Transforming Carbon Dioxide into Sustainable Fuels
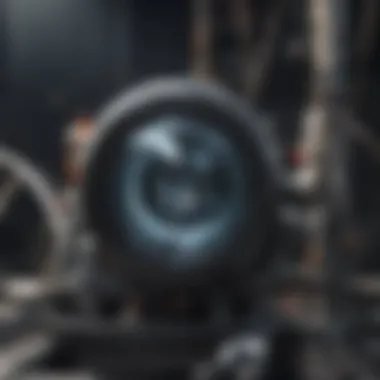
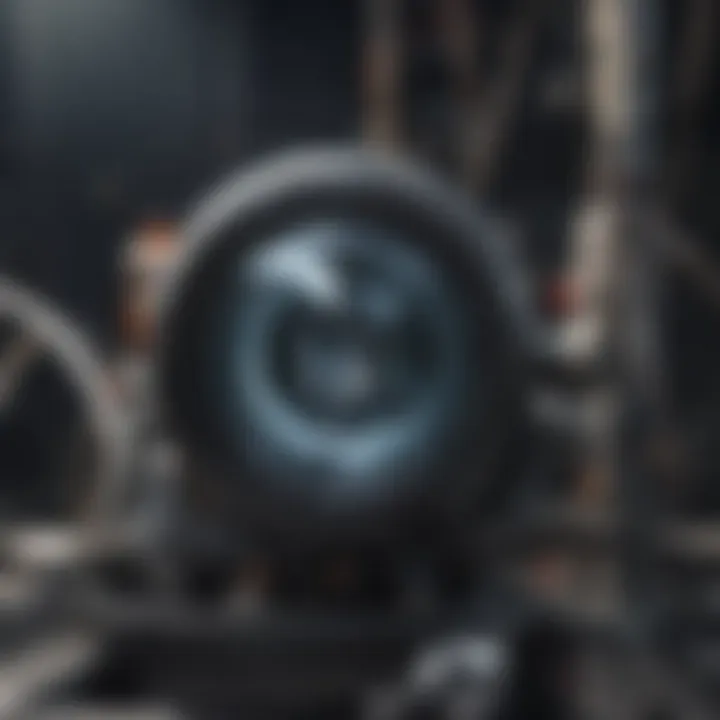
Intro
The ongoing global discourse on climate change presses for more sustainable energy solutions. Amid this dialogue, the transformation of carbon dioxide into usable fuel stands out. This process not only aims to mitigate CO2 emissions but also addresses the pressing need for alternative energy sources. The convergence of scientific research and technological methodologies paves the pathway for this transformation. This section provides an overview of the key elements involved in converting CO2 into fuel, emphasizing both the scientific rigor and practical applicability of contemporary methods.
Research Overview
Methodological Approaches
Various methodologies exist for the conversion of carbon dioxide. These approaches can be broadly categorized into chemical and biochemical processes.
- Chemical Conversion: This method typically employs catalysts to facilitate the reaction between CO2 and other substances to yield hydrocarbon fuels. Common techniques include the Sabatier reaction, which employs nickel or ruthenium catalysts to synthesize methane. Furthermore, electrochemical reduction of carbon dioxide is gaining traction, especially with advancements in electrolysis techniques.
- Biochemical Conversion: This process utilizes microorganisms to convert carbon dioxide into fuel. For instance, certain algae can metabolize CO2 and produce lipids, which can be extracted and processed into biodiesel. Such methods capitalize on biological systems to offer a sustainable fuel alternative.
The significance of these approaches cannot be overstated. They confirm the potential of carbon dioxide not merely as a waste product but as a valuable resource in our quest for sustainable energy solutions.
Significance and Implications
The relevance of converting CO2 into fuel extends beyond energy production. There are several important implications:
- Environmental Benefits: By reducing atmospheric CO2, these methods can contribute significantly to climate change mitigation.
- Energy Security: Utilizing CO2 boosts energy independence and reduces reliance on fossil fuels.
- Economic Opportunities: The development of CO2 conversion technologies can create jobs and stimulate economic growth in new sectors.
"Transforming CO2 into fuel positions itself as a linchpin in the synthesis of sustainable energy systems, bridging environmental responsibility with economic viability."
