Protein Purification Techniques in E. Coli
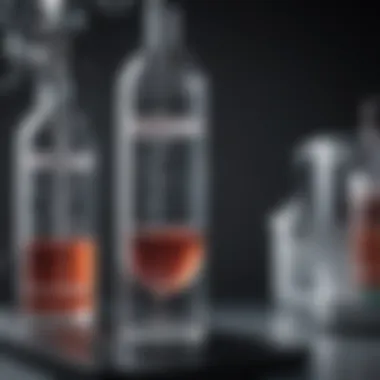
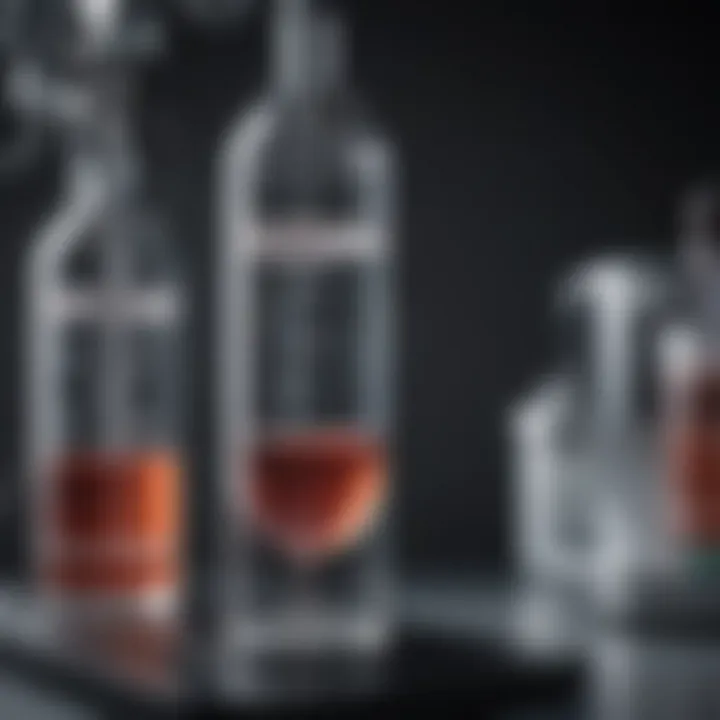
Intro
Protein purification is a fundamental step in molecular biology, particularly when using Escherichia coli as a host organism. The intricate pathways that these proteins take during purification can be as winding as a river, yet understanding them opens up a world of opportunities in fundamental and applied research. In this article, we will explore various techniques used in purifying proteins from E. coli, alongside key considerations that drive the process. With E. coli being a workhorse in biotechnology and pharmaceuticals, knowing how to effectively purify proteins from this bacterium is nothing short of vital.
The methods we delve into—like affinity chromatography and ion-exchange chromatography—are tried and true tools that researchers have honed to achieve reliable yields of high-purity proteins. However, the art of protein purification is not solely about the techniques; it also encompasses a thoughtful approach to variables such as protein stability and yield maximization. This intersection of science and technique is where we'll cast our net, ensuring you not only grasp the theoretical underpinnings but also the practical realities of protein purification.
Research Overview
Methodological Approaches
When it comes to isolating proteins from E. coli, a menu of methodologies awaits to be chosen based on specific goals and resources. Here are some of the main techniques:
- Affinity Chromatography: This method exploits specific interactions between the target protein and a ligand immobilized on a solid support. If done right, it’s as straightforward as pie.
- Ion-Exchange Chromatography: Capitalizing on the charge properties of proteins, this technique is effective in separating proteins under varying pH and salt concentrations. It’s a balancing act similar to walking a tightrope, where small changes can have major effects.
- Gel Filtration Chromatography: Also known as molecular sieve chromatography, this method separates proteins based on size. Think of it as navigating through a crowded room—only those small enough can slip through the gaps.
Each technique comes with its own idiosyncrasies and requires a careful assessment of the specific protein and the desired end outcome.
Significance and Implications
The significance of purifying proteins is not just a matter of academic interest; it has far-reaching implications. The proteins extracted from E. coli can be used for:
- Drug Development: Many therapeutic agents, including insulin, are derived from purifying proteins from this bacterium.
- Research: Protein interactions and functions are critical areas of study that rely heavily on purified proteins to yield reliable data.
- Industrial Applications: Enzymes and other products derived from proteins play substantial roles in sectors like food technology and biofuels.
Understanding these pathways can equip scientists with a better grasp of protein behavior, ultimately leading to breakthroughs in various fields.
