Understanding ATP Energy: Cellular Activities Unveiled
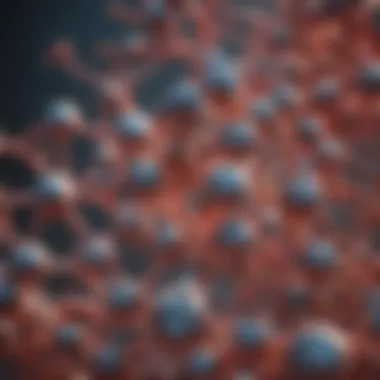
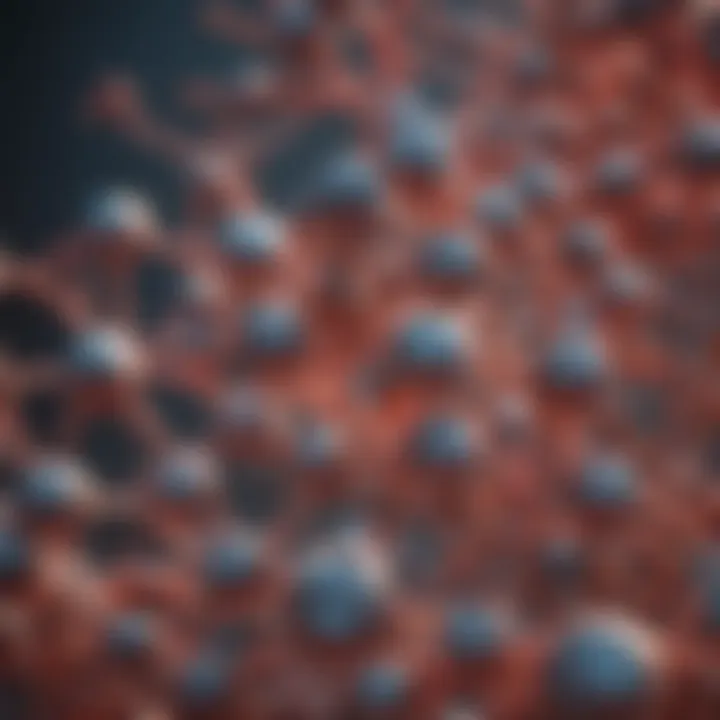
Intro
Adenosine triphosphate, commonly known as ATP, is essential for life. It is often called the energy currency of the cell. This molecule plays a central role in various biochemical processes. Understanding how ATP functions reveals insights into cellular mechanics. The importance of ATP extends beyond basic biology. It intersects with medical research and biotechnology. This article explores the intricate nature of ATP, its synthesis, and its function in cellular activities.
Research Overview
Methodological Approaches
Research into ATP often employs diverse methodologies. Scientists use techniques such as chromatography and spectrometry to analyze ATP concentrations in cells. These methods enable researchers to assess ATP levels under different conditions. Additionally, experiments in molecular biology help to elucidate how ATP interacts with various proteins. The study of both in vitro and in vivo systems is essential to understand ATP's role in cellular processes. One common technique is the use of bioluminescence assays. This allows for real-time measurement of ATP in biological samples.
Significance and Implications
The significance of ATP researchcannot be overstated. It has broad implications for understanding diseases and health. For example, ATP depletion is linked with conditions such as heart disease and neurodegenerative disorders. Furthermore, therapies that target ATP synthesis or degradation can have profound effects.
Research on ATP illuminates fundamental principles of biochemistry. It also has practical applications in medicine and industrial processes. Understanding ATP’s function can lead to advances in treatments or new technologies.
"ATP is not just a molecule; it is a pivot around which many cellular processes revolve."
Current Trends in Science
Innovative Techniques and Tools
Recent advancements in technology enhance ATP research capabilities. High-resolution imaging techniques allow scientists to visualize ATP dynamics in real-time. Tools like mass spectrometry have improved the sensitivity of ATP detection. These innovations contribute to a deeper understanding of ATP's role in metabolic pathways and cellular signaling.