Current Trends in Science
Innovative Techniques and Tools
Ongoing research reveals numerous innovative techniques that enhance the efficiency and feasibility of CO2 conversion. Researchers are exploring advanced catalysts that increase reaction rates and reduce energy input costs.
New tools, such as artificial intelligence, aid in optimizing reaction conditions. Machine learning algorithms can analyze vast datasets to assess which combinations of materials yield the best results, pushing the boundaries of known methods.
Interdisciplinary Connections
The conversion of carbon dioxide into fuel is not restricted to the realm of chemical engineering. It intertwines with various disciplines, including:
- Material Science: Ongoing development of nanomaterials for better catalysts.
- Environmental Science: Understanding the ramifications of large-scale CO2 conversion on ecosystems.
- Economics: Studying the cost-benefit analyses of implementing these technologies across various sectors.
This cross-disciplinary dialogue enriches the research landscape and promotes a holistic understanding of various implications surrounding CO2 to fuel conversion.
Preamble to Carbon Dioxide Utilization
Carbon dioxide (CO2) has become a focal point in the discussions about climate change and energy sustainability. Its role in our atmosphere is complex. On one hand, CO2 is a significant greenhouse gas, contributing to global warming. On the other hand, innovators and scientists have begun to see it as a resource rather than just a pollutant. Utilizing CO2 as a feedstock for fuel production can potentially transform a problematic waste into valuable energy, highlighting both an environmental solution and an energy opportunity.
The Role of CO2 in Climate Change
Carbon dioxide is a natural part of Earth’s atmosphere. However, human activities, such as burning fossil fuels and deforestation, have dramatically increased its levels. This rise in CO2 is a major driver of climate change. As a greenhouse gas, it traps heat in the atmosphere and contributes to rising temperatures, altered weather patterns, and other detrimental environmental impacts. Understanding this relationship is crucial because it shapes the motivation behind CO2 utilization strategies. Reducing atmospheric CO2 through innovative methods could mitigate climate impacts while offering new economic avenues.
Importance of Carbon Capture
Before transforming CO2 into fuel, effective carbon capture is essential. Carbon capture technologies aim to intercept CO2 emissions emitted from various sources like power plants and industrial processes. Capturing CO2 not only helps reduce the overall concentration of greenhouse gases but also provides the raw material for fuel production. This integration can create a closed-loop system, where CO2 emissions are minimized and converted into sustainable fuels, effectively reducing the carbon footprint. The importance of carbon capture lies in its dual ability to address global warming and repurpose emissions into useful products. This highlights a proactive step in combating climate change.
Overview of Fuel Production from CO2
The production of fuel from carbon dioxide (CO2) is an endeavor of paramount importance in today’s energy landscape. As global energy demands rise and concerns about climate change intensify, exploring alternative fuel sources becomes an urgent priority. CO2 utilization presents a dual advantage: it helps mitigate greenhouse gas emissions while providing pathways to sustainable fuel solutions. In this section, we will examine the significance and implications of CO2-based fuel production, addressing its potential benefits and the key considerations involved.
The production of CO2-based fuels can significantly contribute to a circular economy, where waste materials are reused, minimizing the need for fossil fuels. This approach not only reduces carbon footprint but also enhances energy security by diversifying energy sources. Moreover, CO2-derived fuels can often be produced using existing infrastructure, making their implementation potentially more feasible and cost-effective than completely new systems.
However, the transition to fuels generated from CO2 involves several key challenges. It requires advancements in technology, sufficient investment, and supportive policies to encourage industrial uptake of these processes. Understanding the science behind CO2 conversion is essential for developing realistic strategies to optimize yield efficiencies and reduce overall costs. Now, we turn our focus to specific definitions and historical context surrounding this topic.
Definition of CO2-Based Fuels
CO2-based fuels can be described as energy carriers that are synthesized from carbon dioxide, often in combination with hydrogen derived from renewable sources. These fuels can take various forms, including synthetic natural gas, methanol, and hydrocarbons suitable for conventional engines. The chemical processes involved in creating these fuels typically aim to mimic those found in traditional fossil fuel formation, but with the crucial difference that CO2 is utilized as a primary feedstock instead of extracted from the Earth.
