Understanding Photocatalytic Oxidation: Principles & Applications
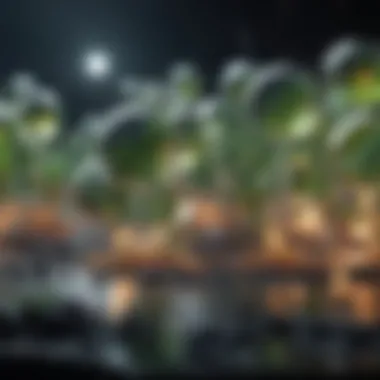
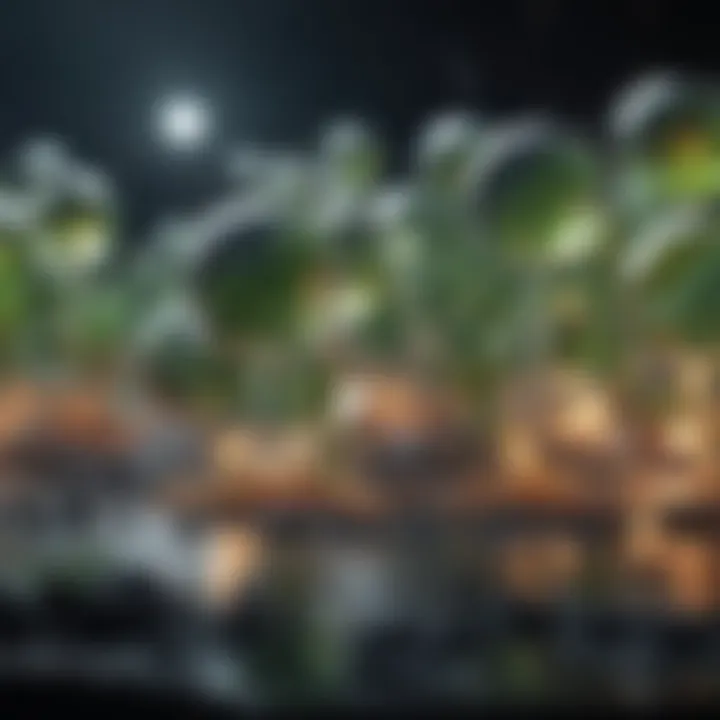
Intro
Photocatalytic oxidation is an innovative process that capitalizes on light to trigger oxidation reactions, fundamentally altering how we think about chemical transformations. This technology is not only pivotal for advancements in energy production but also crucial in applications aimed at environmental remediation. The blend of energy sustainability and ecological restoration presents a fascinating arena for both researchers and professionals.
The central aspect of this article is to delve deeper into the principles, materials, and applications surrounding photocatalytic oxidation, while also addressing current challenges and aspirations for future development within this domain.
Research Overview
Methodological Approaches
Understanding the methodology behind photocatalytic oxidation involves scrutinizing the mechanisms that drive these reactions. Photocatalysts, typically semiconductor materials like titanium dioxide, harness sunlight to produce reactive species which can oxidize various pollutants or organic substances. This research often employs a combination of experimental and computational approaches. Experimental setups may include batch and continuous flow reactors, while computational methods contribute to modeling reactions at the molecular level. The combination of these methods provides a robust framework for advancing knowledge in photochemical reactions.
Significance and Implications
The implications of photocatalytic oxidation extend across multiple fields. In environmental science, the capacity to degrade organic pollutants in water and air can significantly aid in pollution management efforts. This not only alleviates environmental decay but also contributes to public health safety. Moreover, in energy contexts, photocatalytic processes can facilitate hydrogen production, transitioning us toward greener energy sources.
Photocatalytic oxidation's significance lies in its potential to blend technology and sustainability, paving new paths to address contemporary challenges associated with energy consumption and environmental degradation.
Current Trends in Science
Innovative Techniques and Tools
Recent developments in photocatalytic oxidation include enhancing the efficiency of photocatalysts through doping, heterojunctions, and the use of nanostructures. These advancements aim to maximize light absorption and increase surface area for reactions. Techniques such as photochemical grafting and advanced characterization methods have also emerged, providing insights into the functionalization of materials, thus leading to improved photocatalytic activity.