Interdisciplinary Connections
The study of ATP is not confined to biochemistry. It bridges disciplines such as molecular biology, pharmacology, and bioengineering. Collaborative efforts between these fields lead to new discoveries in cellular energy dynamics. This interdisciplinary approach fosters innovations such as targeted drug delivery systems, which optimize ATP utilization in therapeutics.
Intro to ATP
Adenosine triphosphate, or ATP, is fundamental to almost all biological processes. It functions as the primary energy currency in living organisms and is integral to cellular activities. Understanding ATP is crucial because it offers insights into how cells generate, store, and utilize energy. This knowledge serves a wide range of applications, from basic cellular biology to advanced medical research. The significance of ATP extends beyond energy production; it impacts muscle contraction, active transport, and biochemical reactions vital for life.
What is ATP?
Adenosine triphosphate is a complex organic molecule. It consists of three main components: adenine, ribose, and three phosphate groups. ATP serves as the energy transfer molecule in cells, facilitating energy-requiring processes. When one of its phosphate bonds breaks, ATP releases energy, transforming into adenosine diphosphate (ADP). This cycle of ATP synthesis and utilization drives a myriad of biological functions. Without ATP, cellular life would be unsustainable, as energy wouldn’t be available for necessary reactions.
The Chemical Structure of ATP
The structure of ATP is critical to its function. The adenine component is a nitrogenous base, while ribose is a five-carbon sugar. The phosphorus groups are arranged in a chain, with each linked by high-energy bonds. These bonds store potential energy, which can be quickly accessed when the cell requires it. The structure allows ATP to engage in various reactions, both energy-releasing and energy-consuming, making it versatile. Understanding this structure helps explain ATP’s role as an energy carrier in metabolic pathways.
Historical Context of ATP Discovery
The discovery of ATP dates back to the early 20th century, specifically in 1929 when biochemist Karl Lohmann first identified it as a component responsible for energy transfer in muscle contraction. Subsequent research expanded on its significance in cellular processes. In 1948, Paul D. Boyer and John E. Walker further studied ATP's role in cellular respiration, resulting in a broader understanding of bioenergetics. This historical perspective provides context for ATP's crucial role in biological processes and underpins many current scientific inquiries into energy metabolism.
ATP Synthesis Mechanisms
ATP synthesis is crucial for maintaining cellular function and health. Understanding how ATP is produced offers insight into energy transfer processes that sustain life. The mechanisms through which ATP is generated are diverse, involving various metabolic pathways. Each of these pathways plays a critical role in meeting the energy requirements of different cells and organisms.
Cellular Respiration Overview
Cellular respiration is the primary method by which ATP is produced in many organisms. This process occurs in multiple stages, including glycolysis, the Krebs cycle, and the electron transport chain. Glycolysis happens in the cytoplasm and converts glucose into pyruvate, generating a small amount of ATP. Following this, the pyruvate moves into the mitochondria for further processing through the Krebs cycle, also known as the citric acid cycle.
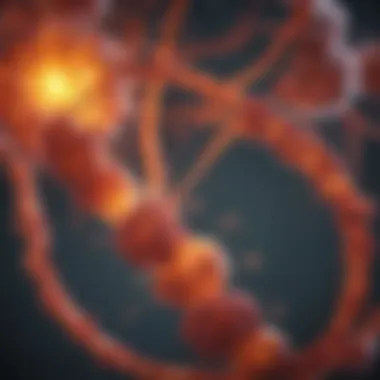
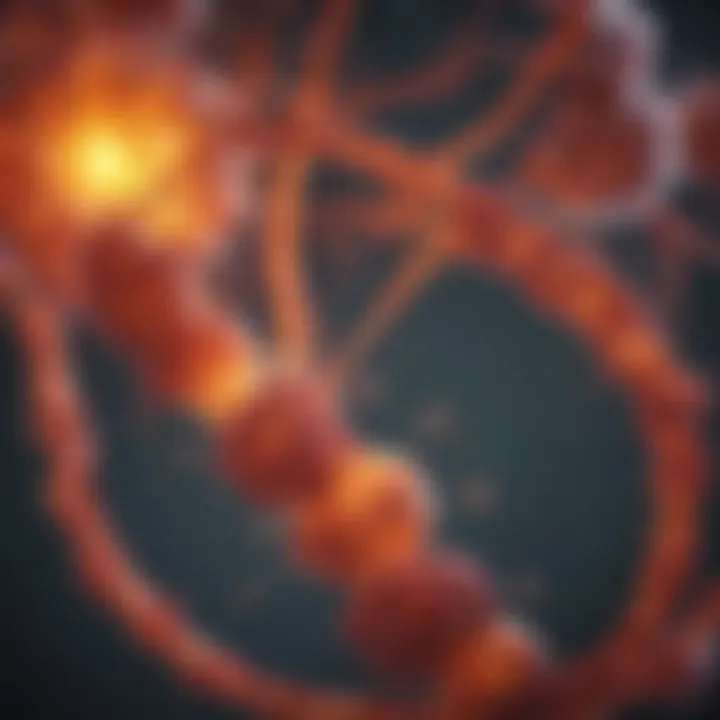