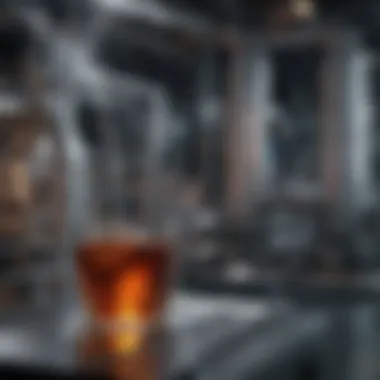
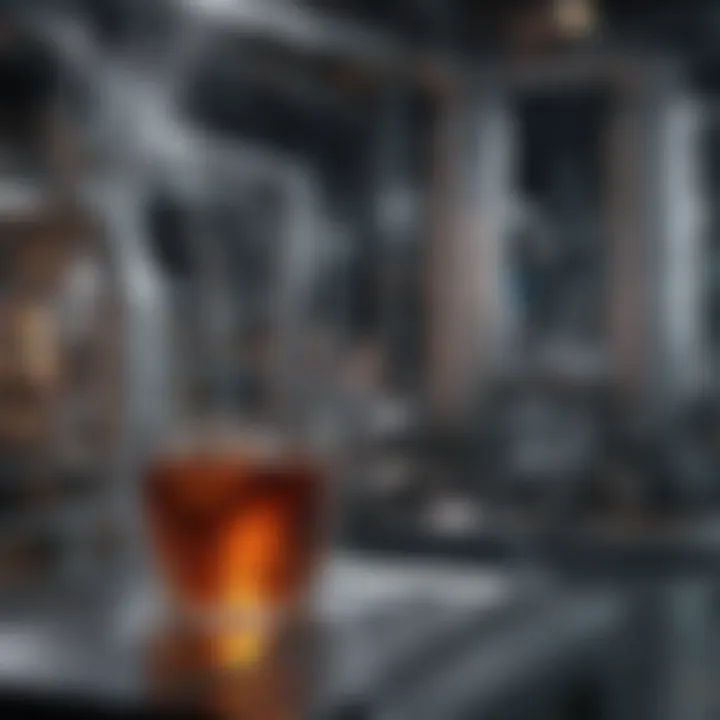
The use of CO2 for fuel production is not only innovative but also a vital component in addressing the excess CO2 in the atmosphere. Harnessing this greenhouse gas can assist in slowing the pace of climate change by repurposing it in useful ways, rather than allowing it to accumulate in the environment.
Historical Context of CO2 to Fuel Conversion
The journey of converting CO2 into usable fuels has its roots in the early 20th century when scientists first explored the reactions involved in carbon fixation. Early methods aimed to utilize CO2 for producing hydrocarbons focused on chemical processes like the Fischer-Tropsch synthesis, which converts carbon monoxide and hydrogen into liquid hydrocarbons.
Over time, advancement in catalytic processes and increased understanding of electrochemical reduction have created new opportunities for CO2 conversion. In the last couple of decades, there has been a growing recognition of the potential for CO2 utilization driven by the heightened discourse on climate change, leading to significant investments in research.
Notably, the commercialization of CO2 conversion technologies has been slow, but various pilot projects have laid the groundwork for larger initiatives. As awareness and scientific knowledge expand, so too does the potential for incorporating CO2-derived fuels into the global energy mix. This historical context sets the stage for exciting developments in the field of alternative fuels as we continue to innovate in the search for sustainable energy solutions.
Technological Methods of CO2 Conversion
The conversion of carbon dioxide into usable fuels is a multifaceted challenge that demands innovative technological solutions. Various methods are being developed to effectively transform CO2 into energy carriers, addressing both climate change and energy diversification. These technological methods can be broadly categorized into chemical and biochemical solutions. Each category presents its own unique benefits and considerations, thereby shaping the future of sustainable fuel production.
Chemical Conversion Techniques
Chemical conversion techniques play a critical role in the transformation of CO2 into fuels. They encompass a variety of processes designed to facilitate the direct conversion of carbon dioxide into chemical energy. Two notable subcategories are electrochemical reduction and thermochemical processes.
