Exploring the Intricate Structure of Red Blood Cells
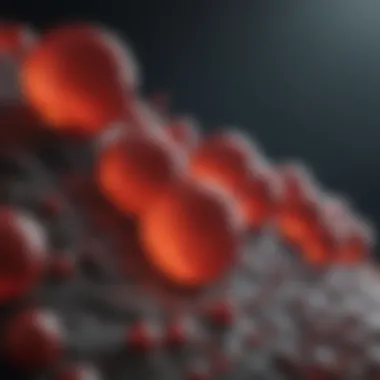
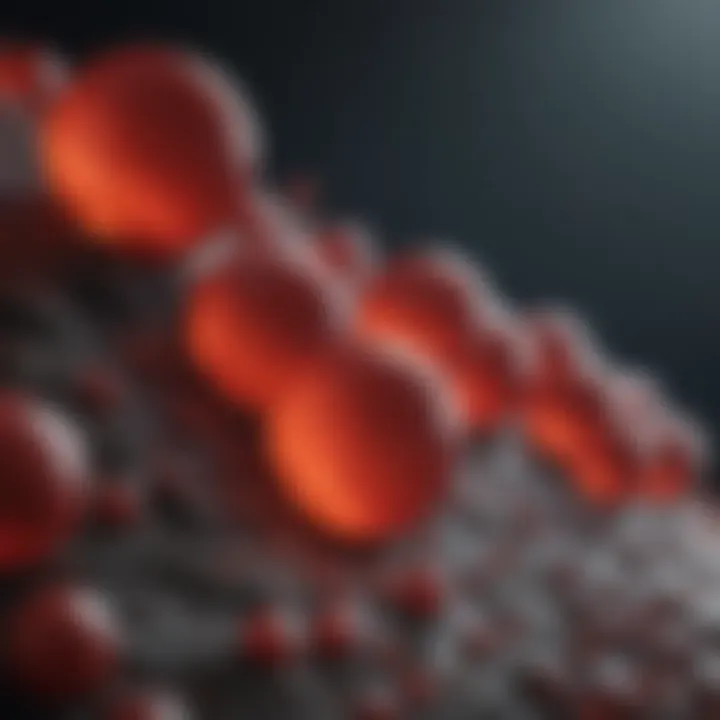
Intro
Red blood cells, or RBCs, play a vital role in maintaining the body's health. They aren't just simple cellular structures; instead, they are intricately designed to carry oxygen, and their unique features make them essential for life. This article aims to peel back the layers on these fascinating cells, diving into their structure, function, and importance in human physiology, as well as examining how they vary across different species.
As we venture through the cellular landscape, we will uncover elements of red blood cells that are not immediately obvious. We’ll discuss how their biconcave shape allows for more surface area for gas exchange, making them efficient transporters of oxygen. Moreover, the journey will lead us to understanding the cellular composition and membrane structure of RBCs, which underpins their functionality.
Whether you’re a student, educator, or researcher, this exploration aims to enrich understanding and spur further contemplation about these remarkable components of the bloodstream.
Research Overview
Methodological Approaches
Delving into the structure of red blood cells necessitates a comprehensive approach. Various methodologies come into play:
- Microscopy Techniques: Light and electron microscopy allow us to visualize the intricate structures of RBCs at different magnifications.
- Biochemical Analysis: Studying the membrane proteins and lipid compositions gives insight into the physiological functions of these cells.
- Genetic Studies: Exploring the genetic factors that influence red blood cell development helps us understand anomalies that may arise.
Each of these methods provides a unique lens through which to view RBCs, contributing valuable data to this multifaceted topic.
Significance and Implications
Understanding the structure and function of red blood cells is crucial for several reasons:
- Clinical Relevance: Abnormalities in RBC structure, such as in sickle cell anemia, can lead to significant health issues.
- Biological Insights: Studying RBCs across different species provides a window into evolutionary adaptations and ecological roles.
