Understanding Exoskeletons: Structure, Function, and Applications
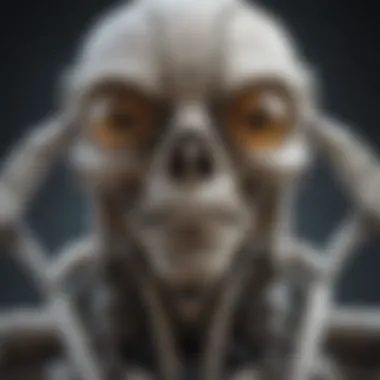
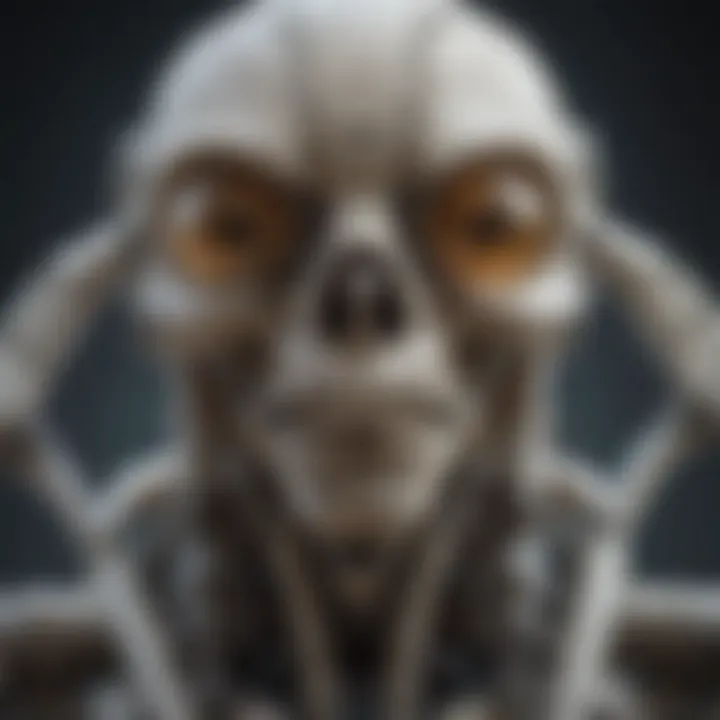
Intro
Exoskeletons serve as fascinating subjects of study in both biological and engineering contexts. Their unique structure and function offer insights into how organisms interact with their environments and how we can mimic these systems for various applications. Essentially, exoskeletons can be defined as supportive external structures that provide protection and facilitate movement.
In biology, they represent a significant evolutionary adaptation for many species, ranging from arthropods to certain mollusks. Artificially, exoskeletons have opened doors in engineering and medical fields, where they are utilized for rehabilitation and to enhance human capabilities.
This article will explore in detail the fundamental aspects of exoskeletons, discussing their structural characteristics, functional roles, and diverse applications. By delving deeper into these areas, we aim to present a comprehensive overview that will engage a diverse readership, including students, researchers, educators, and professionals.
Research Overview
Methodological Approaches
In researching exoskeletons, both biological and artificial, a variety of methodologies are employed. These often include:
- Field Studies: Observing natural organisms in their habitats provides insights into how exoskeletons function in real-world environments.
- Laboratory Experiments: Controlled experiments help in understanding the mechanics of exoskeletons, allowing for focused studies on specific characteristics.
- Computer Simulations: Modeling tools can predict outcomes and performance, particularly in the design of artificial exoskeletons.
Combining these approaches yields a holistic understanding of how exoskeletons operate both in nature and technology. Researchers adopt a multidisciplinary perspective, drawing from biology, materials science, and engineering principles.
Significance and Implications
The significance of studying exoskeletons cannot be overstated. Understanding their design and functionality has crucial implications in various fields. For instance:
- Medical Applications: Exoskeleton technologies enhance mobility for individuals with disabilities, partly improving their quality of life.
- Robotics: Insights from biological exoskeletons inform robotic design, leading to advancements in bio-inspired robotics that can navigate complex terrains.
- Environmental Science: Knowledge of exoskeletal functions in ecosystems contributes to conservation efforts and the understanding of biodiversity.
"Studying the structure and function of exoskeletons provides valuable insights not just into nature, but also into technological advancements that align with biological mechanisms."
Current Trends in Science
Innovative Techniques and Tools
Recent trends in the study of exoskeletons include the use of advanced scanning techniques, such as 3D scanning and imaging technologies. These tools enable scientists to create detailed models and better understand the anatomy of exoskeletal structures. Moreover, the development of robust materials that mimic natural exoskeletons aids in the creation of more efficient and flexible artificial designs.
Interdisciplinary Connections
In the study of exoskeletons, cross-disciplinary collaboration is vital. Fields such as biomechanics, mechanical engineering, and biology come together to advance the understanding of these structures. Innovations often arise at the intersection of these disciplines, leading to synergies that propel research forward.