"The purification of a single protein can illuminate the intricate dance of biological processes."
Current Trends in Science
Innovative Techniques and Tools
As the scientific community pushes the envelope, novel tools and techniques emerge, ensuring that protein purification remains at the forefront of research. Innovations such as:
- Microfluidics: Streamlining the purification process by allowing small volumes of samples to be processed rapidly and effectively.
- Automated Systems: Equipment designed to enhance productivity while minimizing human error.
- Nanoparticles: Used to improve specificity and capture of target proteins, increasing yield and purity.
These advancements promise to reshape the landscape of protein purification in ways eluding traditional methodologies.
Interdisciplinary Connections
The protein purification landscape is highly interconnected. Fields such as bioinformatics play a significant role in predicting protein behavior and attributes before purification begins. Collaborations across disciplines, like chemistry and molecular biology, pave the way for innovative problem-solving approaches to these persistent challenges.
Protein purification from E. coli is, without a doubt, a pilgrimage worth taking for anyone aiming to conquer the intricacies of protein function and application. By understanding both the techniques and the resultant implications, we take one step closer to mastering the art of science that feeds the worlds of medicine and industry.
Prelude to Protein Purification
Protein purification is a cornerstone in the field of molecular biology, serving as a bridge between understanding cellular mechanics and harnessing biological engineering for research and commercial applications. The significance of this process cannot be overstated; it is the pathway through which scientists isolate proteins for detailed study, enabling advancements in various arenas like therapeutics, diagnostics, and fundamental biology.
The essence of protein purification lies in its ability to yield proteins free of contaminants, allowing researchers to probe their structure and function. With the increasing demand for high-quality proteins in pharmaceuticals, the techniques used become not just useful; they become critical.
Efficiency, yield, and purity are the three pillars upon which effective protein purification rests. A robust protocol ensures that the protein of interest remains functional while being isolated from the myriad other cellular components that comprise E. coli. This aspect emphasizes the importance of tailored strategies depending on the unique characteristics of the protein and its intended end-use.
"In the world of biochemistry, isolated proteins are the key to unlocking a plethora of biological mysteries."
Choosing Escherichia coli as a host organism amplifies those efforts. It delivers rapid growth and the capability to express a range of proteins, which enhances the overall productivity of purification processes. This article will delve into the nuances of purifying proteins from E. coli, focusing on the specific techniques, considerations, and best practices that can make or break a research outcome.
Furthermore, understanding the underlying principles of protein behavior and the broader contexts in which these proteins are used allows for innovative approaches that can lead to groundbreaking discoveries. As the article unfolds, we will explore the significance of protein purification, the specific advantages of using E. coli, and the overarching methodologies that guide this crucial process.
Significance of Protein Purification
In the grand scheme of biological research, the purification of proteins holds immense significance. Proteins, as workhorses of the cell, play critical roles in nearly every biological process. Therefore, uncovering their individual functions requires meticulous isolation. In particular, a few key reasons underscore the necessity of protein purification:
- Functional Analysis: Purified proteins are essential for detailed studies of biological functions, enzyme activity, and interaction mapping. Without purification, understanding these complex mechanisms becomes an uphill battle.
- Therapeutic Applications: Many proteins serve as biopharmaceuticals or are used in the development of therapeutic agents. Their purity is paramount to ensure safety and efficacy.
- Structural Studies: Structural biology relies heavily on the high purity of proteins for methods such as X-ray crystallography or NMR spectroscopy. Contaminants can obscure or distort critical data.
By improving our techniques in protein purification, we essentially pave the way for the next significant breakthroughs in biochemistry.