- Research Advancements: Current studies aim to develop therapeutic techniques for blood-related disorders, enhancing treatment options for patients.
By dissecting the complexities of RBC structures, we can derive insights that impact both basic biological research and clinical applications.
Current Trends in Science
Innovative Techniques and Tools
Recent technological advancements have propelled research on red blood cells into new territories. Some noteworthy trends include:
- Single-Cell Sequencing: This cutting-edge technique allows scientists to analyze individual RBCs, revealing heterogeneity in cellular function and response.
- 3D Imaging: Advanced imaging techniques provide a comprehensive view of red blood cell interactions within the vascular system.
These innovations amplify our ability to study RBCs and their pivotal roles in health and disease.
Interdisciplinary Connections
As science progresses, the study of red blood cells is becoming increasingly interdisciplinary. Collaboration across various fields has opened up new avenues:
- Materials Science: Researchers are exploring biomimetic materials inspired by the membrane of red blood cells for drug delivery systems.
- Artificial Intelligence: Machine learning algorithms are being utilized to analyze and predict patterns in RBC behavior.
The blending of these disciplines enriches the study of RBCs, fostering a deeper understanding and broader implications in medicine and biology.
Understanding red blood cells isn’t just an academic exercise; it’s a pathway to unraveling many mysteries of human biology and health.
Intro to Red Blood Cells
The study of red blood cells (RBCs) is crucial for understanding not just human physiology but the broader processes that sustain life in many organisms. These cells, often referred to as the body’s oxygen carriers, are fundamental in ensuring that tissues receive the oxygen necessary for cellular metabolism.
RBCs exhibit a fascinating structural design that allows for their primary function: transporting oxygen from the lungs to various parts of the body and carrying carbon dioxide back for exhalation. This two-way street of gas exchange is vital for maintaining homeostasis and ensuring that the body's many systems operate smoothly.
Historical Overview
To appreciate the role of red blood cells today, it's often enlightening to take a step back in time and observe how our understanding has evolved. The historical quest to comprehend the nature of blood began centuries ago, with notable figures like William Harvey in the 17th century laying the groundwork for circulation theories. Yet, the distinct nature of red blood cells wasn’t clearly defined until advances in microscopy allowed scientists to view blood under magnification. In the late 1800s, scientists like Paul Ehrlich started to describe the shape and structure of RBCs among other blood components. The discovery of hemoglobin's role in oxygen transport came next, opening doors to various research areas such as blood diseases and transfusion science.
Function of Red Blood Cells
The primary function of red blood cells is nothing short of remarkable. Each RBC is packed with hemoglobin, a protein that binds oxygen and facilitates gas exchange. But that’s not the whole story. The design of these cells—biconcave in shape—maximizes surface area, improving oxygen absorption and delivery efficiency.