Electrochemical Reduction
Electrochemical reduction involves using electrical energy to convert CO2 directly into hydrocarbons or alcohols. This technique is characterized by its potential for high conversion efficiencies, which make it appealing for practical applications. One key characteristic of electrochemical reduction is its ability to operate at ambient temperatures and pressures. This property makes it a viable option for integration with renewable energy sources, such as solar and wind power.
The unique feature of electrochemical reduction is its flexibility in producing various liquid fuels, like methanol and ethanol. These fuels can be readily utilized in existing infrastructure, providing a significant advantage. However, challenges remain. The efficiency of electrochemical reactors can be affected by factors like electrode material and electrolyte composition. Improved materials and designs are needed to enhance overall performance and lower costs.
Thermochemical Processes
Thermochemical processes utilize high temperatures to drive the conversion of CO2 into fuels. Typically, these processes involve the reaction of carbon dioxide with hydrogen derived from water splitting or methane. A significant advantage of thermochemical methods is their ability to produce hydrogen-rich gases, which can be further processed into fuels.
One key characteristic of thermochemical processes is their scalability. They can be designed to accommodate large-scale operations, making them suitable for industrial applications. A unique feature of this method is its potential for integrating carbon capture with fuel production. As a result, it can contribute to both CO2 reduction and energy generation efforts. However, these processes often require substantial energy input, leading to questions about overall efficiency. Additionally, the availability of thermal energy and the need for advanced reactor designs pose challenges that must be addressed.
Biochemical Methods
Biochemical methods include leveraging biological systems to convert CO2 into energy-rich fuels. These processes often utilize microorganisms or enzymes to facilitate conversion, offering promising pathways due to their inherent sustainability.
Microbial Fuel Cells
Microbial fuel cells are systems where microorganisms convert organic matter into electrical energy, including CO2 as a terminal electron acceptor. The key characteristic of microbial fuel cells lies in their biological basis, allowing for low-energy input and sustainable operation. They are a beneficial choice as they not only purify wastewater but also generate energy.
A unique feature of microbial fuel cells is their dual functionality—waste management and energy production. They can be an effective solution in treating waste while simultaneously generating useful energy. However, the scalability of microbial fuel cells remains a concern, often limited by the speed of microorganism growth and overall energy output.
Enzymatic Processes
Enzymatic processes leverage specific enzymes to catalyze reactions that convert CO2 into fuels. This technique is characterized by its high specificity and efficiency, often operating under mild conditions.
The unique aspect of enzymatic processes is the potential for targeted production. By utilizing tailored enzymes, it’s possible to produce specific desired fuels, which can lead to enhanced economic viability. Despite these advantages, enzymatic processes face challenges related to enzyme stability and recovery over time, which can affect long-term viability and costs.
"Advancements in both chemical and biochemical methods present unique opportunities not only for fuel production but also for active climate strategies."
Catalysts in CO2 Conversion
Catalysts play a crucial role in the conversion of carbon dioxide into fuels, enhancing the efficiency of the biochemical and chemical processes involved. The effectiveness of these catalysts can have significant implications for energy production and climate change mitigation. Their function is to lower the activation energy needed for these reactions, thus increasing the reaction rates without being consumed in the process. This property makes them essential in making CO2 conversion technologies economically viable.
Types of Catalysts Used
Homogeneous Catalysts
Homogeneous catalysts are defined as catalysts that exist in the same phase as the reactants. They are typically dissolved in the same solvent, facilitating easier mixing and interaction with CO2 and other reactants. One of the key characteristics of homogeneous catalysts is their ability to provide specific active sites for chemical reactions to occur more efficiently. This specificity often leads to higher selectivity and greater yields of desired products, making them a popular choice in various CO2 conversion processes.
The unique feature of homogeneous catalysts lies in their molecular nature, which allows for fine-tuning of their properties, such as reactivity and selectivity. However, they also come with disadvantages, such as challenges in separation from the reaction mixture after the process is completed. This separation can complicate the recycling process, making the overall system less sustainable.
Heterogeneous Catalysts
Heterogeneous catalysts, on the other hand, are in a different phase than the reactants, typically solid while the reactants are gases or liquids. This feature is advantageous because it allows for easier separation and recovery of the catalyst after the reaction completes. One key characteristic of heterogeneous catalysts is their large surface area, which offers many sites for CO2 to interact with the catalyst, ultimately leading to greater efficiency in fuel production.