During the Krebs cycle, more ATP is created, along with electron carriers. These carriers transport electrons to the electron transport chain, embedded in the mitochondrial membrane. Here, a series of reactions lead to the production of a substantial quantity of ATP. Ultimately, cellular respiration is highly efficient, converting the energy stored in glucose into ATP, which cells then utilize for various functions.
Substrate-level Phosphorylation
Substrate-level phosphorylation is a direct way of generating ATP during specific metabolic reactions. Unlike oxidative phosphorylation, which relies on electronic transport chains, substrate-level phosphorylation involves the direct transfer of a phosphate group to ADP, forming ATP. This process primarily occurs during glycolysis and the Krebs cycle.
For example, in glycolysis, ATP is produced when a high-energy phosphate group from phosphorylated substrates is directly transferred to ADP.
This mechanism is significant because it allows cells to produce ATP quickly, even when oxygen levels are low. However, it generates less ATP compared to oxidative phosphorylation, making it a complement rather than a replacement.
Oxidative Phosphorylation
Oxidative phosphorylation is the most prolific form of ATP synthesis, occurring in the mitochondria. It uses the energy from electron transport to create a proton gradient across the mitochondrial membrane. As electrons are transported through the chain, protons are pumped from the mitochondrial matrix into the intermembrane space, creating a potential energy difference.
This proton gradient drives ATP synthesis as protons flow back into the matrix through ATP synthase, an enzyme that catalyzes the conversion of ADP and inorganic phosphate into ATP. Overall, this process is integral to aerobic metabolism, allowing for efficient ATP production from nutrients.
"Oxidative phosphorylation accounts for approximately 90% of ATP produced in cells under aerobic conditions."
Photophosphorylation in Photosynthesis
Photophosphorylation is the mechanism of ATP production in photosynthetic organisms, such as plants. During photosynthesis, light energy is captured by chlorophyll and converted into chemical energy. This process involves two major stages: the light-dependent reactions and the light-independent reactions (Calvin cycle).
During the light-dependent reactions, light energy splits water molecules, releasing oxygen and generating ATP through a process similar to oxidative phosphorylation. Protons build up in the thylakoid lumen, and as they flow back into the stroma, ATP is synthesized. This ATP, produced through photophosphorylation, is then utilized in the Calvin cycle to convert carbon dioxide into glucose.
In summary, ATP synthesis mechanisms are varied yet interconnected. Each pathway, whether through cellular respiration, substrate-level phosphorylation, oxidative phosphorylation, or photophosphorylation, contributes to the complex energy balance within biological systems. Understanding these mechanisms underscores ATP's central role in powering life.
Functions of ATP in Biological Systems
Adenosine triphosphate is often referred to as the energy currency of the cell. Its functions extend to various biological processes crucial for maintaining life. ATP is integral to energy transfer, which underpins many cellular activities. Understanding how ATP aids these functions is essential for grasping its overarching role in biology.
Energy Currency in Cells
ATP serves as the primary energy source within cells. When energy is required for a biological reaction, ATP is converted to adenosine diphosphate and a free inorganic phosphate. This process releases energy. This energy drives endergonic reactions. These reactions include the biosynthesis of macromolecules like proteins and nucleic acids, along with muscular contraction and molecular transport.
