Understanding Chemical Synthesis: Definitions and Implications
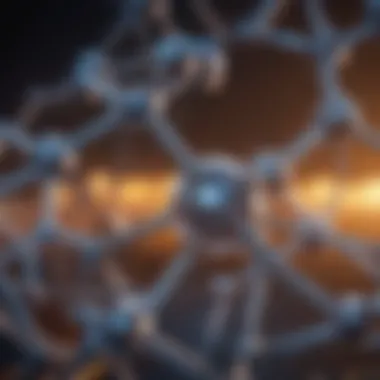
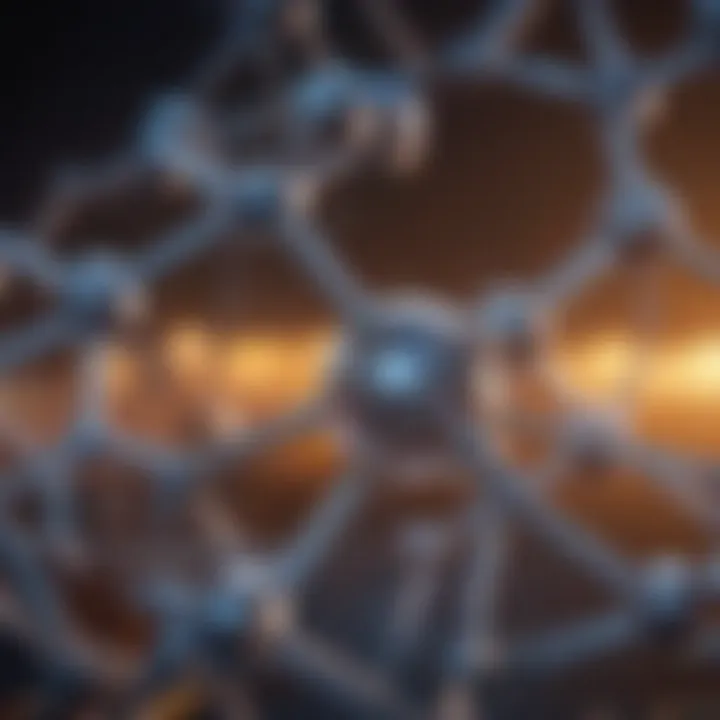
Intro
Chemical synthesis plays a crucial role in the world of science. It involves combining different substances to create new compounds, essential for various applications. This process has transformed numerous industries, including pharmaceuticals, materials science, and environmental engineering. Understanding chemical synthesis enriches our knowledge about how substances interact and how new solutions can be developed.
In this article, we will examine the definitions, methodologies, significance, and contemporary trends in chemical synthesis. By exploring different synthesis processes and technological advancements, we aim to provide valuable insight into this complex field. It is important not just for researchers and students but also for professionals navigating the ever-evolving landscape of science and industry.
Research Overview
Methodological Approaches
The methods of chemical synthesis can be broadly classified into two categories: organic and inorganic synthesis. Each has its own set of techniques and applications. Organic synthesis often involves reactions between carbon-containing compounds. For example, the Bayer-Villiger oxidation transforms ketones into esters. This particular process is valuable in the production of fragrances and pharmaceuticals.
In contrast, inorganic synthesis focuses on compounds that do not primarily contain carbon. A common approach here is solvothermal synthesis, which uses solvents under high temperature and pressure to create nanoparticles. This technique is crucial in creating materials with unique properties.
Different methodologies also emphasize sustainability. Green chemistry aims to minimize the environmental impact of chemical processes. Techniques like biocatalysis utilize natural enzymes to promote reactions at lower energy expenses, leading to more sustainable production.
Significance and Implications
Understanding chemical synthesis is significant for multiple reasons. Firstly, it underpins countless innovations in medication and treatments. For instance, aspirin, a compound synthesized from organic materials, has dramatically improved healthcare.
Secondly, advancements in chemical synthesis can lead to innovative materials that enhance daily life. From new polymers with specific properties to advanced materials for electronics, the potential applications are vast.
Moreover, research in chemical synthesis often intersects with other fields. For instance, studying the chemical pathways in plants can lead to discoveries in natural product synthesis, which can be applied in drug discovery. This interconnectedness highlights the broader implications chemical synthesis has on various scientific disciplines.
