be achieved through metagenomic approaches, improving outbreak response times.
Metagenomics shines in its ability to uncover the hidden biodiversity that traditional approaches may overlook.
Clinical Applications
Clinical applications of NGS are among the most promising areas of development. The precision and speed of NGS technologies transform diagnostics, treatment planning, and personalized medicine practices.
- Oncology: NGS helps identify mutations in cancer patients, allowing for targeted therapies tailored to individual tumors.
- Genetic Disorders: NGS can diagnose hereditary diseases by pinpointing pathogenic variants, facilitating timely interventions.
- Infectious Diseases: Pathogen sequencing can lead to quick identification of infectious agents, enhancing treatment effectiveness.
The integration of NGS into clinical workflows is beginning to redefine standards of care and improve patient outcomes. NGS is paving the way for precision medicine, which tailors treatment to the unique molecular profile of each patient.
The applications of next-generation sequencing demonstrate its transformative impact on science and medicine. The ability to decode the vast information held within genomes and transcripts enhances our understanding of biological systems.
Data Management and Analysis
Data management and analysis are essential components of next-generation sequencing (NGS). As the volume of data generated by NGS increases exponentially, effective strategies for data handling become crucial. Researchers must navigate not only the sheer scale of data but also the complexity of its interpretation. This section explores the main aspects of data management and analysis, highlighting challenges, tools, and the importance of accurate interpretation.
Data Generation Challenges
Generating data through NGS brings various challenges. One significant issue is the sheer volume of data produced during sequencing. Thousands to millions of sequences can be obtained in a single run, leading to difficulties in storage, processing, and analysis. Additionally, sequencing errors can occur, where incorrect bases are read, impacting data quality. Thus, managing this influx and ensuring its integrity is vital.
Moreover, differences in sequencing technologies can create discrepancies in data formats and quality metrics. This variability complicates comparisons across studies or platforms, requiring standardization efforts. Researchers must be equipped to address these challenges to utilize NGS efficiently.
Bioinformatics Tools for NGS
Several bioinformatics tools exist to assist in NGS data management and analysis. These tools help streamline the workflow from data acquisition to interpretation, facilitating the handling of large datasets. Some notable tools include:
- Bowtie: It effectively aligns sequencing reads to lengthy reference genomes, optimizing speed and accuracy.
- GATK (Genome Analysis Toolkit): A versatile toolkit used for variant discovery and genotyping. It includes tools for pre-processing, calling variants, and filtering.
- HISAT2: A fast and sensitive alignment program, suitable for mapping RNA-seq data.
- Galaxy: An open-source platform that enables users to access diverse bioinformatics tools via a web interface, promoting accessibility.
These tools are instrumental in managing the various stages of NGS data analysis, from raw data extraction to precise alignment and variant identification.
Interpreting NGS Data
Interpreting NGS data is a complex task requiring a deep understanding of both the biological context and computational methods. The interpretation involves identifying meaningful biological insights from raw data, such as identifying potential mutations associated with diseases or understanding gene expression patterns.
High-throughput sequencing results often require sophisticated statistical methods to discern true biological signals from noise. Machine learning algorithms can assist in predictive modeling and classification of genomic features, enhancing the interpretation process.
Aligning findings with existing databases, such as those maintained by the NIH or EMBL, is crucial for validating results. Ongoing cross-referencing facilitates knowledge building and the potential development of new hypotheses.
"Effective data analysis in NGS not only relies on robust computational tools but also on the ability to interpret findings within the broader context of existing scientific knowledge."
In summary, data management and analysis for NGS demands careful consideration of various challenges. Employing proper bioinformatics tools enhances workflows and aids interpretation. As the sector grows, the integration of sophisticated analytical approaches will become increasingly important to realize the full potential of NGS in modern research.
Ethical Considerations in NGS
Next Generation Sequencing (NGS) technology offers remarkable advancements in genomics, but with these advancements come significant ethical considerations. Understanding the ethical implications is essential for responsible use in research and clinical practice. This section addresses privacy and consent issues, along with data sharing concerns, both crucial for navigating the ethical landscape of NGS.