E. Coli as a Host Organism
When it comes to selecting a host organism for protein expression, E. coli stands out as a popular choice. The bacterium offers several distinct advantages:
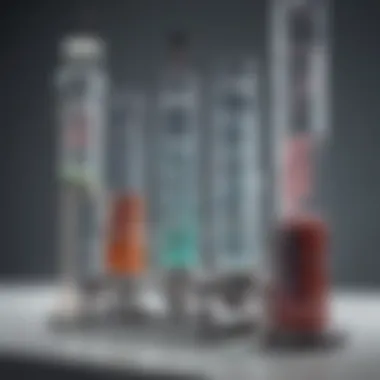
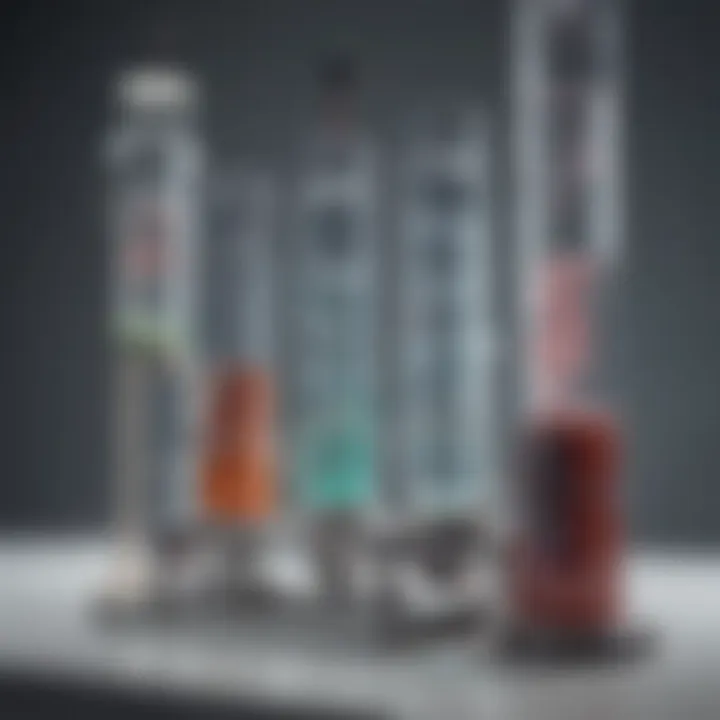
- Fast Growth and High Yield: E. coli can double its population in just 20 minutes. This means researchers can obtain large quantities of protein in a short span of time.
- Well-Characterized System: Decades of research have provided a thorough understanding of E. coli genetics and metabolism. This familiarity engenders confidence in experimental design and execution.
- Cost-Effective: Growing E. coli in laboratory settings is relatively inexpensive compared to eukaryotic systems, which often require more resources and time.
Despite its advantages, using E. coli is not without its challenges. It lacks certain post-translational modification systems, which can be crucial for the proper functioning of some eukaryotic proteins. Nevertheless, with strategic engineering, many of these issues can be mitigated.
In sum, the choice of E. coli as a host organism significantly influences the success of protein purification efforts. It provides a fertile ground for burgeoning fields like synthetic biology and bioengineering, making the purification process all the more relevant and exciting.
Fundamental Concepts of Protein Purification
Understanding the foundational aspects of protein purification is crucial for anyone engaged in biochemical research or industrial applications. The process is not merely about obtaining proteins; it involves comprehending their unique properties, behaviors, and the specific objectives behind purification. This section aims to lay a solid groundwork that will guide the exploration of various techniques later on.
Protein Properties and Behavior
Proteins are complex molecules, each with distinct properties that dictate how they behave in various environments. Factors such as size, charge, hydrophobicity, and stability are pivotal. For example, the size of a protein influences the choice of purification methods; larger proteins may traverse gel filtration systems effectively, while smaller proteins might require different strategies to optimize separation.
Solubility is another critical factor. Some proteins readily dissolve in aqueous solutions, while others precipitate easily. This property can guide the initial steps of protein extraction. Further, the isoelectric point (pI), which is the pH where a protein carries no net charge, plays a significant role in ion-exchange chromatography. Ensuring that the protein is separated in an environment conducive to its stability and activity is essential.
"Grasping the nuances of protein characterization can dramatically enhance purification results."
There are also additional considerations regarding the thermal and chemical stability of proteins. Exposure to harsh conditions can lead to denaturation, thus impacting yield. Hence, researchers must keep a weather eye on conditions such as temperature and pH, always optimizing for the specific protein of interest. These properties form the backbone of a successful purification strategy, as tailoring the approach based on understanding these characteristics directly impacts efficiency and effectiveness.
Purification Goals
Defining the goals of purification is one of the first steps an investigator must take. It's not merely about achieving a high concentration of a protein but understanding the intended application. The goals may differ significantly based on whether the protein will be used in research or industrial applications.
Some common purification goals include:
- Maximizing purity: Isolating the target protein from contaminants and other cellular components.
- Enhancing yield: Obtaining as much protein as possible while minimizing losses.
- Retaining functionality: Ensuring the protein maintains its biological activity and structural integrity.
- Facilitating downstream applications: Preparing the protein in a way that makes it suitable for future experiments, be it enzymatic activity tests, binding studies, or therapeutic developments.
Identifying a clear goal can streamline the purification process, reducing trial and error that is often the bane of protein scientists. By keeping these objectives in focus and adapting techniques accordingly, researchers can more efficiently navigate the complicated landscape of protein purification. The following sections will delve into specific techniques that offer solutions tailored to these varied qualifications.