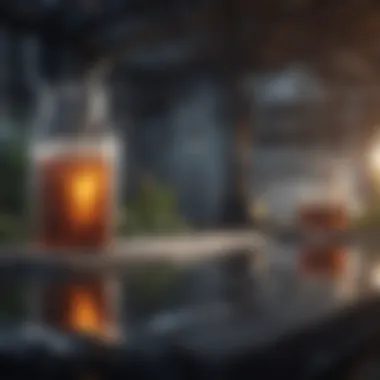
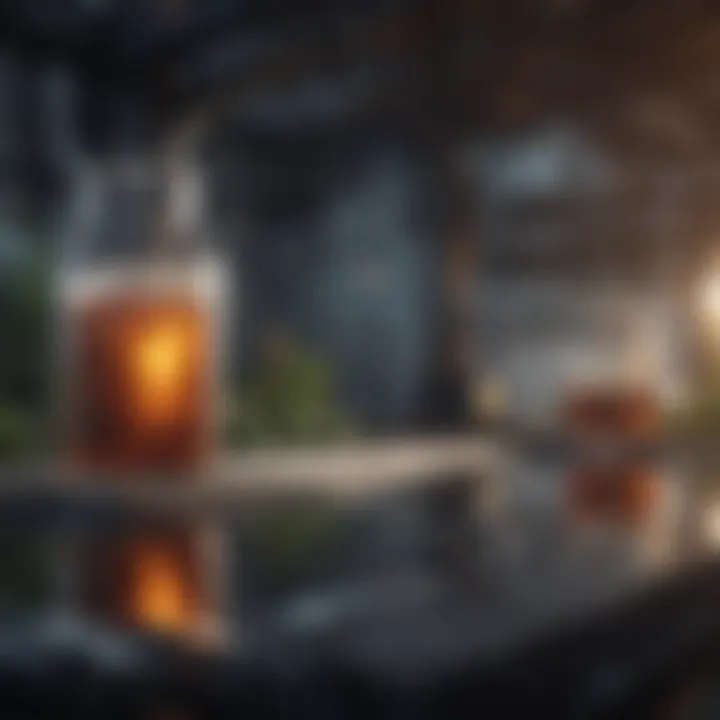
A primary advantage of using heterogeneous catalysts includes their durability and stability during reactions, which makes them suitable for large-scale industrial applications. However, they may also have limitations regarding the reactivity and selectivity of some reactions compared to homogeneous catalysts. Their design often requires optimization to maximize performance, which can be a challenging aspect in the development of CO2 conversion processes.
Role of Nanotechnology
Nanotechnology has emerged as a vital component in enhancing the performance of catalysts for CO2 conversion. The utilization of nanoscale materials can provide unique properties, such as increased surface area and improved catalytic activity. By engineering catalysts at the nanoscale level, researchers can increase their effectiveness in facilitating chemical reactions.
Moreover, nanotechnology enables the development of catalysts with specialized functions, such as photocatalysts that harness solar energy for CO2 reduction. The integration of these advanced materials into CO2 conversion processes could yield more efficient and sustainable fuel production pathways.
Current Research Trends
Current research trends in the utilization of carbon dioxide (CO2) are essential as they guide the advancement of technologies for sustainable fuel production. Targeting both climate change mitigation and energy security, these trends reveal innovative strategies to transform CO2 into valuable energy sources. Understanding these trends helps to monitor progress made in this area and indicates where future efforts should be focused. The benefits of ongoing research include improved efficiency, cost reduction, and minimizing environmental impacts.
Innovative Approaches in CO2 Utilization
Artificial Photosynthesis
Artificial photosynthesis represents a significant development in the quest to convert CO2 into fuels. This approach mimics the natural process of photosynthesis used by plants, where light energy is harnessed to create energy-dense compounds. A key characteristic of artificial photosynthesis is its potential to use sunlight directly, providing an environmentally friendly method of fuel production.