Interdisciplinary Connections
This area of research often intersects with various scientific disciplines, including materials science, chemistry, and environmental engineering. Collaborative efforts among these fields catalyze breakthroughs in photocatalytic systems, leveraging diverse expertise to innovate solutions.
Photocatalytic oxidation, therefore, stands as a testament to how interdisciplinary efforts can significantly push forward the boundaries in technology and environmental science.
"Photocatalytic oxidation is not just a scientific endeavor; it is a bridge toward sustainable solutions for the pressing issues of our time."
Intro to Photocatalytic Oxidation
Photocatalytic oxidation is a significant area of study that blends chemistry, environmental science, and engineering. Its relevance cannot be understated in the context of contemporary challenges surrounding sustainability and energy use. Photocatalytic processes leverage light to initiate chemical reactions that can help purify air and water and degrade pollutants. This section serves as a preliminary overview, setting the tone for a deeper exploration of the principles, materials, and applications involved in photocatalytic oxidation.
Definition of Photocatalytic Oxidation
Photocatalytic oxidation refers to a chemical process that utilizes a photocatalyst to accelerate the oxidation of a substrate upon exposure to light. In this context, the photocatalyst absorbs light and generates charge carriers that facilitate oxidation reactions. This process is crucial in breaking down organic compounds and contaminants in both air and water. The key aspect here is the role of light, which acts as an energy source, enabling reactions that would not typically occur under normal circumstances.
History and Development
The history of photocatalytic oxidation dates back to the late 20th century. Initial discoveries were focused on titanium dioxide, which was found to be an effective photocatalyst. Researchers started exploring its properties and applications in various fields, including environmental remediation. It became evident that this material could break down pollutants effectively, fostering optimism about its potential. Over the years, advancements in material synthesis and characterization have expanded research into other photocatalytic materials, such as zinc oxide and graphene-based substances. This evolution reflects a growing understanding of the mechanisms of photocatalysis and its expanding significance in addressing environmental issues.
Fundamental Principles of Photocatalysis
The understanding of fundamental principles of photocatalysis is essential in grasping how photocatalytic oxidation functions. By engaging with these principles, one can appreciate the various mechanisms at play and identify why certain materials and conditions are preferred in photocatalytic processes. These principles offer insights not just into the chemical reactions, but also into design strategies for optimizing performance in applications ranging from environmental remediation to energy conversion.
Mechanism of Photocatalytic Reactions
Photocatalytic reactions rely fundamentally on the absorption of light, which initiates a series of events. When photons interact with a photocatalyst, they generate electron-hole pairs. The electrons can then be transferred to reactants, facilitating oxidation reactions. Simultaneously, the holes can oxidize water molecules. These steps are interrelated. The efficiency of these processes depends on the properties of the photocatalyst, including its band gap and surface area. A critical observation is that the specific wavelength of light and the energy level of the photons greatly influence the excitation of the electrons. Thus, understanding the precise mechanisms involved aids in refining photocatalytic systems to optimize their effectiveness.
Key Components Involved
Photocatalytic oxidation is characterized by a few critical components:
Light Source
The light source is a fundamental aspect that drives the photocatalytic process. The primary characteristic of the light source is its ability to emit photons of sufficient energy. Common sources include ultraviolet (UV) and visible light, each having unique benefits and challenges. UV light, for instance, tends to be more effective in exciting electrons in many photocatalysts due to its higher energy. However, UV light is less abundant and can create safety issues due to its potential for causing skin damage.
On the other hand, visible light has a broader availability, especially from sunlight. Though it may provide less energy for certain reactions, researchers are optimistic about developing catalysts that can easily be activated under visible light. One drawback, however, is the lower reaction rates commonly observed. The choice of light source thus becomes a balance between availability, efficacy, and safety in specific applications.