Privacy and Consent Issues
With the extensive data generated by NGS, protection of individual privacy is paramount. Sequencing can reveal sensitive information about genetic predispositions, health risks, and even information about relatives. The potential for misuse of this data raises urgent questions about privacy.
When individuals undergo genetic sequencing, informed consent must be obtained. This consent should be clear and comprehensive, ensuring that patients understand what their data entails, potential risks, and implications for future research. Clinical practices need to adopt transparent policies regarding how data is used, who has access, and for what purposes.
- Informed Consent
- Anonymization
- Patients must grasp all aspects, from the benefits to the limitations of the sequencing.
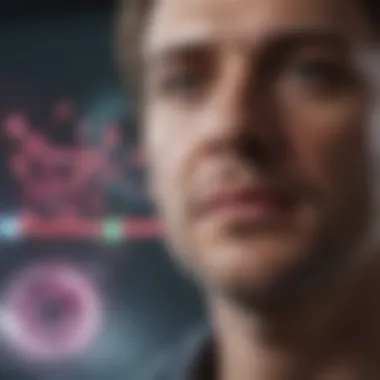
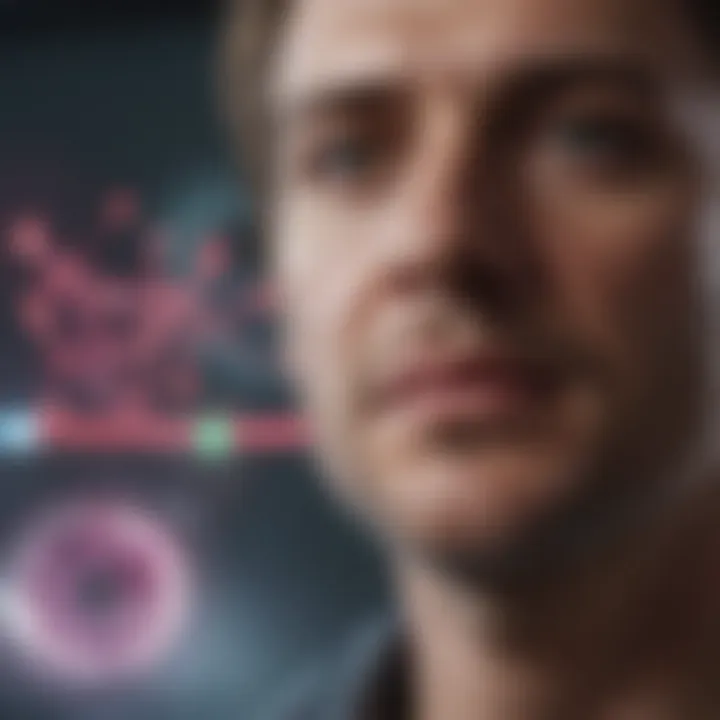
- Techniques must be employed to protect identities while maintaining the integrity of the data.
Organizations should champion ethical practices by adhering to guidelines and regulations that promote both transparency and respect for privacy.
Data Sharing Concerns
The field of genomics thrives on data sharing. Data generated through NGS can lead to breakthroughs in research and personalized medicine. However, sharing this data raises ethical dilemmas.
Researchers often face tension between the collaborative nature of science and the need for individual privacy. In many cases, sharing data can yield insights into rare diseases or population-specific studies. However, there is a risk that identifiable data might be exposed without consent.
Key considerations in data sharing include:
- Regulatory Compliance
- Limiting Access
- Community Engagement
- Following regulations such as the General Data Protection Regulation (GDPR) is vital for ethical data sharing.
- Careful vetting and restrictions on who can access the data will mitigate the risk of misuse.
- Engaging with the community can foster trust and understanding of how their data will be used.
Ultimately, a delicate balance is needed. Ethical frameworks and guidelines must evolve alongside NGS technology to ensure that data sharing benefits society while safeguarding individual rights.
"Ethics in genomic data management is not just a legal requirement; it's the responsibility of the scientific community to honor individual dignity and privacy."
This section illustrates that as NGS technologies expand, so too must our commitment to ethical practices that prioritize patient rights and the integrity of the data. Attention to privacy and data sharing concerns stands as a cornerstone in harnessing the full potential of NGS for future scientific advancements.