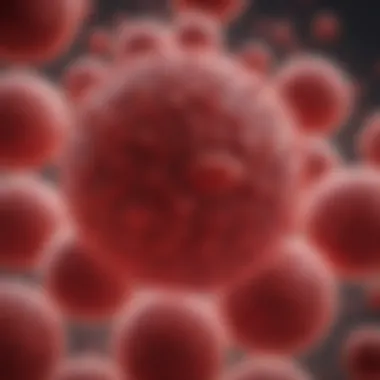
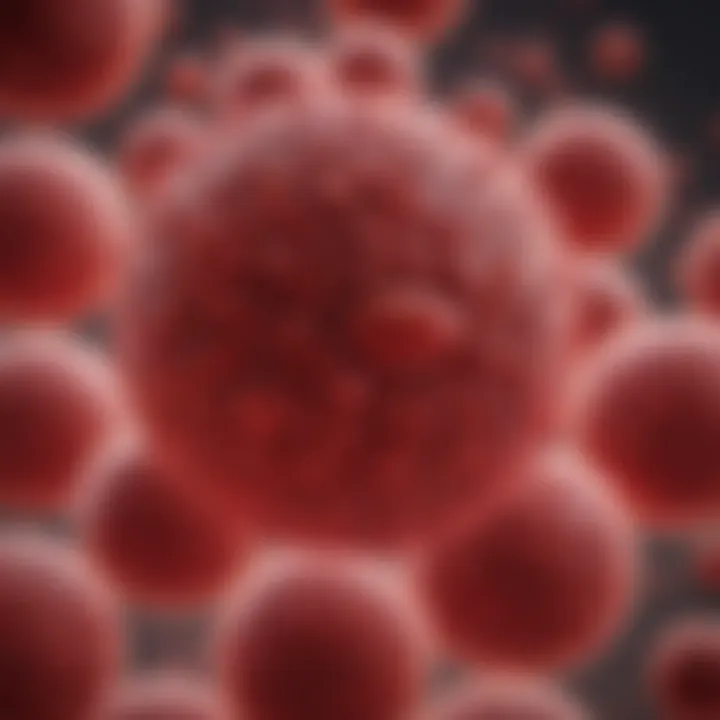
Furthermore, RBCs play a crucial role in maintaining the acid-base balance in the body. By binding to carbon dioxide and transporting it back to the lungs, they help regulate pH levels, which is essential for proper cellular function. Beyond this, the lifespan of an average RBC is around 120 days, after which they are recycled in the spleen and liver, ensuring a continuous supply of these essential cells.
The functionality of red blood cells exemplifies nature's engineering—simple yet profoundly effective in sustaining life.
In summary, understanding the historical context and primary functions of red blood cells sets the stage for deeper exploration into their structure and variability across species, as highlighted in the subsequent sections of this article.
Basic Structure of Red Blood Cells
Understanding the basic structure of red blood cells is crucial as it outlines how these cells operate within our bodies. This facet gives insight into their functionality, adaptability, and even their role in various health conditions. The composition not only impacts how effectively they transport oxygen but also showcases the incredible evolutionary adjustments that enable survival in different environments.
Cytoplasm Composition
Red blood cells, devoid of a nucleus, contain a unique cytoplasm made primarily of hemoglobin, the protein responsible for oxygen transport. The high concentration of hemoglobin gives these cells their distinct red color and enables them to perform their function effectively.
The cytoplasmic environment is also filled with enzymes and electrolytes that facilitate the transport process. Things like carbonic anhydrase play a vital role in the conversion of carbon dioxide to bicarbonate, assisting in efficient gas exchange. An interesting aspect is that while mature RBCs lack organelles, they carry out these essential metabolic activities to sustain energy levels and ensure functionality.
Cell Membrane Characteristics
The cell membrane of red blood cells is critical for maintaining structural integrity and facilitating the exchange of gases. This membrane is composed predominantly of a phospholipid bilayer, which is characterized by its selective permeability. This characteristic enables the cell to control what enters and exits, a necessity for maintaining homeostasis.
Phospholipid Bilayer
The phospholipid bilayer serves as the fundamental component of the RBC membrane, important for maintaining its shape and elasticity. One of the key features is that it allows for flexibility, which is crucial as red blood cells navigate through the narrowest capillaries in the body. This flexibility is pivotal, allowing the cells to deform without rupture, enhancing their ability to deliver oxygen effectively.
Moreover, the bilayer is hydrophobic on the interior, preventing the diffusion of water-soluble substances and contributing to the overall efficiency of gas exchange. This selective barrier creates a highly advantageous environment for the RBC, as it effectively transports oxygen while protecting vital processes from external fluctuations.
Membrane Protein Functions
The membrane proteins embedded within the phospholipid bilayer are not mere passengers; they play crucial roles in the functional dynamics of red blood cells. These proteins are responsible for various actions, including facilitating gas exchange, regulating cell shape, and providing structural support. Notably, proteins like band 3 contribute to anion exchange, helping in acid-base balance – critical for optimal physiological function.