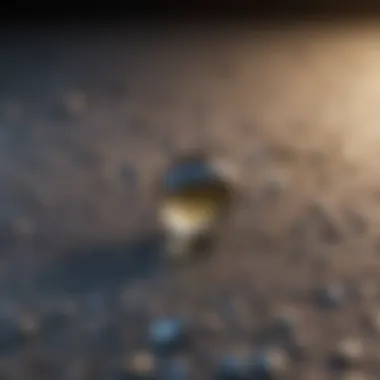
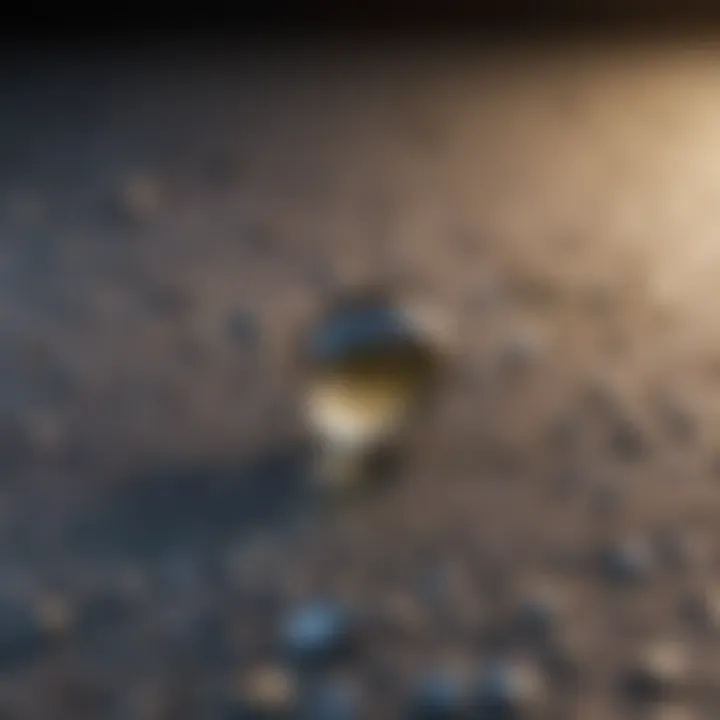
Photocatalyst
The photocatalyst is the heart of the photocatalytic process. Its role is pivotal as it mediates the absorption of light and the subsequent generation of reactive species. A key characteristic of effective photocatalysts, such as titanium dioxide and zinc oxide, is their appropriate band gap, which allows them to absorb significant portions of the light spectrum.
One unique feature of well-designed photocatalysts is their surface properties, which enhance reaction kinetics. Increased surface area allows for a greater number of active sites, facilitating better interaction with reactants. However, certain photocatalysts can experience issues of stability or reusability, especially under harsh operational conditions. Understanding these nuances ensures the selection of suitable photocatalysts tailored for specific applications.
Reactants
The reactants in a photocatalytic system are generally the substances that undergo oxidation or reduction reactions. The choice of reactants significantly shapes the overall effectiveness of the process. Typical reactants include organic pollutants, which play a crucial role in environmental applications, and hydrogen for energy applications.
A noteworthy characteristic of reactants is their varying reactivity and concentration levels, influencing the rate of photocatalytic reactions. Highly reactive substances tend to lead to quicker reaction rates, yet they can also lead to complex by-products that require further treatment. Selecting appropriate reactants can optimize the desired outputs while minimizing unwanted effects. Thus, careful consideration is needed to not only choose the right types of reactants but also to manage their concentrations for effective outcomes.
Understanding the fundamental principles of photocatalysis allows for significant advancements in sustainable technologies and environmental remediation efforts.
Types of Photocatalytic Materials
Photocatalytic materials form the backbone of photocatalytic oxidation. Their role is crucial, as they facilitate the interaction of light energy with reactants, leading to chemical transformations. Selecting the right materials is essential to optimize the photocatalytic process for specific applications such as environmental remediation and energy conversion. In this section, we dissect three main types of photocatalytic materials: semiconductors, metal oxides, and graphene-based materials.