Common Techniques in Protein Purification
Protein purification remains a cornerstone in molecular biology, especially when using E. coli as a host. Mastering these techniques brings both theoretical knowledge and practical skills into play. Every method discussed below carries its own merits, challenges, and ideal applications, making it essential for researchers and industries alike to navigate through them effectively.
Affinity Chromatography
Affinity chromatography is often hailed for its specificity when it comes to isolating desired proteins. It's built on the premise that proteins have a unique affinity for certain ligands, allowing for more straightforward separation from a mix of proteins.
Principles of Affinity Chromatography
At the heart of affinity chromatography lies the interaction between a specific ligand and its target protein. When a cell lysate is treated with a column containing the ligand, only the protein of interest binds, while others flow through. This technique leverages the unique properties of the target protein, which can make it a popular choice for those seeking high-purity outputs. The ability to finely tune conditions, such as pH and ionic strength, adds to its appeal. However, one must weigh the advantage of high selectivity against the potential drawback of using ligands that may alter protein structure or functionality.
Applications of Specific Ligands
Specific ligands provide unique hooks for proteins, immensely contributing to the effectiveness of affinity chromatography. A common application is using His-tagged proteins, which bind to nickel ions in the column. This method is user-friendly, making it a go-to in many labs. The distinct feature here is the versatility of the ligands, allowing researchers to target a wide range of proteins with tailored approaches. However, a downside is the possibility of non-specific binding, which could lead to contaminants that muddle results.
Ion-Exchange Chromatography
Ion-exchange chromatography serves as another pillar of protein purification, relying on the charge properties of proteins to separate them.
Mechanisms of Ion-Exchange
In ion-exchange chromatography, proteins are retained on a column filled with charged resin—positively charged resin captures negatively charged proteins, and vice versa. The elegance of this design allows for a wide range of proteins to be separated based on their charge states. This method is thought to have a robust capability to achieve high resolution in protein purification. Some researchers find its adaptability appealing; however, care must be taken as the results can vary with small changes in conditions.
Selecting pH and Ionic Strength
When it comes to optimizing ion-exchange chromatography, selecting the right pH and ionic strength is crucial. The pH influences the overall charge of the protein, while ionic strength affects the ionic environment within the column, determining the degree of interaction between proteins and the resin. A well-chosen pH can enhance binding significantly but requires careful consideration to avoid protein denaturation. Thus, navigating these parameters is essential for achieving the desired outcome.
Gel Filtration Chromatography
Gel filtration chromatography, also known as size-exclusion chromatography, rounds out the arsenal of protein purification techniques. The principle here is fairly intuitive: larger molecules pass through the column faster than smaller ones.
Size Exclusion Principles
The basic premise of size exclusion is that a porous matrix allows smaller proteins to enter particles while letting larger proteins pass around them. This leads to a clear separation based on size. The notable feature of this technique is its gentle nature, preserving protein integrity because there are no harsh conditions, unlike other methods. This characteristic makes it particularly useful when dealing with delicate proteins, though it is not suited for purifying proteins based solely on their charge or binding affinity.
Limitations of Gel Filtration
Despite its usefulness, gel filtration chromatography does come with limitations. For one, it generally does not offer high resolution for closely sized proteins, which might lead to muddled results or loss of target proteins in fractions. Another aspect to consider is the time factor; this method can often be slower. Therefore, while gel filtration is yet another tool added to the purification toolbox, the user must remain ever mindful of its constraints.