The unique feature of these membrane proteins is that they are not static; they can shift and change position within the bilayer, which enhances the cell's responsiveness to environmental changes. Coupled with the phospholipid bilayer, these proteins form a dynamic interface that promotes effective cellular communication and functionality.
"The intricate balance between elastic structure and functional protein roles defines the efficiency of red blood cells in their vital task of oxygen transportation."
Hemoglobin and Oxygen Transport
Hemoglobin serves as the backbone of red blood cells (RBCs), playing a crucial role in the transport of oxygen throughout the body. This section outlines the intricate relationship between hemoglobin structure and its ability to ferry oxygen efficiently. Understanding hemoglobin and its function is vital for grasping how our bodies maintain oxygen levels, which is essential for all cellular processes.
Structure of Hemoglobin
Hemoglobin Subunits
Hemoglobin is composed of four protein subunits, each made from a polypeptide chain. These subunits are generally classified into two types: alpha and beta globins. In the adult human, two alpha chains and two beta chains come together, forming a unique structure called tetramer. This arrangement allows hemoglobin to bind four oxygen molecules simultaneously.
A pivotal characteristic of hemoglobin subunits is their cooperative binding nature. When one molecule of oxygen attaches to a hemoglobin subunit, the structure undergoes a conformational change, increasing the affinity for subsequent oxygen molecules. This feature is not just beneficial; it’s crucial for ensuring that oxygen is picked up in the lungs and delivered effectively to tissues that need it most.
One unique aspect of hemoglobin subunits is their role in not just oxygen binding but also in sensing carbon dioxide levels. This dual function aids in regulating blood pH and adjusting oxygen delivery based on metabolic needs. Though subunit composition may vary across species, its fundamental role remains unchanged, highlighting an evolutionary consistency vital for survival.
Heme Groups
Integral to hemoglobin's function are the heme groups, iron-containing organic compounds tucked within the hemoglobin structure. Each of the four subunits houses one heme group, which is vital in oxygen transport. The ability of iron to oxidize, thereby forming a strong bond with oxygen, is a critical characteristic. This not only allows for high-capacity oxygen transport but also plays a role in the release of oxygen in tissues due to varying oxygen pressures.
What sets heme groups apart is their unique ability to undergo reversible binding. This property gives hemoglobin the flexibility to release oxygen where it’s needed most in a dynamic manner, adjusting according to the physiological environment. Although beneficial, the reliance on iron also poses a risk, as deficiencies or excesses can negatively impact overall hemoglobin functionality. The careful maintenance of iron levels is thus crucial for optimal RBC performance.
Mechanism of Oxygen Binding
The mechanism of oxygen binding involves several biochemical interactions, chiefly among hemoglobin’s heme groups. When oxygen approaches a heme group, it binds to the iron atom, forming a coordinate bond. This initiates an allosteric effect across the hemoglobin molecule, influencing the affinity of the remaining heme groups for oxygen.
Another interesting aspect is the role of 2,3-bisphosphoglycerate (2,3-BPG) in this process. Increased levels of 2,3-BPG reduce hemoglobin’s oxygen affinity, facilitating oxygen release in actively metabolizing tissues. This adds a layer of sophistication to the oxygen delivery system, enabling the body to respond to varying demands based on activity levels.