Semiconductors
Semiconductors are pivotal in photocatalysis due to their unique electronic structures, which allow them to absorb light and generate electron-hole pairs. This property is essential for initiating oxidation reactions. Common semiconductor materials include titanium dioxide and zinc oxide.
Benefits of using semiconductors include:
- High Reactivity: They can drive various redox reactions effectively.
- Stability: Many semiconductors have stable structures that resist degradation over time.
- Wide Availability: Materials such as titanium dioxide are abundant and cost-effective.
However, there are considerations to be aware of. For instance, their light absorption capacity is often limited to ultraviolet ranges, which restricts their use under natural sunlight. Improving the absorption spectrum of semiconductor materials might enhance their applicability in various fields.
Metal Oxides
Metal oxides are categorized as another significant type of photocatalytic material. They exhibit similar properties to semiconductors but offer different advantages. Examples include tungsten oxide and iron oxide.
Key aspects of metal oxides are:
- Versatility: These materials can catalyze a wide range of chemical reactions.
- Environmental Resilience: Metal oxides often resist harsh environmental conditions, making them suitable for outdoor applications.
- Cost-Effectiveness: Like semiconductors, many metal oxides are inexpensive and readily available.
On the downside, some metal oxides have poor electronic properties, which can affect their efficiency in photocatalytic processes. Research into enhancing their conductivity and overall catalytic activity is ongoing.
Graphene-Based Materials
Graphene-based materials are emerging as innovative photocatalysts due to their exceptional electrical and thermal conductivity. Graphene oxide and reduced graphene oxide are particularly noted for their potential in photocatalytic applications.
The advantages of graphene-based materials include:
- High Surface Area: This leads to increased interaction with reactants, enhancing reaction rates.
- Electron Mobility: Superior electron transport properties can improve photocatalytic efficiency.
- Light Absorption: They can absorb a broader spectrum of light, including visible wavelengths, making them suitable for solar-driven applications.
Nonetheless, challenges exist in the synthesis and stability of graphene-based materials. Ensuring uniform distribution and effective integration with other photocatalytic systems remains a topic of intensive research.
As the field of photocatalytic oxidation evolves, the selection and development of these materials play a critical role in the advancement of sustainable technologies.
Applications of Photocatalytic Oxidation
The applications of photocatalytic oxidation span a diverse range of fields, from environmental management to energy solutions. The importance of understanding these applications lies in their potential to address current global issues, such as pollution and sustainable energy. Specifically, photocatalytic oxidation serves as a vital tool in promoting cleaner environments, efficient energy conversion, and overall sustainability.
Environmental Remediation
Environmental remediation is one of the primary applications of photocatalytic oxidation. This process has become essential in tackling various contaminants that affect air, water, and soil quality. Photocatalysts can effectively break down organic pollutants into less harmful substances when exposed to light. These reactions produce non-toxic byproducts, which contribute to a cleaner ecosystem.
The implementation of photocatalytic oxidation can be seen in several case studies. For instance, titanium dioxide is often utilized for degrading volatile organic compounds in the atmosphere. Its ability to operate effectively under ultraviolet light allows for large-scale use in urban environments where pollution is critical. Many researchers are exploring innovative methods to make these processes more efficient, focusing on optimizing the photocatalytic materials and reaction conditions.