"Chemical synthesis is not merely a form of experimentation; it is the foundation of innovation across diverse scientific realms."
Current Trends in Science
Innovative Techniques and Tools
The landscape of chemical synthesis is transforming due to innovative techniques and advanced tools. Enhanced analytical methods, such as mass spectrometry and NMR spectroscopy, allow researchers to closely monitor chemical reactions. This can lead to a more precise understanding of synthesis pathways and the detection of unexpected byproducts. Also, software tools help in predicting reaction outcomes, making the process more efficient.
Another trend is the utilization of machine learning in synthesis optimization. By analyzing vast datasets from past experiments, algorithms can recommend reaction conditions that lead to higher yields. This integration of technology marks a significant advancement, minimizing trial-and-error approaches often seen in traditional synthesis.
Interdisciplinary Connections
Chemical synthesis does not exist in isolation; it connects various disciplines. For instance, in biology, knowledge of chemical synthesis can enhance the development of new drugs targeting specific diseases. In environmental science, synthesized materials can be designed to capture pollutants or improve energy storage solutions.
The collaboration between fields paves the way for breakthroughs. As industries become more aware of sustainability, interdisciplinary approaches can guide the development of greener synthesis methods. The convergence of chemistry with computer science and biology presents an exciting frontier for the future of research and application.
Understanding the implications of chemical synthesis reveals its foundational role in scientific advancement. This exploration illustrates the necessity of ongoing research and innovation within this field, which continues to shape our understanding of materials and compounds crucial to modern science.
Defining Chemical Synthesis
The concept of chemical synthesis is fundamental to the field of chemistry, serving as the backbone for various scientific advancements. This section provides clarity on the term, establishing a framework for understanding its significance and applications. By defining chemical synthesis distinctly, we highlight the processes and techniques concerned, which are vital for students, researchers, and professionals alike. Recognizing the nuances of this definition guides readers in their pursuit of knowledge within the expansive realm of chemistry.
Scientific Definition
Chemical synthesis refers to the process of creating complex chemical compounds from simpler substances. This usually involves a sequence of chemical reactions that transform starting materials, known as reagents, into products. The scientific definition captures the essence of chemical synthesis as both an art and a science.
One way to articulate this is through the equation:
[ extReagents \rightarrow \textProducts ]
Here, reagents undergo various transformations including addition, substitution, and elimination reactions to yield desired compounds. The precision of this process is critical. For instance, in pharmaceutical chemistry, chemical synthesis enables the creation of important drug compounds, making it an essential area of study and work.
Historical Context
The historical evolution of chemical synthesis reveals much about its current applications and methodologies. Beginning in ancient times with alchemy, the aim was to transform common metals into gold and seek elixirs for life. Although these early pursuits were not scientifically valid, they sparked interest in material transformation and experimentation.
The transition to modern chemistry began in the 18th century with figures like Antoine Lavoisier, who emphasized rigorous experimentation over philosophy. This shift set the groundwork for the systematic classification of elements and compounds.
By the 19th century, advancements in organic chemistry further propelled synthetic methods, notably with the synthesis of urea by Friedrich Wöhler. This demonstrated that organic compounds could be generated from inorganic substances, fundamentally altering perceptions of chemical synthesis.
Today, the historical advancements inform current practices in chemical synthesis, emphasizing the importance of synthetic routes, reaction conditions, and material selection. As we continue to develop more efficient and sustainable methods, understanding this context remains crucial for advancing the field.
The Importance of Chemical Synthesis
Chemical synthesis is a cornerstone of modern chemistry and plays a critical role in various scientific and industrial applications. Its importance can be observed in multiple domains, ranging from pharmaceuticals to environmental science. Understanding the implications and benefits of chemical synthesis enhances our knowledge of its vast capabilities. This section focuses on the vital contributions of chemical synthesis and its relevance in today's society.