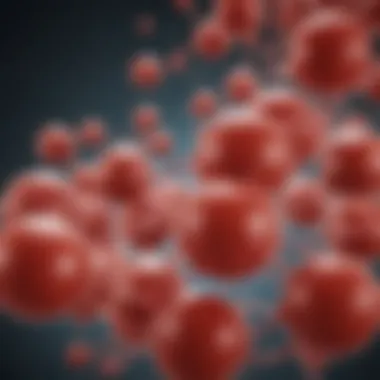
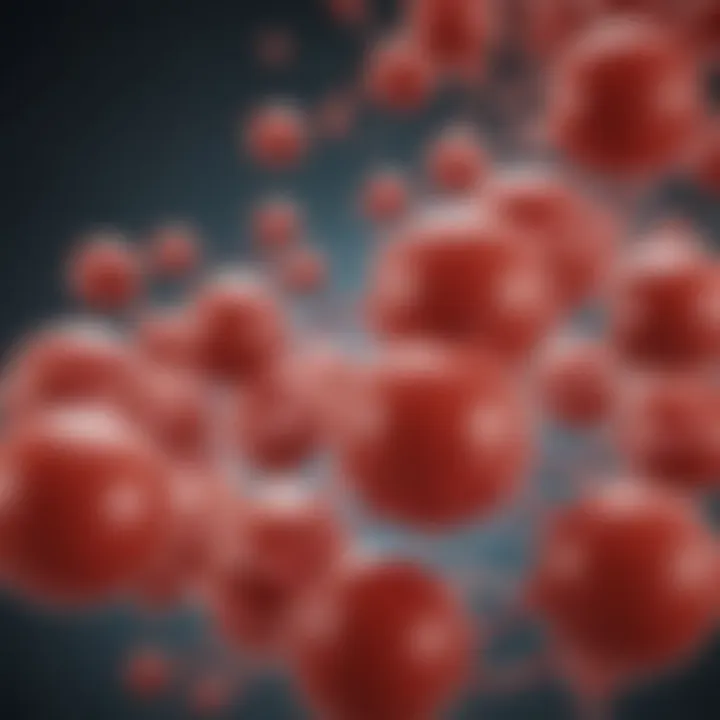
Understanding the fine nuances of how hemoglobin interacts with oxygen not just sheds light on basic biology, but also points to significant clinical implications. Conditions like anemia or chronic obstructive pulmonary disease can severely affect this transport mechanism, affirming the need for ongoing research and better treatment options for related ailments.
Understanding hemoglobin's role is crucial not only for academic pursuits but also for practical applications in medicine and health sciences.
Variability in Red Blood Cell Structure
The structure of red blood cells (RBCs) is not uniform across different species, making their variability a crucial area of study. Understanding these variations provides insights into how different organisms adapt their oxygen transport systems to diverse environments and physiological demands. Variability in RBC structure can influence their efficiency in transporting oxygen, survival in extreme conditions, and their role in various diseases. In essence, recognizing these differences helps in tailoring medical treatment and enhancing our knowledge of evolutionary biology.
Species Differences
Human vs. Animal RBCs
Human RBCs are quite remarkable in their biconcave shape, which increases their surface area, allowing for greater oxygen absorption. This specific structure is a key characteristic of the human red blood cell that contributes significantly to their functionality. In contrast, animal RBCs, such as those found in birds and reptiles, are nucleated and can vary greatly in size and shape.
- One of the most notable aspects of human RBCs is the absence of a nucleus. This deficiency allows more space for hemoglobin, thereby optimizing oxygen transport. However, the presence of nuclei in animal RBCs can be beneficial for certain regulatory functions.
- Unique features such as the size and shape variability in animal RBCs can provide advantages in adapting to different oxygen levels and environmental conditions.
This disparity emphasizes why understanding both human and animal RBC structures is essential; it informs medical research and treatment strategies tailored to specific species' needs.
"Variability in RBC structure enhances adaptability and overall physiological performance across different species."
Adaptations in Extreme Environments
Different organisms have evolved unique adaptations in their RBC structure that allow them to thrive in extreme environments. For instance, fish living in high-altitude lakes exhibit larger and more flexible red blood cells, enhancing their oxygen-carrying capacity under low-pressure conditions.
- A key characteristic of these RBC adaptations is their increased hemoglobin concentration. This trait is beneficial as it enables the fish to extract more oxygen from their environment, crucial for survival.
- Such adaptations also come with disadvantages; the larger cells can be more prone to rupture, although these species have other mechanisms to mitigate such risks.