Air Purification
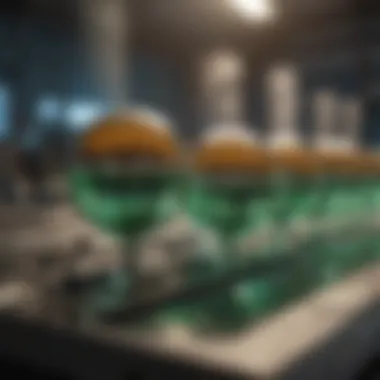
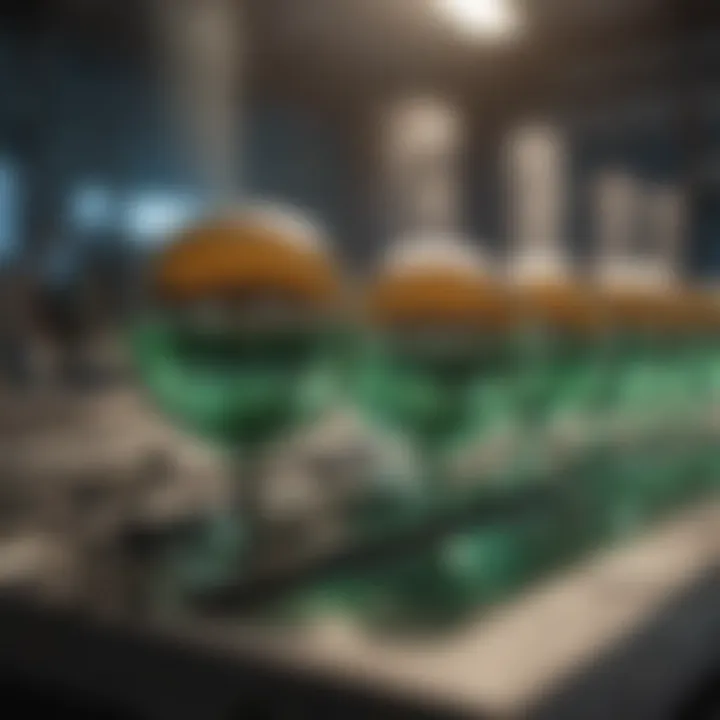
Air purification benefits significantly from photocatalytic oxidation. The technology can remove harmful pollutants and pathogens from the air we breathe. The process involves catalytic reactions that destroy a wide range of contaminants, like formaldehyde and benzene, often found in indoor environments.
Photocatalytic air purifiers are emerging as a promising solution in homes and workplaces. These devices use light to activate photocatalysts, removing airborne bacteria and volatile organic compounds. Studies show that using these purifiers can lead to significantly improved indoor air quality, making them a viable option for health-conscious consumers.
Water Treatment
Water treatment is another vital area where photocatalytic oxidation shows promise. The technique provides a method to decompose pollutants such as pesticides, pharmaceuticals, and industrial waste in water sources. This process can effectively mineralize harmful compounds into harmless residues.
Photocatalytic reactors are designed for both small-scale and large-scale applications, allowing treatment of waste streams before they can pollute natural water bodies. Additionally, the use of sunlight for these reactions can greatly reduce operational costs, making water treatment more sustainable and cost-effective.
Energy Conversion and Storage
Photocatalytic oxidation can also play a role in energy conversion and storage. By utilizing sunlight to drive chemical reactions, this technology opens pathways for producing renewable energy. For example, photocatalysts can facilitate the conversion of carbon dioxide and water into fuels, such as hydrogen or methanol, under controlled conditions.
This approach not only reduces greenhouse gas emissions but also offers a sustainable method for energy production. As research progresses, optimizing these reactions for better efficiency and yield is crucial for creating feasible applications in real-world settings.
“Advancements in photocatalytic processes could lead to groundbreaking improvements in environmental sustainability and energy solutions.”
In summary, the applications of photocatalytic oxidation demonstrate substantial promise in addressing environmental issues and energy challenges. As these technologies evolve, they hold the potential to make a significant impact on sustainability efforts in various sectors.
Advantages of Photocatalytic Oxidation
Photocatalytic oxidation has emerged as a promising solution for multiple environmental challenges. Its advantages significantly contribute to sustainability and efficiency in various applications. Understanding these benefits is crucial for both academic research and practical implementation in real-world scenarios.
Sustainability
Sustainability is at the heart of photocatalytic oxidation. This process employs light as an energy source, significantly reducing dependence on fossil fuels. By using renewable energy like sunlight, photocatalytic systems present a cleaner alternative to traditional chemical processes. The potential for utilizing sunlight aligns with global efforts to mitigate climate change and support greener technologies.
Moreover, photocatalytic oxidation can help in breaking down harmful pollutants, which improves air and water quality. For example, substances like volatile organic compounds (VOCs) can be effectively decomposed. This decomposition not only removes contaminants but also produces less harmful byproducts, contributing to a healthier environment.