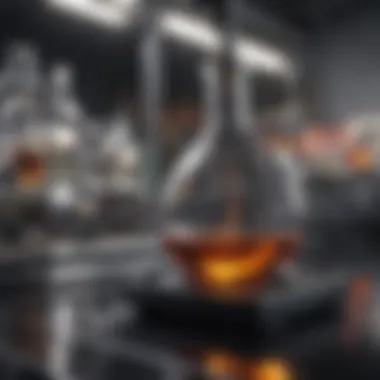
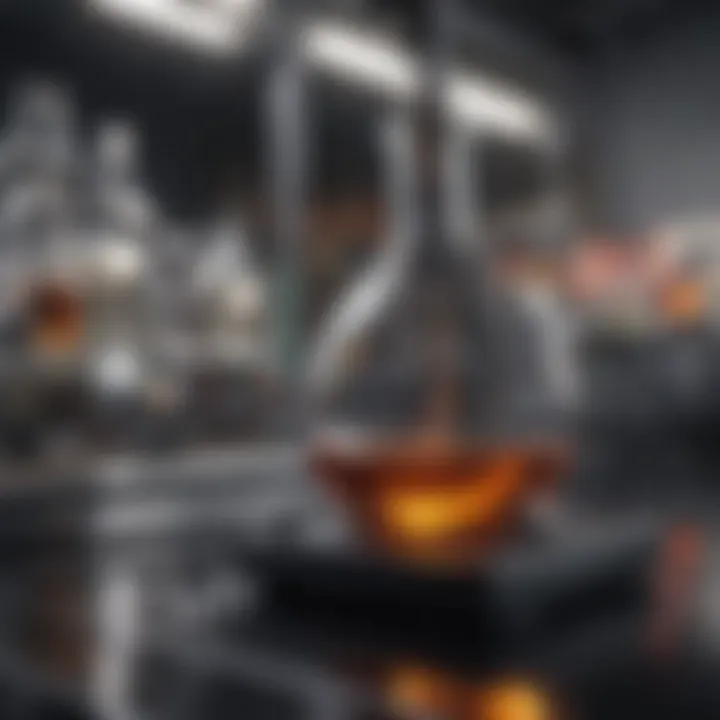
Role in Pharmaceutical Development
Chemical synthesis is indispensable in the field of pharmaceuticals. The development of new drugs heavily relies on the ability to synthesize compounds that can target specific biological functions. Through chemical synthesis, researchers can construct molecules with precise configurations, optimizing their efficacy and safety for medical use.
Many pharmaceuticals, including antibiotics, analgesics, and antihypertensives, stem from complex chemical synthetical routes. Without chemical synthesis, producing these vital compounds would be nearly impossible. The development of synthetic pathways enables the efficient production of these drugs at scale, ensuring availability to those in need.
Moreover, advancements in synthesis techniques have led to the discovery of new therapeutic agents, which can significantly impact disease treatment. Organic compounds, many of which are produced via synthesis, are fine-tuned for selective action against diseases, reducing side effects while improving patient outcomes.
Environmental Applications
In addition to pharmaceutical applications, chemical synthesis plays a crucial role in addressing environmental issues. The synthesis of biopolymers, for example, fosters the development of sustainable materials that can replace conventional plastics. These materials, derived from renewable resources, contribute to reducing waste and pollution.
Moreover, chemical synthesis finds relevance in creating catalysts that promote green chemical reactions. These catalysts provide pathways for reducing energy consumption and minimizing hazardous byproducts.
"Chemical synthesis not only drives innovation in pharmaceuticals but also advances eco-friendly solutions, highlighting its critical role in sustainable development."
Additionally, chemical synthesis is utilized in the formulation of agrochemicals, which optimize agricultural production while decreasing the environmental impact. Innovations in this field can lead to the development of pesticides and fertilizers that are less harmful to ecosystems.
Types of Chemical Synthesis
Chemical synthesis is a diverse field with various methods, each suiting different scientific objectives. In this section, we will explore three primary types of synthesis: Total Synthesis, Partial Synthesis, and Asymmetric Synthesis. Understanding these types is crucial for researchers and professionals as each method brings unique benefits and challenges applicable in varying contexts.
Total Synthesis
Total synthesis refers to the comprehensive process of creating complex organic molecules from simpler ones. This method is particularly significant in pharmaceutical chemistry where complex drug molecules often need full chemical construction. One advantage of total synthesis is that it allows researchers to create new compounds that may not exist in nature. By synthesizing compounds, scientists can also study their properties and potential applications more thoroughly.