These adaptations shed light on how RBCs evolve in response to environmental pressures, crucial for understanding the limits of life on Earth and might even suggest clues about extraterrestrial life potential.
Anomalies and Diseases
A focus on red blood cell structure would be remiss without addressing the anomalies that can arise, leading to various diseases.
Sickle Cell Disease
Sickle Cell Disease (SCD) is a prime example of how a single mutation in the hemoglobin gene can lead to significant structural changes in red blood cells. In SCD, RBCs become rigid and take on a peculiar crescent shape, which hinders their ability to navigate through small blood vessels.
- The unique feature of these sickle-shaped cells is their susceptibility to blockages in the circulatory system, leading to painful crises. In this way, SCD illustrates the importance of RBC shape on overall health and functionality.
- While this condition prevails as a disadvantage, some carriers of the sickle cell trait gain resistance to malaria, underscoring the complex interplay between genetic anomalies and environmental factors.
Thalassemia
Thalassemia, on the other hand, results from an imbalance in the production of hemoglobin chains, leading to ineffective red blood cell formation. This genetic disorder compromises the body's ability to produce enough healthy RBCs, causing symptoms like anemia and fatigue.
- Its defining characteristic, an unequal ratio of alpha and beta globin chains, makes it a pertinent topic in understanding hematological functions. This disorder also highlights the importance of genetic screening and early detection in patient management.
The variability inherent to red blood cell structure, from species adaptations to illness manifestations, provides a layered perspective on their role in biology, pushing the envelope of how we comprehend life, health, and medicine.
Cellular Aging and Lifespan
Understanding the aging and lifespan of red blood cells is crucial in grasping their role in human physiology. These cells, which transport oxygen and carbon dioxide, have a life cycle that affects the efficiency of our circulatory system. By delving into the intricacies of their cellular aging, we get a clearer picture of health conditions related to blood and how to optimize red blood cell functionality.
As red blood cells age, several processes come into play that can impact their performance and overall health. It is through the examination of these processes that we can understand the relationship between cellular aging and diseases such as anemia or polycythemia.
Erythropoiesis Process
Formation in Bone Marrow
The formation of red blood cells takes place within the bone marrow, which acts like a bustling factory of sorts for these essential units. This process is known as erythropoiesis. Bone marrow is uniquely suited for this task, as it provides a microenvironment filled with necessary nutrients and growth factors conducive to cell development.
A key characteristic of the formation in bone marrow is the presence of erythroid progenitor cells, which are specifically designed to develop into mature red blood cells. This specialization is a fundamental advantage, ensuring that the body can produce billions of new red blood cells each day to replace those that have perished.
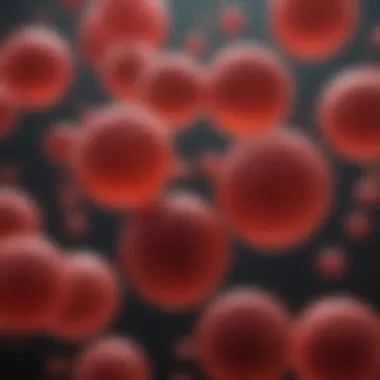
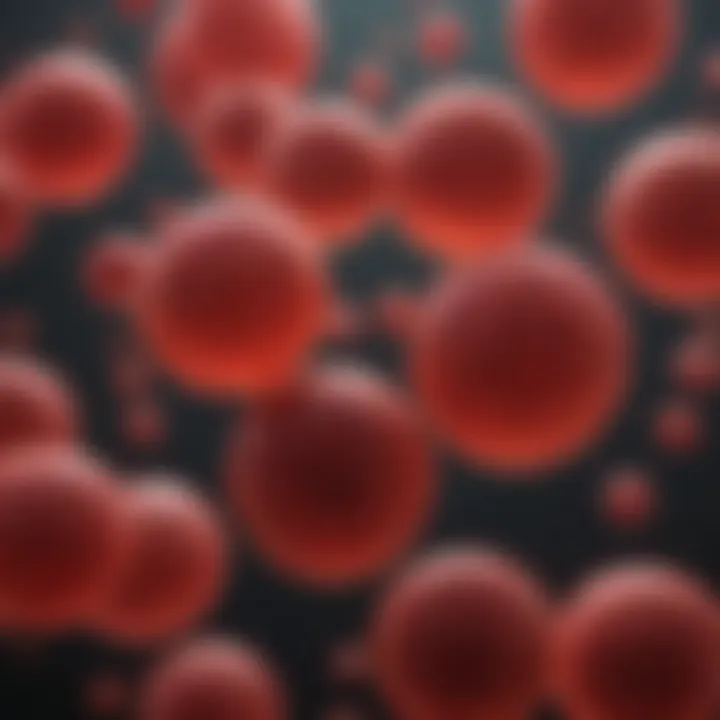