Future Directions for Next Generation Sequencing
The field of next-generation sequencing (NGS) is evolving rapidly. Emerging technologies and methodologies are being developed, leading to new horizons in research and clinical applications. Understanding these future directions is crucial for researchers, educators, and professionals who aim to stay at the forefront of genomics. This section will explore key elements that shape the future of NGS, its benefits, and considerations that must be taken into account.
Innovations on the Horizon
Several innovations promise to enhance the capabilities of NGS platforms. These advancements may lead to faster sequencing speeds and increased accuracy in results. Some noteworthy innovations include:
- Ultra-high-throughput sequencing: This method aims to significantly increase the volume of data generated in a single run, thus reducing the cost per base sequenced.
- Miniaturized sequencing devices: The emergence of portable sequencing technologies could enable in-field analysis, making it accessible in remote locations.
- Artificial intelligence integration: AI could play a significant role in data processing and interpretation, helping to identify patterns and anomalies that human analysis might overlook.
These innovations will not only improve efficiency but also broaden the scope of applications across various disciplines.
Impact on Personalized Medicine
The integration of NGS into personalized medicine represents a landmark shift in healthcare. By analyzing an individual's genetic information, medical professionals can tailor interventions based on specific genetic markers. Key impacts include:
- Targeted therapies: Treatment plans can be designed according to genetic profiles, making use of specific drugs that are most likely to be effective.
- Predictive analytics: NGS can identify predispositions to certain diseases, allowing for early prevention strategies to be implemented.
- Improved patient outcomes: Personalized approaches can streamline diagnosis and treatment, ultimately enhancing the effectiveness of medical care.
This transformation in healthcare highlights the necessity for ongoing research in NGS technologies to ensure accuracy and affordability.
Integration with Other Technologies
The future of NGS is likely to involve extensive integration with other technologies, enhancing its capabilities. Possible integrations include:
- Clinical informatics: Combining NGS with electronic health records (EHR) could pave the way for more informed decision-making and patient management.
- Wearable technology: Integrating genomic information with real-time health data from wearable devices could provide a more comprehensive view of an individual's health state.
- CRISPR and gene editing technologies: NGS can guide gene editing efforts by identifying specific target sequences, ensuring high precision in therapies for genetic disorders.
These integrations will not only facilitate more robust analyses but also promote a more holistic approach to health and disease management.
The landscape of next-generation sequencing is continuously transforming, driven by technological advancements and the pressing need for personalized healthcare solutions.
The End
The conclusion of this article serves a critical role in distilling the essence of next-generation sequencing (NGS) technology and its significance in various fields. Summarizing key insights helps reinforce understanding and appreciation for the advancements in sequencing methods. NGS has transformed genomic research by providing deeper insights into genetic variations and complex biological systems. Therefore, recognizing the advantages of NGS over traditional methods enhances the value of this technology.
Summarizing Key Insights
Next-generation sequencing represents a leap in technology that has implications for genomics and personalized medicine. Key insights from this article include:
- Technological Advancements: NGS platforms generate massive amounts of data quickly and cost-effectively, enabling large-scale studies that were previously impractical.
- Broad Applications: From clinical diagnostics to environmental research, NGS is versatile, providing applications across disciplines.
- Ethical Considerations: The articles discusses the importance of privacy and data security in handling genetic information, highlighting that ethical issues must be managed as NGS continues to evolve.
These insights collectively illustrate how NGS is reshaping existing paradigms for understanding genetics and disease.
The Role of NGS in Future Research
Looking ahead, the role of next-generation sequencing in future research is poised to expand significantly.
NGS technology will continue to refine our understanding of genomics and will play a central role in the further development of personalized medicine. This tailor-made approach to healthcare can lead to more effective treatments, as therapies become increasingly aligned with individual genetic profiles.
Additionally, integration with artificial intelligence and machine learning will likely enhance the analysis of genomic data. These advancements will help in deciphering intricate patterns and associations that remain obscured in traditional analytical approaches. Overall, NGS stands as a crucial element in driving forward research initiatives and clinical applications in ways that will continue to benefit society.