However, total synthesis can be resource and time-intensive. The number of steps involved can lead to increased costs and the potential for lower yields. Despite these challenges, total synthesis remains vital for advancing our understanding of molecular design.
Partial Synthesis
Partial synthesis focuses on modifying existing substrates to achieve a desired product. This process often involves the alteration of naturally occurring compounds to enhance their properties. For example, in the development of agrochemicals, researchers may take a naturally occurring herbicide and modify its structure to improve its effectiveness or reduce toxicity.
The benefit of partial synthesis lies in its ability to leverage nature's chemistry. Since the starting materials are often easier to obtain than constructing a compound entirely from scratch, this method can be more economical. Nonetheless, researchers must still carefully consider how modifications will affect the compound's properties and functions.
Asymmetric Synthesis
Asymmetric synthesis specifically produces molecules with a preferred orientation, which is vital in creating chiral molecules prevalent in pharmaceuticals. Chiral molecules are non-superimposable mirror images, which means they can exhibit considerably different biological activities. For example, the two enantiomers of a drug may have different effects; one may be therapeutic while the other could be toxic.
By focusing on producing one enantiomer over the other, asymmetric synthesis is key in drug design. Techniques in this area have advanced significantly, including the use of catalysts that facilitate the production of the desired outcome. However, this method remains technically challenging and requires a high level of expertise to handle correctly.
Methods of Chemical Synthesis
Chemical synthesis encompasses a variety of methods that are essential for creating compounds in both academic research and commercial applications. Understanding these methods is vital for anyone interested in the field of chemistry. Methods vary significantly in their approaches, complexity, and outcomes.
The efficiency and effectiveness of chemical reactions can be enhanced by employing established methods or modern techniques. Each method carries with it specific advantages, possible limitations, and particular suitable contexts for deployment. This section will examine both classical methods and modern techniques of chemical synthesis in detail.
Classical Methods
Classical methods of chemical synthesis have been the backbone of organic and inorganic chemistry for centuries. These methods typically include direct reactions, condensation reactions, and substitution reactions. Traditionally, they involve the manual orchestration of reagents, reagents' careful mixing, and meticulous temperature control.
Key Classical Methods include:
- Synthesis of Alcohols: Often done through reduction of carbonyl compounds.
- Grignard Reactions: Used to form carbon-carbon bonds by reacting magnesium with haloalkanes.
- Esterification: A condensation reaction between alcohols and acids to form esters.
- Aldol Reactions: Combining aldehydes or ketones with an alpha hydrogen to create larger molecules.
While these methods are well-established, they also have certain drawbacks. They often require significant time and labor, leading to lower yields and higher costs. Furthermore, these classical approaches may not readily adapt to the growing demand for sustainable chemistry practices.
Modern Techniques
In response to the limitations of classical methods, modern techniques in chemical synthesis have emerged, enhancing productivity and sustainability in chemical processes. These techniques incorporate advanced scientific concepts and technologies such as automation, flow chemistry, and catalytic methods.
Some prominent modern techniques include:
- Microwave-Assisted Synthesis: This technique uses microwave radiation to accelerate chemical reactions, often reducing the time needed for synthesis.
- Continuous Flow Chemistry: This method allows for continuous processing of reactants, improving efficiency and safety by minimizing hazardous accumulation.
- Photoredox Catalysis: Utilizing light to drive reactions can lead to new pathways and less waste.
- Biocatalytic Methods: The application of enzymes for synthesis can be more environmentally friendly and selective compared to traditional catalysts.
Modern synthesis approaches reflect a shift towards sustainability and efficiency, addressing both cost and environmental concerns.
Technological Advances in Chemical Synthesis
The field of chemical synthesis has experienced considerable transformation due to technological advances. Various innovative techniques and tools have emerged, enabling researchers and industry professionals to enhance productivity, reduce costs, and better address environmental concerns. This section focuses on two significant advancements: automation in synthesis and computational chemistry. These developments have not only improved the efficiency of synthesis processes, but also impacted the way research is conducted in various scientific fields.