"ATP's role as the energy currency is pivotal in cellular metabolism and is fundamental to the functioning of all life forms."
This transaction of energy is what sustains numerous cellular processes. The ability of ATP to reform from ADP during cellular respiration or photosynthesis ensures constant energy availability for cells.
Role in Muscle Contraction
Muscle contraction is a highly energy-dependent process where ATP plays a vital role. During contraction, ATP binds to myosin, allowing it to detach from actin filaments within muscle fibers. Once detached, ATP breaks down, which can reset the myosin head for another contraction cycle.
Thus, a continuous supply of ATP is necessary for sustained muscle activity. In the absence of ATP, muscles would remain in a contracted state, leading to paralysis or rigor mortis.
ATP in Active Transport Mechanisms
Active transport mechanisms are crucial for maintaining cellular homeostasis. ATP powers the transport of ions and molecules against their concentration gradients, a process integral for nutrient absorption and waste elimination. For instance, the sodium-potassium pump uses ATP to move sodium ions out and potassium ions into the cell. This transport is critical for maintaining membrane potential and cellular excitability.
The effectiveness of active transport largely depends on ATP availability. Without enough ATP, the transport processes would fail, leading to cellular dysfunction and possible cell death.
ATP as a Signaling Molecule
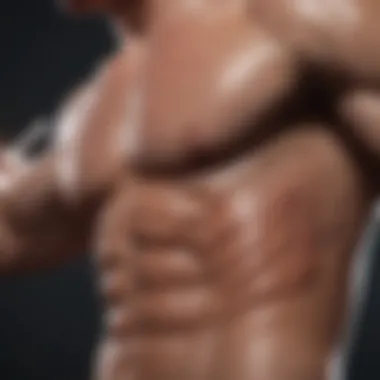
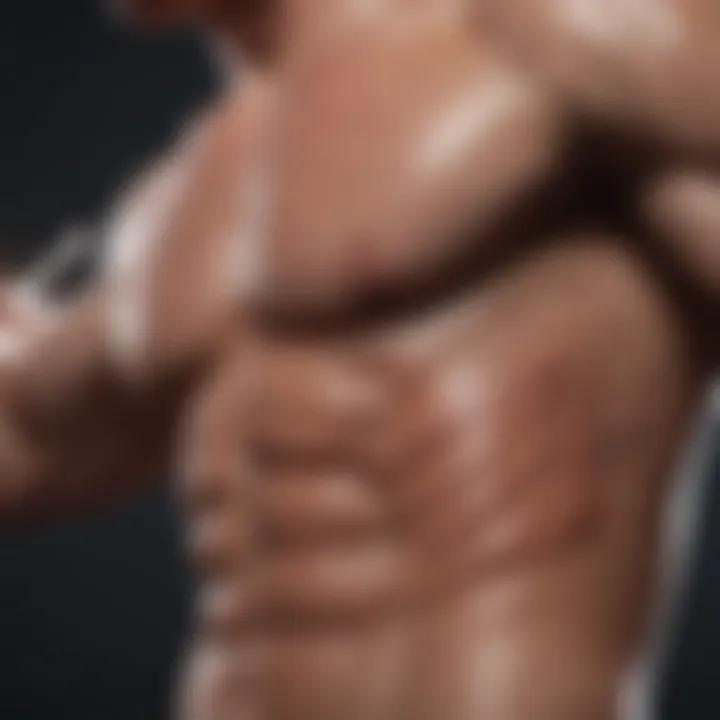
In addition to its energy transfer role, ATP functions as a signaling molecule in various physiological processes. It is involved in cell signaling pathways where it acts as a substrate for kinases, which phosphorylate target proteins, leading to changes in their activity.
ATP’s signaling capability is not limited to simple pathways; it also participates in more complex signaling cascades, impacting numerous cellular functions such as growth, differentiation, and apoptosis. Moreover, it is a crucial component in synaptic transmission within the nervous system, since it is released during neurotransmitter release and modulates neuronal communication.