This technique's unique feature lies in its ability to produce oxygen and energy simultaneously, which is beneficial for maintaining ecological balance. Advantages of artificial photosynthesis include high energy efficiency and the capability to store energy in chemical bonds for later use. However, challenges remain, particularly concerning scalability and the need for robust materials that can withstand varying environmental conditions.
Direct Air Capture Technologies
Direct Air Capture (DAC) technologies focus on extracting CO2 directly from the atmosphere, which can then be converted into fuels or stored. This method plays a crucial role in reducing atmospheric CO2 levels, contributing to climate change mitigation. A key characteristic of DAC technologies is their versatility, as they can be deployed at varying scales, from small installations to large industrial operations.
The unique feature of DAC lies in its ability to directly address excess atmospheric CO2. While the advantages of DAC include enhanced carbon management and the possibility of circular carbon economies, there are also disadvantages. These include high energy demands for operation, significant implementation costs, and potential technological challenges associated with large-scale deployment.
Collaboration between Academia and Industry
Collaboration between academia and industry serves as a driving force in CO2 conversion research. Such partnerships facilitate the exchange of knowledge, resources, and expertise essential for innovation. By uniting theoretical understanding with practical application, these collaborations yield advancements that can lead to more efficient conversion methods and technologies.
Moreover, industry involvement ensures that research is aligned with market needs, promoting the development of commercially viable solutions. This synergy can accelerate the transition from laboratory success to real-world deployment, making it a vital aspect of progress in this field. Establishing networks and collaborative projects fosters an environment conducive to innovation and rapid advancement, essential for meeting global energy demands.
Applications of CO2-derived Fuels
The conversion of carbon dioxide into usable fuels presents a pivotal opportunity in the quest for sustainable energy solutions. CO2-derived fuels are designed to mitigate climate change while addressing the growing energy needs of our society. Their applications are varied and significant, ranging from transportation to power generation, and even chemical manufacturing. By highlighting these applications, we can appreciate the potential that these fuels hold in reshaping our energy landscape.
Transportation Sector
The transportation sector is one of the largest contributors to carbon emissions. Utilizing CO2-derived fuels like methanol or synthetic gasoline can play a vital role in reducing this impact. These fuels can be blended with traditional fossil fuels or used in modified engines designed for optimal performance. For instance, carbon-neutral methanol can be utilized in vehicles without extensive alterations or infrastructure changes.
A few benefits of implementing CO2-derived fuels in transportation include:
- Lower emissions: Using these fuels can significantly decrease greenhouse gases compared to conventional fuels.
- Energy security: By generating fuels from CO2, dependence on fossil fuels may lessen, contributing to a more secure energy future.
- Integration with existing systems: This strategy allows for the use of current vehicle technologies and fuel distribution systems, making the transition smoother.
Chemical Manufacturing
Chemical manufacturing relies heavily on fossil fuels as feedstocks. The development of CO2-derived equivalent feedstocks, such as urea or various hydrocarbons, enables the industry to shift towards more sustainable practices. Using CO2 as a feedstock can lead to the production of valuable chemicals while simultaneously reducing carbon emissions.
The potential applications within this sector include:
- Production of polymers: CO2 can be converted into polymers used in various products, from plastics to packaging materials.
- Synthetic fuels: Chemical manufacturing would enable the production of synthetic fuels, thus facilitating a circular carbon economy.
- Broader applications: The chemicals derived from CO2 can have applications in agriculture, energy storage, and pharmaceuticals.
Power Generation
Power generation from CO2-derived fuels presents an innovative approach to reducing emissions while still meeting energy demands. Using CO2-based fuels in power plants, especially those adapted for dual-fuel systems, can significantly cut harmful emissions. By incorporating CO2-derived outputs into energy generation, power plants can mitigate their operational footprint.
The benefits of CO2-derived fuels in power generation include:
- Carbon neutrality: Power generation that utilizes CO2-derived fuels can become closer to carbon-neutral over time as the emissions offset the CO2 used in the fuel-making process.
- Flexibility in energy sources: CO2-derived fuels enable energy diversification, offering new avenues for sustainable energy production, particularly in conjunction with renewable sources.
- Job creation: The investment in CO2-derived fuel technologies could lead to new job opportunities across various sectors, especially in research and development.
"Transforming CO2 into fuel is not just about energy production; it's about redefining our relationship with carbon and our environment."