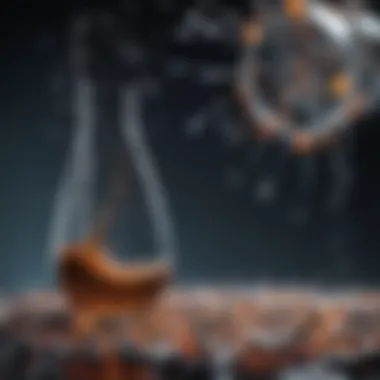
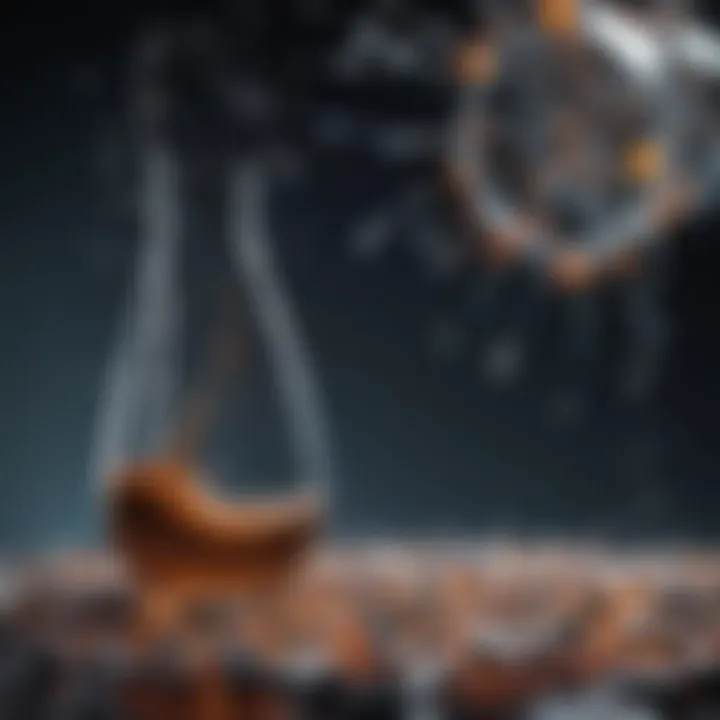
Automation in Synthesis
Automation has revolutionized the landscape of chemical synthesis. Automated systems facilitate repetitive tasks, thereby minimizing human error and increasing throughput. This means that laboratories can produce larger quantities of compounds with consistency and reliability. Most importantly, automation allows chemists to focus on complex problem-solving and innovative design rather than mundane tasks.
Moreover, automated synthesis platforms can operate continuously, leading to significant time savings. Continuous flow synthesis is an example where reactions occur in a flowing system rather than in batches. This method offers several advantages, including better temperature control, improved safety, and enhanced efficiency of reagent use.
Benefits of automation include:
- Increased precision and reproducibility of chemical reactions.
- Reduction in hazardous exposure for chemists working with toxic substances.
- Miniaturization of experiments, allowing for high-throughput screening of compounds.
As automation technology advances, combining it with artificial intelligence further enhances decision-making and optimizes synthesis pathways. Such innovations promise quicker turnaround times for compounds critical in sectors like pharmaceuticals and materials science.
Computational Chemistry
Computational chemistry plays a crucial role in modern chemical synthesis. By using mathematical algorithms and models, researchers can predict the behavior of molecules before they are synthesized. This predictive capability greatly reduces the time and resources required for experimental work.
Key uses of computational chemistry include:
- Molecular modeling: Understanding how different compounds will interact based on their molecular structures aids chemists in designing new drugs.
- Reaction prediction: Computational methods can help foretell the outcomes of synthetic reactions by estimating reaction pathways and product distributions.
- Energy minimization: It allows researchers to find the most stable configurations of molecules, which is essential for synthesis related to biomaterials and other complex structures.
"Computational chemistry empowers chemists to transition from trial and error to informed decisions, increasing the efficiency of synthetic processes."
The results obtained from computational studies can significantly inform experimental synthesis, guiding chemists to more successful and efficient routes.
Both automation and computational chemistry represent critical technological advances, shaping the current and future landscape of chemical synthesis. These tools not only facilitate complex synthesis tasks but also help in mitigating environmental impacts, ensuring that the field addresses some of the most pressing challenges today.
Challenges in Chemical Synthesis
Chemical synthesis faces various challenges that can impede progress and efficiency in the field. Recognizing these obstacles is crucial, as it allows researchers and professionals to develop strategies for overcoming them. The challenges can stem from economic, safety, and environmental considerations, each with its implications for scientific research and commercial applications.