Understanding the multifaceted roles of ATP enhances comprehension of how cells maintain their functions and respond to environmental changes. ATP does not just supply energy; it orchestrates various biochemical pathways that sustain life.
Regulation of ATP Levels
The regulation of ATP levels is a critical aspect in maintaining cellular functions effectively. ATP, being the primary energy carrier in cells, plays a fundamental role in various biological activities. When ATP levels drop or fluctuate, it can significantly impact cellular processes. Proper management of ATP concentration ensures that cellular activities are carried out smoothly, enabling life processes to function optimally.
Homeostasis of ATP in Cells
Homeostasis of ATP refers to maintaining a stable concentration of ATP within cells. Cells have intricate mechanisms to balance ATP production and consumption. This equilibrium is vital for sustaining cellular health. The main strategy for homeostasis involves synthesizing ATP through pathways such as glycolysis, the citric acid cycle, and oxidative phosphorylation.
Moreover, ATP consumption happens during cellular activities like muscle contraction, biosynthesis, and even active transport. If there is any disruption in these processes, it can lead to an energy crisis within the cell. Cells also adapt to changing conditions, preserving adequate ATP levels. For example, under hypoxic conditions, cells may shift towards anaerobic metabolism to ensure some energy is produced, although less efficiently.
Factors Affecting ATP Production
Several factors determine how much ATP a cell can produce. These include:
- Oxygen Availability: Oxygen is essential for aerobic respiration, which generates the most ATP.
- Nutrient Availability: The presence of glucose and fatty acids directly impacts ATP synthesis. Insufficient nutrients can lead to reduced energy production.
- Environmental Conditions: Factors such as temperature and pH can affect the efficiency of enzymatic reactions involved in ATP production.
- Cell Type: Different cell types have varying capacities for ATP generation based on their metabolic demands. For instance, muscle cells have a higher ATP requirement compared to skin cells.
Understanding these factors helps researchers develop strategies to enhance ATP production, especially in conditions where cellular energy demands are high.
The Role of ATP in Energy Metabolism
ATP is at the center of energy metabolism in biological systems. It acts as a substrate in various biochemical reactions, making it essential for metabolic pathways. ATP not only supplies energy for chemical reactions but also serves to drive necessary biological functions.
Some key roles include:
- Energy Currency: ATP molecules transport energy. They constantly cycle between being stored in a high-energy state and breaking down to release that energy.
- Regulation of Pathways: ATP concentrations can signal metabolic pathways to activate or inhibit, influencing overall energy metabolism.
- Cellular Signaling: In some pathways, ATP is used as a signaling molecule, indicating the energy status of the cell and influencing cellular responses.
ATP in Physiology and Pathology
The understanding of ATP's role in both physiology and pathology is essential for grasping how energy dynamics influence health and disease. ATP, as the main energy carrier in cells, impacts numerous physiological processes by providing the necessary power for countless cellular activities. The significance extends beyond just energy transfer; ATP is also involved in cellular signaling, influencing how cells communicate and respond to various stimuli.
The Role of ATP in Cellular Signaling
ATP acts as a critical signaling molecule in various cellular systems. It is not just a fuel source; rather, it participates in signaling cascades that can alter cell functions. When ATP is hydrolyzed, it releases energy that can drive biochemical reactions essential for life.
- Extracellular Signaling: ATP can be secreted into the extracellular space, where it plays a pivotal role in autocrine and paracrine signaling. It binds to purinergic receptors and influences various physiological responses. This can include vasodilation or neurotransmitter release in neurons.
- Intracellular Signaling: Within the cell, ATP is involved in activating protein kinases and other signaling molecules, leading to a cascade of molecular events crucial for processes like cell proliferation and differentiation.
Understanding these pathways can provide insight into how cells function under normal and pathological conditions.
Lifespan and Turnover of ATP
The lifespan and turnover of ATP are critical components in maintaining cellular energy balance. ATP is constantly being synthesized and utilized to meet the energetic demands of the cell. Some important aspects include:
- Rapid Turnover: ATP turnover can be very rapid, with the average turnover time being just seconds. This is critical in high-energy demanding tissues like heart muscle and skeletal muscle during exercise.