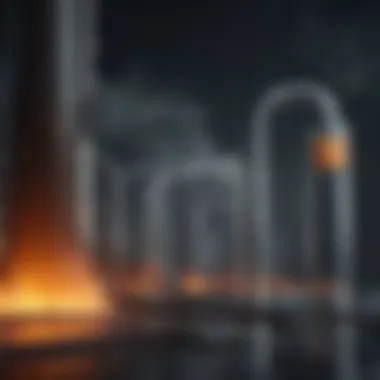
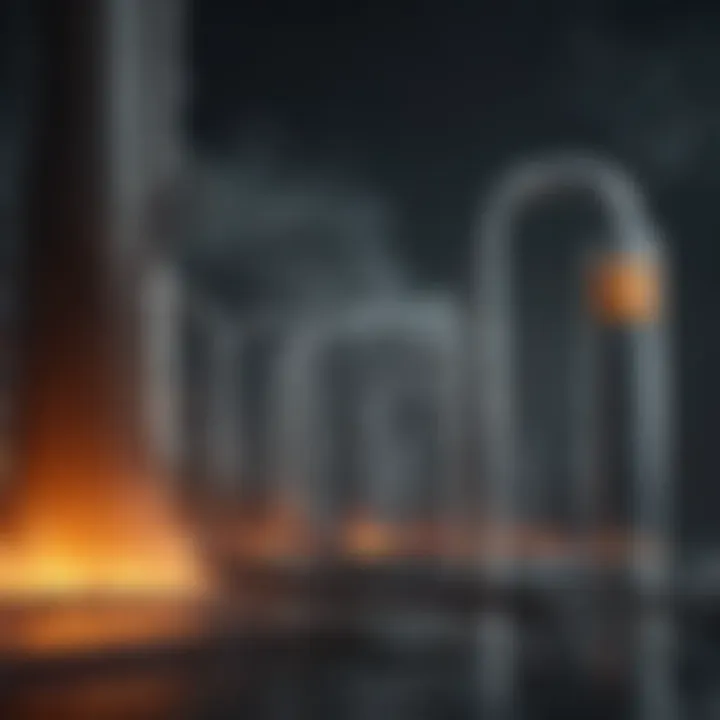
Challenges in CO2 to Fuel Technologies
The transformation of carbon dioxide into usable fuel presents a range of challenges that must be addressed if these technologies are to fulfill their potential. Economic viability, technical barriers, and regulatory complexities all play critical roles in determining the feasibility and scalability of CO2 conversion processes. Understanding these challenges is essential for guiding future research and development efforts, ensuring that the move towards sustainable fuel solutions can be realized.
Economic Viability
Economic factors dictate whether a technology can be adopted on a large scale. The costs associated with capturing, converting, and utilizing CO2 can be significant. Currently, many CO2 conversion processes are not yet competitive with traditional fossil fuels. Investing in new technologies often carries risk, especially if the market for CO2-derived fuels is not well-established.
Several strategies can improve the economic viability of these technologies:
- Scaling Up Production: Larger scale operations can reduce per-unit costs through economies of scale.
- Government Incentives: Financial support from government agencies can help alleviate initial costs.
- Research and Development: Ongoing innovation can lead to more efficient processes that lower costs.
Technical Barriers
Besides economics, technical barriers represent a significant challenge in converting CO2 into fuel. The efficiencies of current technologies often do not meet the demands for large-scale fuel production. Key technical challenges include:
- Energy Input Requirements: Many conversion processes require substantial amounts of energy, which diminishes the net environmental benefits.
- Selectivity of Catalysts: Achieving high selectivity in chemical reactions is crucial. Many catalysts can react with compounds other than CO2, leading to byproducts and lower yields.
- Process Integration: Integrating CO2 conversion systems into existing infrastructure often presents challenges, as compatibility needs to be established across various industrial processes.
Regulatory and Policy Issues
The regulatory environment surrounding CO2 conversion technologies adds yet another layer of complexity. Policymakers must create frameworks that encourage the development and deployment of these technologies while ensuring safety and transparency. Important considerations include:
- Standardization: Establishing common standards for CO2-derived fuels will aid in market acceptance and regulatory compliance.