Cost Considerations
Cost is a significant factor in chemical synthesis. The financial implications of developing synthetic pathways can be high. For instance, raw materials and reagents used in synthesis can vary in price, impacting the overall cost of production. Additionally, labor costs and the expenses associated with maintaining sophisticated equipment also play a role.
Furthermore, the cost of failure in experiments can add up quickly. Researchers must allocate funds wisely to avoid unnecessary expenditures. One approach is to use computational modeling to predict the yield and efficiency of chemical reactions, thereby minimizing wasted resources. Angleing for efficient, cost-effective methods not only enhances the viability of projects but also boosts the potential for innovation in chemical synthesis.
Safety and Environmental Concerns
Safety is paramount in any scientific endeavor, and chemical synthesis is no exception. The handling of hazardous substances can pose risks to both researchers and the environment. Accidental spills, exposure to toxic chemicals, and the generation of hazardous waste are pressing concerns that need careful management.
In addition to safety risks, there are increasing regulatory pressures regarding environmental sustainability. Many traditional synthesis methods can lead to significant environmental degradation due to the use of toxic solvents or the generation of hazardous waste. As a response, green chemistry principles are gaining traction. These principles advocate for the design of processes that minimize hazardous substances and waste production.
"Green chemistry seeks to reduce the negative impacts of chemical processes on human health and the environment."
Adopting safer and greener methods can help mitigate these environmental concerns. Researchers are encouraged to explore alternative solvents and catalysts that are less harmful to both people and the planet. Implementing these measures requires a cultural shift within organizations relying on traditional synthesis methods, but it is essential for meeting the challenges of contemporary chemical synthesis.
The Future of Chemical Synthesis
The field of chemical synthesis is undergoing a transformation driven by technological advancements and a growing awareness of sustainability. As the world faces urgent challenges like climate change and resource depletion, the future of chemical synthesis must incorporate innovative approaches and ethical considerations. This section explores pivotal elements such as green chemistry initiatives and emerging trends, emphasizing their importance in reshaping how substances are synthesized.
Green Chemistry Initiatives
Green chemistry focuses on designing chemical processes that minimize waste and harmful substances. This philosophy positions itself as a more sustainable alternative to traditional synthesis methods.
Key principles of green chemistry include:
- Prevention of waste: Creating processes that do not generate unnecessary by-products.
- Atom economy: Maximizing the number of atoms from the reactants that end up in the final product.
- Safer solvents and reagents: Reducing toxic chemicals in reactions.
Implementing green chemistry in industrial processes offers not only environmental benefits but also economic advantages. Companies adopting these principles often find reductions in costs associated with waste disposal and regulatory compliance. Emerging technologies, such as biocatalysis and microwave-assisted synthesis, showcase the potential of green chemistry. Using enzymes in chemical reactions decreases energy consumption and enhances selectivity, leading to fewer wastes. This shift is also mirrored in education, where greening the chemistry curriculum prepares future scientists to prioritize sustainability.
Emerging Trends
Several significant trends are shaping the future of chemical synthesis, reflecting the dynamic intersection of science, technology, and societal needs. Some notable trends include:
- Personalized medicine: The rise of personalized therapies requires unique molecules tailored to individual patients. Chemical synthesis will need to adapt to create these specialized compounds efficiently.
- Automation and robotics: Automation is revolutionizing laboratories, allowing for rapid synthesis and high-throughput screening. This streamlining reduces human error and accelerates the discovery process.
- Machine learning and AI: These technologies facilitate predictive modeling in synthesis, making it possible to foresee potential reactions and optimize processes. This advancement leads to smarter and more resource-efficient methods of synthesis.
The orientation toward interdisciplinary approaches, integrating principles from biology, computer science, and engineering, signifies a paradigm shift. Such integration lays the groundwork for innovations that can tackle complex societal problems, proving that the future of chemical synthesis is not merely about creating new compounds, but also about doing so responsibly.