- Synthesis and Degradation: The continuous synthesis of ATP through cellular respiration and degradation through ATP hydrolysis ensures that energy supply meets the demand. Cellular ATP concentrations remain relatively stable due to these tightly regulated processes.
The high turnover rate indicates a dynamic energy management system that is crucial for cell survival, especially in non-dividing cells.
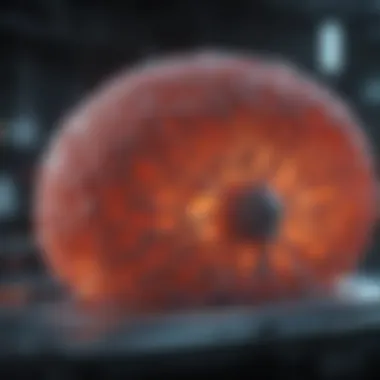
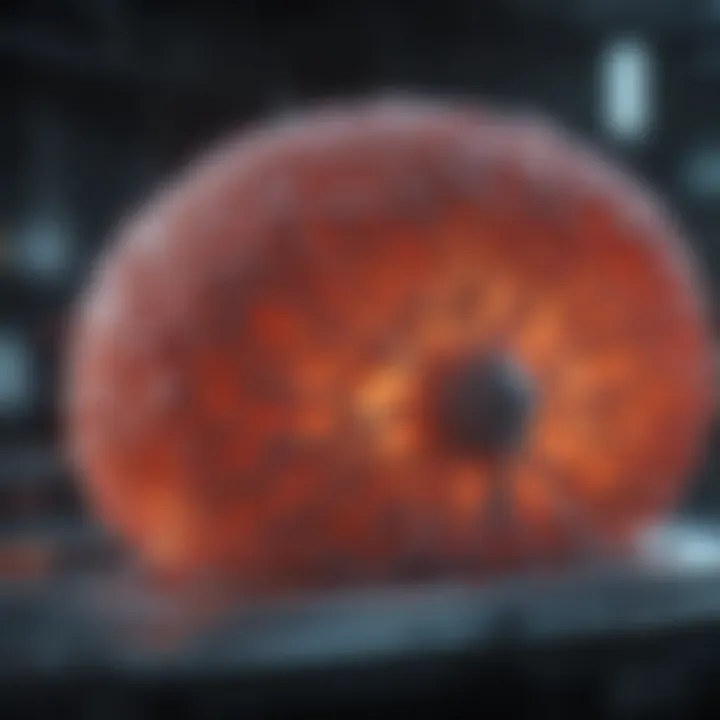
ATP and Disease Mechanisms
Alterations in ATP levels and its signaling pathways can lead to various disease mechanisms. A few areas of concern include:
- Metabolic Disorders: Conditions like diabetes and obesity can be linked to impaired ATP production. Insulin resistance can decrease cellular glucose uptake, leading to less ATP production.
- Neurodegenerative Diseases: In disorders such as Alzheimer’s and Parkinson’s, mitochondrial dysfunction can lead to reduced ATP availability. This is detrimental since neurons heavily rely on ATP for maintaining their functions.
- Cancer: Cancer cells show altered metabolism, often leading to increased glycolysis and ATP production, even under low oxygen conditions (known as the Warburg effect). This shift supports rapid cell division and tumor growth.
In summary, understanding ATP's involvement in physiology and pathology not only clarifies its central role in cellular energetics but also underlines its implications for health and disease management.
Research Advances in ATP Studies
Research on adenosine triphosphate (ATP) continues to unveil a vast array of insights into cellular mechanics and human health. By exploring ATP further, researchers have been able to elucidate its multifaceted role in biological processes. Some key areas of focus include developing new detection methods and understanding ATP's contributions to various diseases. These advances are not only critical for science but they also hold potential for therapeutic advancements, making this a vital topic in the study of cellular activities.
Innovations in ATP Detection Techniques
The evolution of ATP detection methods marks a significant advancement in biochemistry. Traditional techniques like luciferase-based assays have been foundational, but their limitations have pushed the development of more refined approaches. For instance, newer fluorescent probes that are sensitive to changes in ATP concentrations are now in use. These methods allow for real-time monitoring of ATP levels within cells, providing insights into metabolic states and cellular responses to various stimuli.