Collaborative efforts foster the exchange of knowledge and ideas, which is essential for tackling complex problems associated with exoskeleton design and application. By integrating diverse perspectives, the research community can push the boundaries of what is currently possible.
Overall, exploring exoskeletons through these lenses will not only deepen our understanding but also inspire future innovations that enhance both human and ecological wellbeing.
Intro to Exoskeletons
The study of exoskeletons captures the imagination of scientists, engineers, and scholars alike. Exoskeletons serve as a fascinating intersection of nature and technology, illustrating how structural supports can emerge in biological organisms and artificial constructs. This section will provide a foundational understanding of exoskeletons, setting the stage for deeper exploration into their characteristics, functions, and various applications.
Definition of Exoskeletons
Exoskeletons are rigid external coverings that provide structural support to certain organisms. They differ from endoskeletons, which are internal and found in animals like mammals. The primary function of an exoskeleton is to protect the organism from predators and environmental threats. Moreover, it also contributes to mobility and support, allowing the organism to thrive in its environment. Exoskeletons are found in a wide range of species, including insects, crustaceans, and mollusks. In artificial context, human-made exoskeletons aim to augment human abilities or assist in rehabilitation.
Historical Overview
The concept of exoskeletons has existed for centuries, although its understanding and applications have evolved significantly over time.
- In Biology: The earliest records can be traced back to ancient texts that mention the protective features of crustaceans and insects. Over time, naturalists like Aristotle studied these organisms, noting their unique adaptations.
- In Engineering: The modern exoskeleton, particularly in robotics, began developing in the latter half of the 20th century. Early prototypes were simplistic, but advancements in technology have led to more sophisticated models that can support human movement. Key developments occurred during military research aimed at enhancing soldier capabilities in arduous environments.
Understanding exoskeletons is more than an academic pursuit; it bridges various disciplines—biology, technology, medicine, and environmental science. As we explore the many facets of exoskeletons in subsequent sections, a comprehensive view will emerge that highlights their significance in both natural ecosystems and technological innovations.
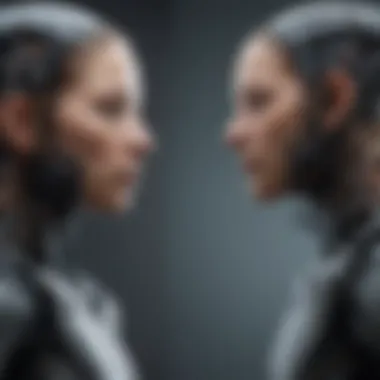
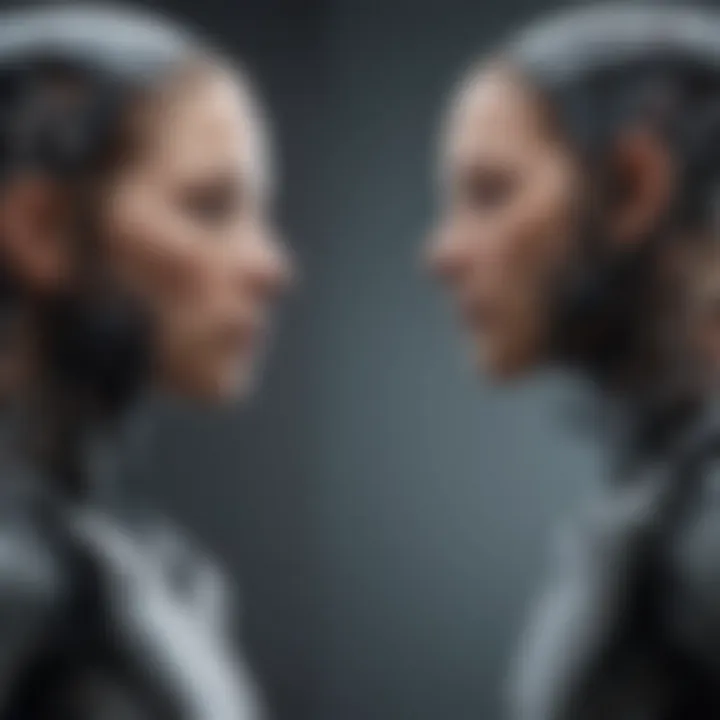
Biological Exoskeletons
Biological exoskeletons represent an essential element in the understanding of exoskeletal structures and functionalities. These natural frameworks not only provide durability but also facilitate adaptations for survival in varied ecosystems. By analyzing biological exoskeletons, we gain insight into evolutionary advancements that have shaped entire species. The complexities of these structures offer numerous benefits, such as protection from predators and environmental stressors, which are critical for the survival of organisms. Furthermore, they serve as a foundation for biomimicry in the design of artificial exoskeletons, thus bridging biological and technological innovations.