Applications of Chemical Synthesis
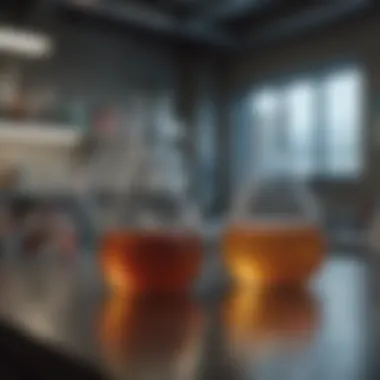
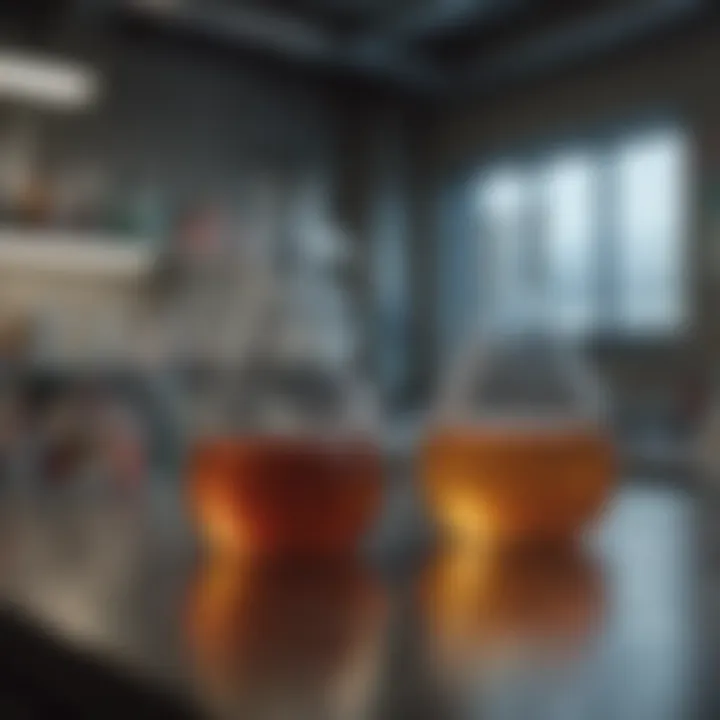
Chemical synthesis plays a crucial role in various scientific fields. It encompasses the creation of complex chemical compounds through specific reactions to achieve desired structures and functionalities. Understanding the applications of chemical synthesis is essential. It highlights the significance of this process in innovation and development across multiple industries.
Chemical synthesis impacts day-to-day life in profound ways. This includes sectors like healthcare, agriculture, and material science. The processes aid in the development of important products—from pharmaceuticals to eco-friendly materials. Knowing how chemical synthesis functions enhances our ability to address global challenges.
Key elements of chemical synthesis applications include:
- Biomaterials: Development of materials that can integrate with biological systems.
- Agriculture: Creation of fertilizers, pesticides, and plant growth regulators to improve crop yield.
Biomaterials Production
Biomaterials are substances engineered to interact with biological systems. They find strong applications in medical fields. For instance, biomaterials like implants and prosthetics require precise chemical synthesis to ensure biocompatibility. Techniques used can range from total synthesis of polymers to partial modifications of existing materials.
In recent years, the significance of biodegradable biomaterials has surged. These materials reduce long-term environmental impact. The synthesis of polylactic acid from renewable resources, for example, represents a major advancement in green chemistry.
Benefits of biomaterials production through chemical synthesis:
- Improved patient outcomes
- Reduced toxicity
- Sustainability
"The efficient synthesis of biomaterials opens doors to innovations in regenerative medicine and tissue engineering."
Agricultural Chemistry
Agricultural chemistry is another significant area influenced by chemical synthesis. This field focuses on the development of products to enhance agricultural productivity. Synthesis processes are vital for creating fertilizers, herbicides, and insecticides. Each component is carefully designed through specific reactions to optimize performance and minimize harm to non-target organisms.
Advancements in chemical synthesis have led to more efficient agricultural inputs. For instance, the synthesis of controlled-release fertilizers ensures that nutrients remain available to plants longer. This reduces the environmental impact of over-fertilization and enhances crop growth. The market for biopesticides is also growing. These products arise from natural sources and involve less synthetic chemistry, leading to safer alternatives for pest control.