Energy Efficiency
The energy efficiency of photocatalytic oxidation is another critical advantage. Traditional chemical reactions often require high temperatures and pressures, consuming large amounts of energy. In contrast, photocatalytic processes typically operate under mild conditions, utilizing light energy instead.
Many recent studies show that photocatalytic systems can achieve high conversion rates of reactants into products with low energy input. This efficiency makes photocatalytic oxidation an attractive option in industrial applications where energy costs are significant. The use of inexpensive and effective photocatalysts can further enhance this efficiency, making these processes scalable and feasible.
Cost-Effectiveness
Cost-effectiveness is also a key consideration. While the initial investment in photocatalytic technology might be notable, the long-term savings can outweigh these costs. Reduced energy consumption translates into lower operational costs. Moreover, photocatalytic materials like titanium dioxide are abundant and relatively inexpensive.
Implementing photocatalytic systems can lead to decreased expenditures related to waste management and pollution control. For instance, in water treatment plants, using photocatalytic oxidation can lower costs associated with traditional treatment chemicals. Therefore, sectors such as wastewater treatment, air purification, and even energy conversion can benefit economically from this innovative approach.
"The integration of photocatalytic oxidation technology in environmental management could lead to substantial economic and ecological benefits." - Environmental Research Group
Challenges and Limitations
The study of photocatalytic oxidation reveals significant promise in numerous fields, including environmental remediation and energy production. However, alongside its advantages, it faces distinctive challenges and limitations that can hinder its practical application and further development. Understanding these challenges is crucial for advancing research and optimizing photocatalytic systems. By addressing the obstacles, we can better harness the potential of this technology.
Material Stability
Material stability is a paramount consideration in photocatalytic oxidation. Photocatalysts need to withstand operational conditions over extended periods without substantial degradation. Certain materials often fall short in this regard, particularly metal oxides. These materials can degrade under prolonged exposure to sunlight or harsh environmental conditions, influencing their effectiveness.
In practical terms, a photocatalyst with high instability can lead to increased operational costs due to the need for frequent replacements. Moreover, the reusability of catalysts is a vital factor in assessing their performance. A stable material not only enhances efficiency but also contributes to sustainability practices by reducing waste.
Light Absorption Efficiency
Light absorption efficiency is critical because photocatalytic processes are light-driven. The ability of a photocatalyst to effectively absorb specific wavelengths determines its reactivity. Many existing materials are limited by their absorption spectra, which restricts the range of usable sunlight. This inadequacy fundamentally impacts the effectiveness of the photocatalytic process.
Enhanced light absorption can lead to improved reaction rates. Developing composite materials or hybrid systems may be beneficial to broaden the absorption range. Nevertheless, there are challenges related to cost and complexity in production. Balancing efficiency and economic feasibility remains an ongoing challenge in research.
Scalability of Applications
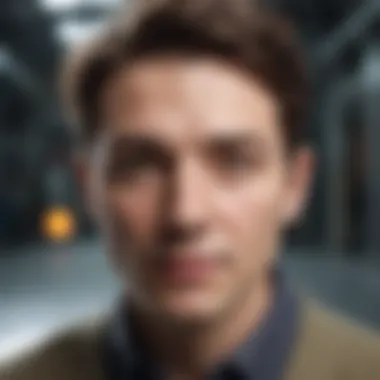
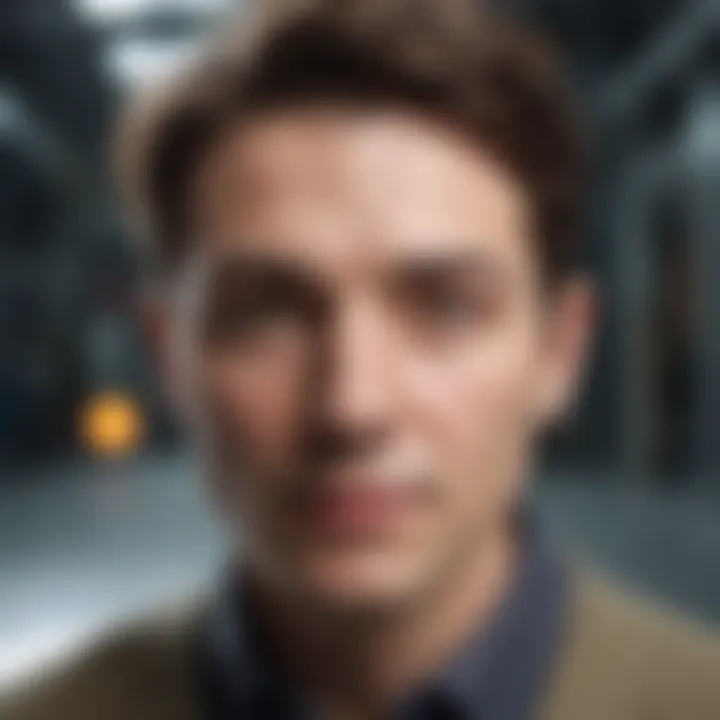
Scalability represents a significant hurdle in transitioning photocatalytic processes from laboratory settings to real-world applications. While laboratory results may show high efficacy, the performance in large-scale operations can diverge drastically. Factors such as reactor design and operational parameters play vital roles in how efficiently the process translates to the field.