A unique feature of the erythropoiesis process in the bone marrow is its adaptability. For instance, in cases of increased demand for oxygen, such as with heavy exercise or high altitude living, erythropoiesis ramps up significantly. This responsiveness ensures that our tissues receive an adequate supply of oxygen, ultimately supporting various bodily functions.
However, if the bone marrow becomes compromised due to certain diseases or aging, its capacity to produce red blood cells diminishes, leading to potential health risks. That's why understanding this formation process is crucial within the context of red blood cell aging.
Release into Circulation
Once matured, red blood cells are released into the bloodstream where they embark on their vital role in gas transport. The release mechanism is a coordinated effort that ensures a steady flow of newly formed red blood cells entering circulation. These cells can survive up to about 120 days in the bloodstream before they begin their gradual decline into senescence.
A critical aspect of the release into circulation is the timing and quantity of red blood cells entering the bloodstream. Maintaining a balanced release is essential, as an excess can lead to conditions like polycythemia, while inadequate release can cause anemia.
An intriguing part of this process is how the body monitors oxygen levels and responds appropriately. When oxygen levels drop, the kidneys release erythropoietin, a hormone that stimulates the bone marrow to increase red blood cell output. This feedback mechanism underscores the elegance of the system in responding to varying demands.
However, if there are underlying health issues affecting this release, such as chronic diseases or bone marrow disorders, the benefits of red blood cell functionality can swiftly turn into challenges, affecting how efficiently oxygen is delivered to tissues.
Senescence Mechanisms
As red blood cells age, they undergo a variety of senescence mechanisms which significantly impact their state. One primary mechanism is the gradual deterioration of their membrane integrity. Over time, the structure of the cell membrane changes, becoming less flexible. This makes it difficult for aged red blood cells to navigate through capillaries, resulting in potential blockages that impede blood flow.
Another crucial aspect involves changes in enzymatic activity within the cells. As these cells age, essential enzymes used for repairing cellular components or dismantling damaged proteins decline in function. This decline can lead to a buildup of waste products within the red blood cells, negatively impacting their efficacy.
Red blood cells also undergo significant changes in metabolism as they age. The shift towards anaerobic respiration can leave aged cells with insufficient ATP production, compromising their functions.
In summary, understanding the mechanisms of cellular aging in red blood cells not only furthers our knowledge about hematology but also sheds light on various health implications associated with aging, making it a topic worth exploring in depth.
Current Research Trends
Current research trends in red blood cells (RBCs) reveal the ever-evolving landscape of hemoglobin studies and advancements in cellular manipulations. The exploration of RBC structure not only deepens our understanding of their biological roles but also underscores potential therapeutic innovations. Researchers continue to focus on how genetic tinkering can improve RBC functions. This, alongside the burgeoning field of artificial blood products, set the stage for future developments that could revolutionize transfusion medicine and treat blood-related disorders.