Benefits of these innovations include:
- Higher sensitivity: Advanced techniques can detect lower concentrations of ATP than earlier methods.
- Real-time data: Researchers can observe cellular ATP changes dynamically, which enhances understanding of energy metabolism in vivo.
- Specificity: New detection methods often target ATP specifically, minimizing interference from other nucleotides.
These improvements are crucial for both fundamental research and clinical applications, providing a more accurate picture of ATP's role in cellular energy dynamics.
ATP's Role in Cancer Research
ATP's involvement in cancer biology is an expanding area of research. Tumor cells often have altered energy metabolism, a phenomenon known as the Warburg effect. This results in increased ATP production even under low-oxygen conditions. Understanding the nuances of ATP metabolism in cancer cells can present new avenues for treatment.
Key points include:
- Potential therapeutic targets: Inhibitors of ATP synthesis pathways could disrupt energy supply to tumors, making them a target for cancer therapies.
- Predictive biomarkers: Measuring ATP levels in tumors may serve as a biomarker for cancer progression and treatment responses, aiding in personalized medicine.
- Understanding tumor microenvironment: ATP release in the tumor microenvironment can promote angiogenesis and immune evasion, which are crucial for tumor growth.
Research into ATP's role in cancer continues to evolve, giving deeper insight into not only how cancer cells operate but also how they might be effectively targeted for treatment.
ATP in Neurobiology and Brain Function
ATP is not just vital for muscle cells but also plays an important role in the brain. Neurons rely heavily on ATP for various functions, such as neurotransmitter release and maintaining ion gradients across the cell membrane. Disruptions in ATP metabolism can correlate with neurodegenerative diseases, making this an essential area of study.
Points of interest include:
- Synaptic function: ATP is crucial for synaptic transmission, impacting how neurons communicate. Changes in ATP levels can directly affect cognition and behavior.
- Neuroprotection: Certain research shows that maintaining ATP homeostasis may protect against conditions like Alzheimer's and Parkinson's disease.
- Role in neural signaling: ATP acts as a signaling molecule, influencing various pathways crucial for sustaining brain health.
Continued research into the roles of ATP in neurobiology can lead to better understanding of neurological diseases and potentially novel therapeutic approaches.
These research advances exemplify how ATP is the keystone in a wealth of biological and pathological processes, underscoring its importance well beyond traditional energy roles.
End
In this article, we have unpacked the multifaceted role of adenosine triphosphate (ATP) in the realm of cellular activities. Understanding ATP extends beyond mere biochemical interest; it is essential for grasping how life operates at the molecular level. The insights gained from examining ATP’s synthesis, function, and regulation present numerous implications for both health and disease management.
Summary of ATP's Importance
ATP is more than just a molecule. It is the fundamental energy currency for all biological systems. From powering muscle contractions to facilitating cellular signaling, ATP plays a critical role in metabolic processes. Its ability to provide immediate energy through hydrolysis makes it indispensable in various physiological functions.
- Energy Currency: ATP acts as the primary energy source, fueling countless biological reactions.
- Regulation of Energy Levels: The cellular homeostasis of ATP influences overall metabolic health and can affect disease states.
- Research and Applications: Ongoing research into ATP’s functions furthers our understanding of health issues, including cancer and neurodegenerative diseases.
The importance of ATP cannot be overstated. It underlines essential biological processes and offers pathways for therapeutic advancement. A comprehensive understanding of ATP equips researchers and medical professionals with the tools needed to innovate in health and science. Overall, ATP stands as a cornerstone of cellular activities, highlighting how energy dynamics govern life itself.
"All life is energy. Understanding ATP is understanding the essence of life."
By honing in on ATP, we illuminate the intricacies of cellular mechanisms and fuel future inquiries into the bioenergetic landscape. Combining fundamental research and applied sciences, the study of ATP reiterates the elegance of life processes, ultimately guiding us toward cures for complex diseases.