Addressing scalability concerns includes considering system design, the integration of photocatalysis with existing technologies, and cost-effectiveness. It is not merely about replicating lab results on a larger scale; the challenges of maintaining consistency and efficiency must be managed carefully.
"Scaling up photocatalytic processes while maintaining performance levels is essential for their successful deployment in real-world applications."
In summary, while photocatalytic oxidation holds significant potential for innovative solutions, its development hinges on overcoming challenges related to material stability, light absorption efficiency, and scalability. Continued research in these areas will be vital for unlocking more extensive applications and for the advancement of sustainability through photocatalytic technologies.
Recent Advances in Photocatalytic Research
Recent advances in photocatalytic research play a crucial role in the evolution of this field. As the demand for sustainable and efficient solutions grows, researchers are focusing on developing materials and systems that enhance photocatalytic processes. This section outlines the significance of innovative materials and hybrid systems that are transforming how photocatalytic oxidation is applied in various industries.
Innovative Materials Development
The search for new photocatalytic materials has accelerated in recent years. Researchers have been exploring various components to improve efficiency and reduce costs. Traditional materials, such as titanium dioxide, have limitations in light absorption and reactivity. Consequently, many studies are now focusing on novel semiconductors and composite materials.
Some significant materials under development include:
- Perovskite materials: These compounds exhibit excellent light absorption properties and exhibit greater catalytic activity compared to traditional materials.
- Graphene-based materials: Their high surface area and electron mobility can significantly enhance photocatalytic performance, leading to quicker and more efficient chemical reactions.
- Metal-organic frameworks (MOFs): MOFs provide a unique structure that can be fine-tuned for specific applications, offering higher selectivity and efficiency.
Innovative materials development is not merely a matter of enhancing efficacy; it also opens doors to more sustainable practices in various sectors. Research into these materials aims to minimize environmental impact and promote more effective use of resources in photocatalytic applications.
Hybrid Systems Integration
The integration of hybrid systems represents a strategic direction in advancing photocatalytic oxidation. Hybrid systems combine different photocatalytic materials or incorporate other technologies, allowing for improved performance and wider applications. The result is a synergistic effect that enhances the overall effectiveness of photocatalytic processes.
Some key aspects of hybrid systems include:
- Multi-component photocatalysts: By combining semiconductors with varying bandgap energies, these systems can utilize a broader spectrum of sunlight, making them more effective even in low-light conditions.
- Coupling with biological systems: Integrating photocatalytic processes with biological mechanisms can lead to novel applications in waste treatment and renewable energy production, improving sustainability.
- Integration with other renewable technologies: Combining photocatalytic systems with solar panels or other energy-generation methods can maximize energy use and improve overall system efficiency.
The development of hybrid systems exhibits a promising avenue for future research. Ongoing study into these combined approaches will likely yield significant improvements in photocatalytic processes, addressing both economic and environmental challenges.