The importance of agricultural chemistry synthesized products can be broken down into:
- Increased crop yield
- Sustainable practices
- Reduction in harmful chemicals
Overall, the applications of chemical synthesis in biomaterials production and agricultural chemistry illustrate wide-ranging benefits. They show how chemistry can enhance quality of life while also addressing pressing environmental issues.
Ethical Considerations
Discussing ethical considerations in chemical synthesis is crucial. Chemistry, like any scientific field, intersects significantly with societal values and responsibilities. The implications of chemical synthesis extend beyond the laboratory; they influence health, environment and economy. Thus, understanding the ethical ramifications of these processes is essential for responsible practice.
Impacts on Society
Chemical synthesis has profound effects on society. For example, pharmaceuticals produced through synthesis can save lives and improve quality of life. However, access to these compounds can sometimes exacerbate inequalities. Additionally, the environmental impact of certain synthesis processes can harm community health.
In agriculture, chemical synthesis contributes to fertilizers and pesticides that enhance food production. Yet, excessive use can lead to soil depletion and water pollution. The balance of benefits and detriments is a point of ethical deliberation.
"The responsibility lies not only in the creation of substances but also in their stewardship in society."
Thus, the implications of chemical synthesis are significant. When developing new compounds, scientists must contemplate possible societal repercussions, emphasizing transparency and community engagement throughout the process.
Regulatory Frameworks
Regulatory frameworks play a vital role in the ethics of chemical synthesis. Various government bodies, such as the Environmental Protection Agency (EPA) in the United States, set guidelines that ensure the synthesis processes do not harm public health or the environment. Compliance with these regulations is essential.
Furthermore, many organizations adhere to specific ethical guidelines. The American Chemical Society (ACS), for example, outlines principles to guide professionals in ethical decision-making. A culture of accountability must be cultivated within the scientific community. By implementing robust protocols, the potential risks associated with chemical synthesis can be mitigated.
In summary, ethical considerations in chemical synthesis underscore the importance of responsibility towards society and the environment. Societal impacts must be weighed against the benefits of innovation. Thus, thorough regulatory frameworks ensure that ethical practices are upheld, allowing the field to advance sustainably.
End
In concluding this exploration into chemical synthesis, it is crucial to understand its broad implications in the scientific and industrial spheres. The topic encompasses various aspects that hold substantive relevance today. From the definitions to the multifaceted applications and ethical considerations, chemical synthesis is not just a branch of chemistry; it is a pivotal component that underpins countless innovations and discoveries.
Summary of Key Points
Chemical synthesis plays a fundamental role in diverse fields such as pharmaceuticals, agriculture, and environmental sciences. Here are some of the key points discussed:
- Defining Chemical Synthesis: Understanding both the scientific definitions and historical context provides a solid foundation for appreciating its evolution.
- Importance: The significant role this process plays in developing new drugs and sustainable materials highlights its relevance in solving global challenges.
- Types and Methods: The categorization of synthesis methods into total, partial, and asymmetric synthesis clarifies the approaches researchers employ.
- Technological Advances: Modern techniques and automation have revolutionized how synthesis is conducted, improving efficiency and precision.
- Challenges and Ethics: Addressing the cost and safety issues ensures responsible advancement in the field.
- Looking Forward: Emerging trends and green chemistry initiatives indicate a shift towards more sustainable practices in synthesis.
Looking Ahead
As the field of chemical synthesis continues to evolve, it is essential to recognize the possibilities ahead.
- Sustainability: The increasing focus on green chemistry will push researchers to seek more eco-friendly methods.
- Interdisciplinary Approaches: Collaborations among chemists, biologists, and engineers are likely to yield innovative solutions. This interdisciplinary synergy will enhance the efficiency and applicability of synthesis techniques.
- Regulatory Frameworks: Understanding and navigating the regulatory landscape will be essential for safe and ethical development of new synthetic methods.
In summary, the conclusion drawn from this article emphasizes the significance of chemical synthesis in shaping the future of science and industry. As researchers and practitioners navigate challenges and explore new opportunities, the insights gathered here will serve as a foundation for greater innovation and understanding in this vital field.
"Chemical synthesis not only enables the creation of new substances but also drives the progress and sustainability of various industries."
The insights presented aim to equip students, researchers, educators, and professionals with a comprehensive understanding of the intricate world of chemical synthesis.