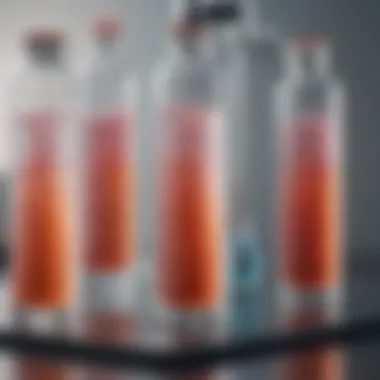
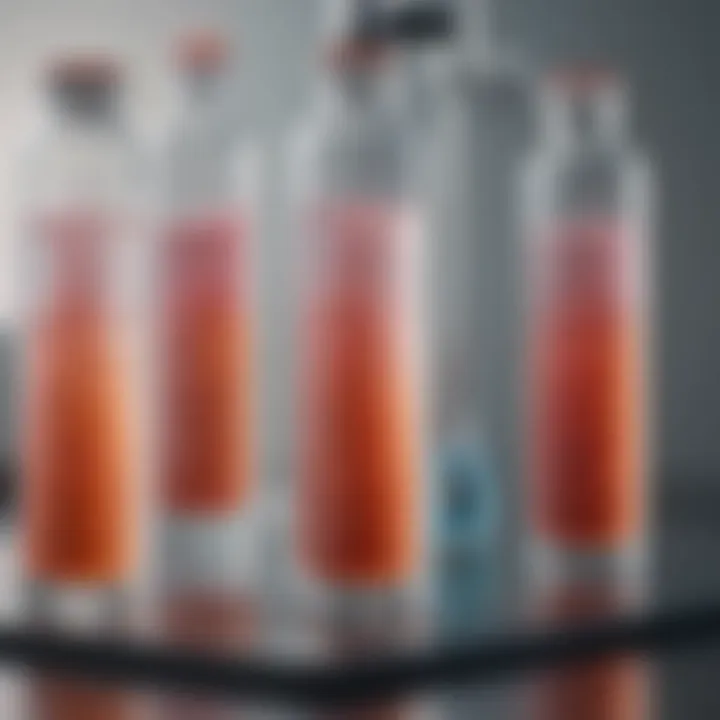
In summary, employing these common techniques—affinity, ion-exchange, and gel filtration chromatography—requires a discerning approach. Each has its unique strengths and caveats, making it essential to align the chosen method with specific purification goals.
Optimizing Purification Processes
Optimizing protein purification processes is crucial for achieving high yields and maintaining protein functionality. This aspect focuses on various strategies to enhance the efficiency and efficacy of purification steps, ensuring the final product is not only abundant but also biologically active. Proper optimization can also lead to significant cost savings and reduced wastage of resources, making it a vital part of the protein purification pipeline when using E. coli.
Maximizing Protein Yield
Adjusting Expression Conditions
Adjusting expression conditions involves fine-tuning several aspects of E. coli growth to maximize protein yield. Key factors include temperature, induction timing, and nutrient composition. Lowering the temperature during protein expression often slows down the metabolism of E. coli, allowing the cells to fold proteins properly. This results in higher amounts of correctly folded, active protein, which is often a top goal in purification processes.
One common approach is modifying the IPTG concentration in E. coli cultures; this allows flexibility in determining optimal induction levels for proteins with different folding kinetics. The critical characteristic of adjusting expression conditions is its flexibility. It can be tailored to specific proteins, making it a beneficial strategy for researchers targeting different projects.
However, it comes with potential downsides, such as increased costs due to additional media or slower growth rates which can affect the overall timeline of the experiment. Nonetheless, when executed effectively, adjusting expression conditions can lead to significant improvements in yield and activity of the protein.
Disruption of E. Coli Cells
Disruption of E. coli cells is essential for releasing the target protein from the cellular environment. Various techniques, such as sonication or enzymatic lysis, are employed to break open the cells. One significant advantage of disruption techniques, particularly sonication, is its efficiency in processing large volumes of culture swiftly.
The method tends to generate significant heat, which can affect protein stability, pointing to its unique feature of needing precise control during application. Still, the advantages can outweigh the disadvantages, especially when dealing with proteins that are expressed in high volumes in E. coli. This step ensures that the purification process effectively extracts the maximum quantity of protein, enhancing overall yield.
Ensuring Protein Stability
Temperature and pH Effects
Temperature and pH play pivotal roles in maintaining protein stability during purification. Proteins are sensitive to environmental conditions; thus, understanding how variations in temperature and pH affect stability is essential. For instance, most proteins have an optimal pH range in which they fold correctly and maintain their active form. Deviations can lead to denaturation, which jeopardizes the purification goals.
The core characteristic of being mindful of temperature and pH is that they are easily adjustable parameters within the purification protocol, making it a practical approach. Employing buffers can help stabilize the pH during purification processes, which is often a straightforward method for keeping proteins in their active forms. Nevertheless, if not managed properly, fluctuations in these factors can lead to significant losses of functionality, making robust monitoring during purification a necessity.
Additives for Stabilization
Additives for stabilization, such as glycerol, salts, or specific cofactors, play an important role in maintaining protein structure and function during purification. These substances can create a protective effect, helping to keep the proteins soluble and active under various conditions. The unique feature of using additives is their ability to provide specific environments that mimic physiological conditions, making them particularly beneficial.
However, it’s worth noting that choosing the right additives can require considerable experimentation. Different proteins respond uniquely to various stabilizing agents. Thus, while additives can greatly enhance stability, the need for extensive testing can often prove to be a double-edged sword. Nonetheless, optimizing the use of these stabilizers is an integral tactic for ensuring protein purity and functionality throughout the purification process.