Genetic Engineering and RBCs
Genetic engineering is holding the spotlight in the study of red blood cells, aiming to tackle various blood disorders and enhance the functionality of these vital cells. The application of CRISPR technology has opened doors for precise modifications in the genetic codes of RBCs.
- Potential Applications:
- Considerations:
- Combatting inherited conditions, like sickle cell disease, by correcting the underlying genetic mutations.
- Enhancing the oxygen-carrying capacity of RBCs to create more efficient blood for patients.
- Ethical implications surrounding gene editing: it is crucial to navigate through the moral landscape of altering human genetics.
- Long-term consequences and stability of genetic modifications must be rigorously studied before clinical application.
Genetic engineering of RBCs represents a transformative approach in medicine that could lead to personalized treatments tailored to individuals’ genetic makeups. With ongoing trials and research, the hope is to not only treat but perhaps fully cure certain hemoglobinopathies, encouraging a new era in blood health.
Innovations in Artificial Blood Products
The demand for safe and sustainable blood alternatives has sparked innovation in artificial blood products. This is particularly relevant in situations where blood supply is limited or where patients have specific blood type needs.
- Types of Innovations:
- Benefits:
- Hemoglobin-Based Oxygen Carriers (HBOCs): These products utilize modified hemoglobin to mimic the oxygen transport capabilities of natural RBCs.
- Perfluorocarbons: While not blood, these compounds can transport oxygen and carbon dioxide and are being investigated for their potential to act as blood substitutes.
- Reduced dependency on human blood donations, which can fluctuate with demand.
- Increased shelf life and storage options compared to real blood, which often has a short usable window.
- Enhanced safety profile by eliminating risks of disease transmission associated with human blood products.
The journey of creating effective artificial blood is not without hurdles, however. Issues related to biocompatibility and immune responses need thorough investigation to avoid adverse reactions post-administration. As researchers push forward, the aim remains to create a product that can seamlessly integrate into human biology, ensuring safety and efficacy in critical medical situations.
"The ongoing development of artificial blood products promises a brighter future for transfusion medicine, potentially saving countless lives with reliable and safe alternatives to traditional blood."
In summary, the current trends in genetic engineering and innovations in artificial products highlight the extraordinary potential within red blood cell research. These advances are not merely incremental; they could reshape the very foundation of clinical practices surrounding blood transfusions and treatments for blood disorders.
End
The significance of the structure and function of red blood cells (RBCs) cannot be overstated. These remarkable cells are not merely a component of blood; they fulfill pivotal roles in sustaining life across various biological systems. As we’ve delved into different aspects of RBCs in this article, it’s clear that their intricate structure—from the unique shape to the efficient transport mechanism of hemoglobin—plays an essential role in their functionality.
To recap, the key points touching upon the importance of RBCs include:
- Oxygen Transport: The design of RBCs, notably their biconcave shape, maximizes surface area for the efficient absorption and delivery of oxygen. This architecture ensures that oxygen reaches every cell in the body.
- Species Variability: Different organisms exhibit variations in RBC structure, with adaptations that suit their environments and lifestyles. Understanding these differences informs researchers about evolutionary biology and allows for better comparative studies.
- Implications of Anomalies: Structural disorders such as sickle cell disease and thalassemia underscore the delicate balance required in RBC function. Studying these conditions highlights the need for innovation in treatments and further research.
- Current Research: As we look towards the future, advancements in genetic engineering and artificial blood products could revolutionize the field of hematology. These innovations could lead to improved therapies for blood-related diseases and enhancements in transfusion medicine.
"Understanding the structure of red blood cells is critical to grasping broader physiological processes and developing innovative medical solutions."
Thus, appreciating the complex nuances of red blood cells enriches our comprehension of human physiology and the interconnections that bind us to countless other species. This exploration isn’t just academic; it reflects on the future of medicine, genetics, and evolutionary studies. As research continues to evolve, hoping to uncover the myriad secrets these cells hold, we find ourselves on the brink of a deeper understanding of life itself.