"Advances in materials and hybrid systems are paving the way for more efficient and sustainable photocatalytic processes, essential for meeting global challenges."
Future Directions of Photocatalytic Oxidation
The field of photocatalytic oxidation is evolving rapidly. Continued research is critical for advancing this technology to meet environmental and energy demands. Understanding the future directions of this field is essential in unlocking its full potential. By investigating trends and policies, stakeholders can position themselves to take advantage of upcoming opportunities.
Research Trends
Researchers are focusing on improving the efficiency and effectiveness of photocatalytic oxidation. This includes:
- Innovative Materials: Developing new photocatalysts that are more active under visible light. This makes utilization under natural conditions more feasible.
- Nanostructuring: Manipulating materials at the nanometer scale can enhance light absorption and charge separation, vital for optimizing reactions.
- Hybrid Systems: Integrating different materials can lead to synergies, enhancing performance. For example, combining metal oxides with graphene can optimize electron transfer, increasing the overall efficiency of photocatalytic processes.
Exploring these directions provides potential breakthroughs in photocatalytic applications. Incorporating machine learning to analyze reaction mechanisms is also a growing trend. This technology can enable rapid forecasting of photocatalyst performance, guiding researchers more efficiently.
Policy and Regulation Implications
The advancements in photocatalytic oxidation must be guided by sound policies. Regulations play a crucial role in ensuring that the benefits of this technology are realized equitably. Key considerations include:
- Environmental Standards: Setting benchmarks for emissions and pollutants can promote the adoption of photocatalytic systems in industrial processes.
- Funding and Grants: Government investment in research can accelerate innovation. Policies should focus on prioritizing sustainable solutions that solve real-world problems.
- Public Awareness and Acceptance: Educating the public on the benefits and safety of photocatalytic oxidation can foster community support. Often, acceptance is as critical as technology itself.
The End and Summary
The significance of photocatalytic oxidation in contemporary research and practical applications cannot be overstated. This process, which utilizes light to initiate oxidation reactions, presents robust solutions to pressing environmental and energy challenges. The careful examination of the principles of photocatalysis, the diverse range of materials available, and the multiple applications underscores the versatility of this technology. By addressing crucial issues such as air and water pollution, photocatalytic oxidation not only enhances environmental quality but also contributes to sustainable energy production.
In the discourse around photocatalytic oxidation, several key elements emerge as crucial to its development and implementation. Understanding the underlying mechanisms is vital for improving efficiency and expanding its application range. The developments in material science, particularly in enhancing the stability and effectiveness of photocatalysts, are promising. Therefore, the intersection of research advancements and practical applications is essential for realizing the full potential of photocatalytic oxidation in real-world settings.
Furthermore, exploring future directions can help direct research efforts towards overcoming existing challenges while also unlocking new applications. The importance of policy and regulation in facilitating supportive frameworks for new technologies should not be overlooked. As the field continues to evolve, fostering collaboration among researchers, policymakers, and industries will pave the way for innovations that address sustainability in a comprehensive manner.
"Photocatalytic oxidation represents a confluence of science and practical application, addressing some of the most critical challenges of our time."
Key Takeaways
- Essential Mechanism: Photocatalytic oxidation operates by utilizing light to drive oxidation reactions, highlighting the significance of understanding this mechanism for practical applications.
- Diverse Applications: The process finds place in a variety of fields, notably environmental remediation, air purification, and renewable energy generation.
- Material Innovation: Ongoing research on photocatalytic materials is pivotal to enhancing effectiveness, stability, and efficiency. This also extends to hybrid systems that can optimize performance.
- Future Perspectives: Exploring future trends is crucial, emphasizing the need for policy frameworks that support research and practical implementation.
- Collaborative Efforts: Success in this field relies not only on scientific advancements but also on effective collaboration among researchers, policymakers, and industries.
In summary, photocatalytic oxidation stands at the forefront of addressing critical environmental and energy issues. Engaging with this topic provides a robust framework for future inquiries and applications, making it an important area for continued research and exploration.