- Carbon Credits and Taxation: Policies that reward carbon capture and utilization can stimulate investments in this area.
- Public Perception: Policy must address potential public concerns about safety and environmental impacts.
Understanding the economic, technical, and regulatory challenges is crucial for the sustainable advancement of CO2-to-fuel technologies.
Future Perspectives
The future of CO2 utilization in fuel production is integral to addressing the dual challenges of climate change and the urgent demand for sustainable energy sources. As global energy needs continue to rise, the quest for innovative pathways to convert carbon dioxide into usable fuels becomes increasingly relevant. The potential for technological advancement, coupled with ongoing research, suggests a transformative shift in how energy is produced and consumed in the coming decades.
Emerging Technologies
Emerging technologies in the conversion of CO2 to fuel present a spectrum of possibilities. One promising area is the development of advanced catalysts, which improve the efficiency and effectiveness of chemical reactions involved in CO2 conversion. Researchers are exploring metal-organic frameworks and nanostructured catalysts that provide higher selectivity and lower energy requirements.
Another significant advancement is the integration of artificial photosynthesis systems. These systems aim to mimic natural processes, using sunlight to drive the transformation of CO2 into hydrocarbons. Such innovations could drastically lower costs and increase productivity in harnessing CO2 for fuel generation.
Additionally, the use of biotechnology offers new avenues for CO2 utilization. Methods employing microalgae and other microorganisms can convert CO2 through biological processes into biofuels. These methods not only recycle CO2 but also contribute to biodiversity and ecosystem health.
Predicted Impact on Energy Landscape
The potential impact of CO2-derived fuels on the energy landscape is significant. With the incorporation of advanced CO2 conversion technology, the energy sector could shift towards a more circular economy. This transition could lead to a reduction in reliance on fossil fuels, decreasing overall carbon emissions.
Furthermore, as CO2 fuels gain traction, they could foster a new industry within the renewable energy sector. Job creation in research, development, and manufacturing processes would be likely, thus contributing to economic growth.
It's also crucial to consider regulatory frameworks and public acceptance as these technologies develop. Policies supporting research and investment in CO2 utilization will play a critical role in determining how swiftly and effectively these innovations are adopted.
The future belongs to those who aim to innovate in carbon capture and utilization, reshaping the face of energy production for sustainability.
Advancements in CO2 utilization technologies are not just beneficial; they are essential for meeting global climate targets. By recognizing the potential shifts in the energy landscape, we can advocate for stronger policies and investments that ensure a cleaner, more sustainable future.
Concluding Remarks
In the exploration of carbon dioxide conversion to fuel, the relevance of the findings cannot be overstated. This process stands as a beacon of hope against the backdrop of climate change and escalating energy demands. Through various methodologies such as chemical and biochemical techniques, significant strides have been made toward making CO2 a viable resource for fuel production. The knowledge synthesized in this article demonstrates how transforming CO2 is not merely an option but a necessity for sustainable energy practices.
Summary of Findings
The essential takeaway from this article is the multifaceted approach needed to make CO2 conversion to fuel a reality. Key findings include:
- Innovative chemical and biochemical methods have shown promising results in converting carbon dioxide into usable fuels.
- Emerging technologies such as artificial photosynthesis highlight the potential for large-scale applications.
- Collaboration between industry and academia is crucial in overcoming technical and economic barriers.
- Ongoing research is vital for refining processes and enhancing efficiency in CO2 utilization.
Overall, the findings emphasize the critical nature of addressing both environmental and energy challenges through CO2 transformation.
Call to Action for Research and Development
The pathway to successful CO2 conversion into fuel is still under construction. Therefore, a strong call to action is needed for all stakeholders involved:
- Invest in research that focuses on new catalytic processes and improved technological approaches.
- Encourage partnerships between universities and companies to foster innovation.
- Support government policies that facilitate funding and resources for sustainable energy projects.
As highlighted throughout this article, the potential to utilize carbon dioxide as a primary feedstock for fuel production is immense. Addressing the challenges requires collaborative efforts, innovative thinking, and substantial investment to translate these promising methodologies into scalable solutions. The efforts made today can significantly impact the future of energy sustainability, making CO2 a resource rather than a waste.