Proper optimization in protein purification can lead to significant cost savings and enhanced protein quality, ultimately benefiting both research and industrial applications.
Applications of Purified Proteins
Purified proteins play a vital role in various fields, from cutting-edge research to large-scale industrial production. By isolating proteins with precision, scientists and industries can harness their unique properties for targeted applications. Understanding these applications not only underscores the significance of protein purification but also provides insights into how these proteins can catalyze advancements in technology and medicine.
Research Applications
Research applications of purified proteins encompass diverse areas of study, allowing scientists to delve deeper into fundamental biological processes, enzymatic functions, and molecular interactions. These applications excite researchers as they can lead to groundbreaking discoveries that enhance our understanding of life itself.
Enzyme Studies
Enzyme studies are paramount in research, shedding light on intricate biochemical pathways. The unique characteristic of enzymes is their ability to catalyze reactions efficiently, often under mild conditions. This makes enzyme studies a popular choice for researchers aiming to examine metabolic processes, enzyme kinetics, or mechanisms of action.
- Contribution to Understanding: By isolating and purifying specific enzymes, researchers can dissect their roles within various biochemical pathways, significantly contributing to the broader understanding of cellular functions and metabolic regulation.
- Advantages and Disadvantages: One unique feature of enzyme studies is that purified enzymes can be utilized in high-throughput screening assays. This provides a significant advantage in drug discovery and enzyme engineering. However, the disadvantage lies in the risk of isolation affecting enzyme activity and stability, potentially leading to misleading results.
Structural Biology
Structural biology focuses on determining the three-dimensional structures of proteins at atomic resolution. This aspect is vital for understanding how proteins function and interact. The ability to purify proteins to homogeneity is critical in this field, given that protein conformation directly influences its biological activity.
- Importance in Research: By studying the structures of proteins, researchers can elucidate the mechanisms of action and design inhibitors or modulators that target these proteins—a critical step in drug development.
- Unique Features and Challenges: A significant aspect of structural biology is the reliance on purified proteins for techniques like X-ray crystallography and NMR spectroscopy. While these methods provide rich structural information, the challenge often lies in obtaining high-quality crystals, which can be tricky and time-consuming.
Industrial Applications
In the industrial arena, purified proteins are invaluable for developing products and processes. These applications not only enhance efficiency but also contribute to innovation across various sectors such as pharmaceuticals, food production, and biofuels.
Biopharmaceutical Development
Biopharmaceutical development capitalizes on purified proteins to create therapeutic agents, ranging from monoclonal antibodies to recombinant proteins. This area is rapidly growing due to the increasing demand for targeted treatments and biologics that offer improved efficacy and specificity over traditional small-molecule drugs.
- Significance and Popularity: The ability to produce highly purified proteins significantly impacts the development of safer therapeutic options. Companies invest heavily in optimizing purification processes to meet stringent regulatory guidelines, which makes this a highly sought-after application of purified proteins.
- Advantages and Limitations: A unique feature of biopharmaceutical development is its capacity for customization, allowing tailored therapies for individual patients. However, the downside often includes the high production costs and lengthy timelines associated with bringing these therapies to market.
Bioprocessing Techniques
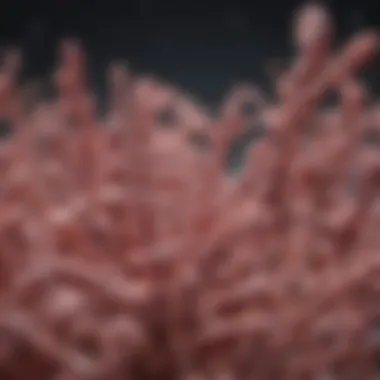
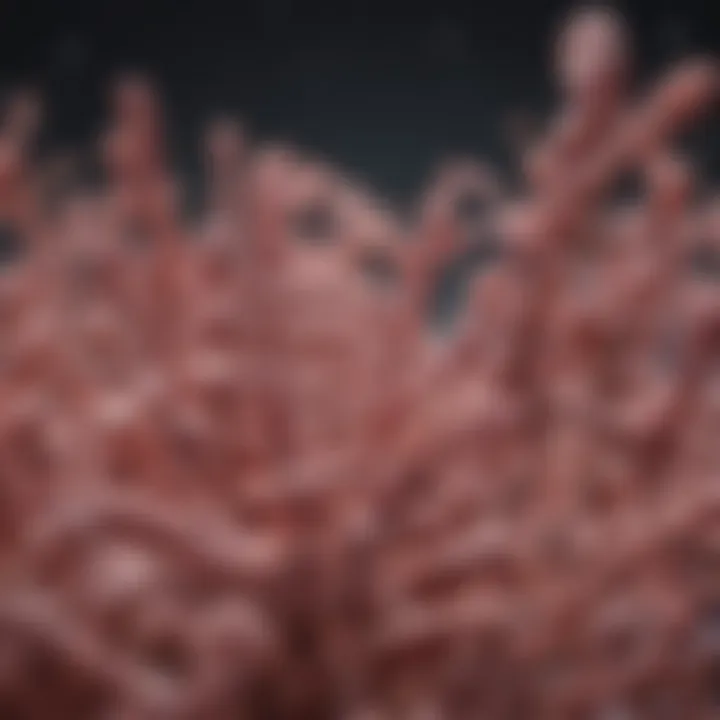
Bioprocessing techniques utilize purified proteins to streamline industrial processes, from food manufacturing to the production of biofuels. The efficiency offered by these techniques contributes to sustainability and cost-effectiveness, making them increasingly popular in various sectors.
- Contribution to Industry: These techniques often employ enzymes to catalyze reactions or modify substrates, leading to increased yields and improved product quality. For instance, in the food industry, enzyme-treated fermentation processes can enhance flavor and texture.
- Unique Feature and Trade-offs: A notable feature of bioprocessing is its adaptability; companies can adjust purification methods to optimize performance based on varying production demands. However, this flexibility also poses challenges, as maintaining consistent quality throughout production can be difficult.
The importance of purified proteins extends beyond simple isolation—these proteins are the backbone of innovations in research and industry, driving forward advancements that reshape our understanding and applications of biological sciences.
Challenges in Protein Purification
When it comes to purifying proteins from E. coli, it’s crucial to acknowledge the hurdles that often crop up. These challenges can dictate the efficiency and effectiveness of the entire purification process. Grappling with issues like protein aggregation and contaminant removal not only affects overall yield but can also have significant implications for the functionality and stability of the purified proteins. Understanding these challenges is essential for anyone in the field, especially students and professionals who are diving into the realm of molecular biology.
Protein Aggregation
Protein aggregation is a notorious challenge that many researchers face during purification processes. This phenomenon occurs when proteins misfold or interact improperly, leading them to clump together. Imagine trying to untangle a knotted ball of yarn; it can be frustrating and time-consuming.
Several factors can contribute to protein aggregation:
- Temperature Fluctuations: High temperatures can increase kinetic energy, resulting in improper folding.
- Concentration: Higher concentrations of proteins can lead to intermolecular interactions that promote aggregation.
- pH: The protein's surrounding pH can impact its charge and, consequently, its solubility.
To combat aggregation, scientists often take a few routes. Refolding strategies may be employed, where the aggregated proteins are denatured and then gradually refolded in a conducive environment. Another strategy could involve the use of additives such as arginine or glycerol, which can prevent aggregation by stabilizing the unfolded state of the proteins. The skills of a lab technician can significantly influence the success of these strategies; the right touch can mean the difference between success and a messy failure.
"To achieve high yield during purification, one must not overlook the potential pitfalls of protein aggregation."
Contaminant Removal
Another major challenge in protein purification is removing contaminants effectively. Contaminants can range from unwanted proteins (like those from E. coli) to nucleic acids, lipids, and even residual reagents from extraction processes. The presence of such impurities can compromise the purity and functionality of the target protein, making it essential to have robust strategies in place for their removal.
Several methods can be applied to ensure contaminants are kept at bay. Here are some popular techniques:
- Precipitation Techniques: By adding salts (like ammonium sulfate), it’s possible to selectively precipitate impurities based on their solubility.
- Affinity Chromatography: This method utilizes specific ligands to bind the target protein, allowing contaminants to be washed away.
- Dialysis and Filtration: These techniques help remove small molecules and salts while retaining the desired protein.
Ultimately, the method selected for contaminant removal should align with the specific characteristics of the desired protein as well as the type of contaminants present. Being meticulous in this phase can save a researcher countless hours in downstream processing.
In summary, addressing the challenges of protein aggregation and contaminant removal is vital for anyone involved in the protein purification process in E. coli. Understanding these issues leads to more efficient practices and ultimately contributes to the success of research and industrial applications.
Future Directions in Protein Purification
The landscape of protein purification is ever-evolving, especially when coupled with the insights gained from E. coli as a prominent expression system. As we stand on the brink of major advancements, it’s essential to delve into what the future holds in the realm of protein purification. This exploration is not just an academic exercise but a necessary shift, ensuring we keep pace with the demands of both basic research and industrial applications.
The integration of new technologies and approaches will likely revolutionize how we purify proteins, addressing existing challenges and embracing efficiency. The future of protein purification isn’t only about refining techniques; it also hinges on our ability to adapt systems that can meet the specifications of diverse applications, ranging from therapeutic proteins to industrial enzymes. Understanding these elements can significantly enhance yield, purity, and the reproducibility of the results we aim for in our experiments.
Advancements in Technologies
Tech innovations are at the heart of the future directions in protein purification. Several cutting-edge techniques are being explored or have already emerged, showcasing the potential to streamline processes while enhancing outcomes. For instance, the adoption of microfluidic devices offers a more controlled environment for purification. These miniaturized systems can reduce the volume of reagents needed, ultimately lessening waste and associated costs.
- High-Throughput Screening: This technology allows scientists to analyze multiple conditions in parallel, drastically cutting down the time spent on purification. Techniques like automated liquid handling can take the grunt work out of sample preparation.
- Enhanced Chromatography Techniques: The introduction of sub-2 micron particles in chromatography columns has shown promise in increasing resolution and speed of separation. This means purifying proteins faster without sacrificing the quality.
- New Affinity Ligands: Innovative ligands that can specifically bind to target proteins with higher efficiency are being developed. These ligands can either be synthetic or derived from natural sources, providing a wider choice for researchers aiming for specificity.
Incorporating such advancements ensures that purification not only meets but exceeds the current standards in various fields ranging from pharmaceuticals to food science.
Integration of Automation
The integration of automation stands to reshape protein purification significantly. As in many other scientific disciplines, automating the purification workflow offers both practical and economic advantages. By reducing human error and labor-intensive practices, researchers can focus on more intricate aspects of protein study and exponentially increase throughput.
- Automated Purification Platforms: These systems can perform multiple purification steps without manual intervention, increasing efficiency while reducing the likelihood of contamination. Imagine running a complex purification on a large scale, where setup is almost entirely self-sufficient.
- Data Management: Automated systems typically come equipped with robust data management capabilities, providing detailed logs of all purification runs. This can help in troubleshooting and ensures compliance with regulatory standards, especially critical in biopharmaceutical manufacturing.
- Real-Time Monitoring: Automation can also allow for real-time monitoring of the purification process, providing dynamic adjustments based on pre-set criteria. Such responsiveness can significantly improve the quality and consistency of the purified proteins.
As we look into the future of protein purification in E. coli, it becomes clear that our current methodologies are ripe for innovation. The continual pursuit of improving techniques not only impacts scientific progress but also poses implications for healthcare, environmental sustainability, and the advancement of technology itself.
By investing in these advancements and embracing automation, the field of protein purification can enhance both its efficiency and effectiveness, ultimately leading to breakthroughs that were once thought to be out of reach.
Finale
The conclusion serves as a vital summary, tying together the threads of discussion throughout the article on protein purification in E. coli. It holds significance not just as a recap, but as an opportunity to underscore the relevance of each technique and consideration.
Recap of Key Techniques
In pursuing protein purification, several techniques stand out for their distinctive advantages and applications:
- Affinity Chromatography leverages specific interactions between a protein and a binding partner, offering high purity yields. This method is particularly suited for proteins with defined tags or specific ligands.
- Ion-Exchange Chromatography utilizes ionic interactions to separate proteins based on their charge. Understanding pH and ionic strength is crucial for optimizing this technique, providing versatility in purification strategies.
- Gel Filtration Chromatography employs size exclusion to separate proteins, allowing practitioners to efficiently remove small molecules and aggregates, although it is limited by size range. By synthesizing these techniques, researchers can tailor their purification workflows to achieve desired outcomes, whether that be purity, yield, or stability.
Final Thoughts on E. Coli Purification
E. coli remains an unparalleled choice for protein expression due to its rapid growth, well-understood genetics, and ability to facilitate the purification of recombinant proteins. However, effective purification is riddled with challenges, including protein aggregation and the necessity for stringent contaminant removal.
"The secret to successful protein purification lies not just in the choice of technique, but in understanding the distinct characteristics of your target protein." The future of E. coli protein purification is likely to see advancements in automation and technology integration. By embracing these innovations, coupled with foundational techniques discussed herein, scientists and engineers can navigate the complexities of protein purification more effectively.
Ultimately, as research and industrial applications demand higher efficiency and specificity from purified proteins, the considerations presented in this article serve as a guide for ongoing exploration in the field.