Characteristics of Biological Exoskeletons
Biological exoskeletons are typically rigid external coverings that provide protection and support. They can vary significantly in material composition, often being made of chitin or calcium carbonate. This variance contributes to the diversity in function and adaptation across different species. One of the primary characteristics of biological exoskeletons is their segmented structure, which allows flexibility while maintaining strength. The robustness of these materials offers a unique strategy for organisms to thrive in diverse environments, making their study invaluable for both biological science and applied engineering.
Examples in Nature
Arthropods
Arthropods are among the most well-known examples of biological exoskeletons. They have a segmented body and jointed limbs, which allow for considerable mobility. The key characteristic of arthropods is their hardened exoskeleton made from chitin, providing both protection and support. This tough outer layer is lightweight, making it a beneficial choice for energy-efficient movement. However, a distinct disadvantage is that arthropods must molt to grow, leaving them vulnerable during this process.
Mollusks
Mollusks exhibit a different type of exoskeletal structure, typically composed of calcium carbonate shells. This choice of material provides significant protection against predators and adverse environmental elements. The unique feature of mollusks is the ability to produce shells that can be thin or thick depending on environmental pressures. While these structures confer advantages in protection, their heavy weight can limit mobility compared to more flexible structures found in other organisms.
Echinoderms
Echinoderms, such as sea stars and sea urchins, display a distinct exoskeletal system characterized by calcareous plates. These plates are often arranged in a unique pattern that gives rise to their well-known radial symmetry. The key characteristic of echinoderms is their regenerative ability, allowing them to recover from damage. This feature makes them popular in research regarding regeneration. However, their rigidity can inhibit flexibility in movement, presenting both advantages and challenges within their ecosystems.
Function and Adaptation
Protection
Protection is a primary function of biological exoskeletons. They serve as barriers against physical threats and environmental hazards. The thickness and material properties of various exoskeletons directly correlate with the level of protection afforded. For instance, the role of protection in mollusks helps them survive in predatory seas. However, increased thickness can lead to limitations in movement or resource acquisition.
Support
Support is another critical function provided by exoskeletons. They offer structural integrity to the organism, allowing for the attachment of muscles and facilitating movement. This function is especially important in arthropods, where the support structure allows for powerful locomotion despite their small size. Yet, an overly rigid exoskeleton can hinder growth as seen in certain scenarios where the organism struggles to expand during the molting phase.
Locomotion
Locomotion relies heavily on the exoskeleton's design and function. Biological exoskeletal structures allow for various modes of movement among different species. The flexibility in segmented arthropods enables quick and agile movements, while echinoderms use their unique arrangement to facilitate slow but steady motion. The major advantage here is that diverse locomotion strategies contribute to adaptability and survival. On the downside, limitations arise when the design cannot support rapid movement in dynamic environments, impacting their ability to escape threats or pursue food.
Artificial Exoskeletons
Artificial exoskeletons represent a fascinating intersection of technology and human capability. These systems aim to enhance physical capabilities, enabling users to perform tasks that may be challenging or impossible due to physical limitations. The relevance of artificial exoskeletons spans various fields, including healthcare, rehabilitation, manufacturing, and military applications. Their potential to improve productivity and quality of life is significant, making them a prominent topic in discussions about future technological advancements.
Definition and Types
Powered Exoskeletons
Powered exoskeletons utilize motors and sensors to assist movement. They actively augment the user's strength and endurance, redefining how individuals approach physical tasks. A key characteristic of powered exoskeletons is their ability to adapt to the user's movements in real time. This responsiveness enhances the user experience, making these devices effective for rehabilitation and workplace assistance. One unique feature is the integration of advanced robotics, allowing for complex motion patterns. However, their high cost and dependency on batteries can limit accessibility for some users.
Passive Exoskeletons
Passive exoskeletons function without motors, relying on mechanical components to redistribute weight and reduce strain on the body. This design is simpler and tends to be more affordable than powered versions. Their main advantage lies in their ease of use; they often require little to no training. A distinctive feature is their lightweight nature, which ensures minimal fatigue during tasks. Nonetheless, passive exoskeletons may not provide the same level of support as powered ones, potentially limiting their effectiveness for intensive activities.
Applications in Industry
Manufacturing
In manufacturing, exoskeletons help improve worker productivity and reduce injury rates. These systems support heavy lifting and repetitive tasks, which are common in industrial settings. A significant benefit is their ability to alleviate physical strain, allowing workers to maintain a higher output level with less fatigue. The efficiency gained can lead to increased production rates, making them appealing to employers.
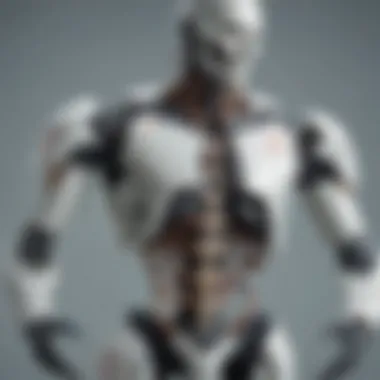
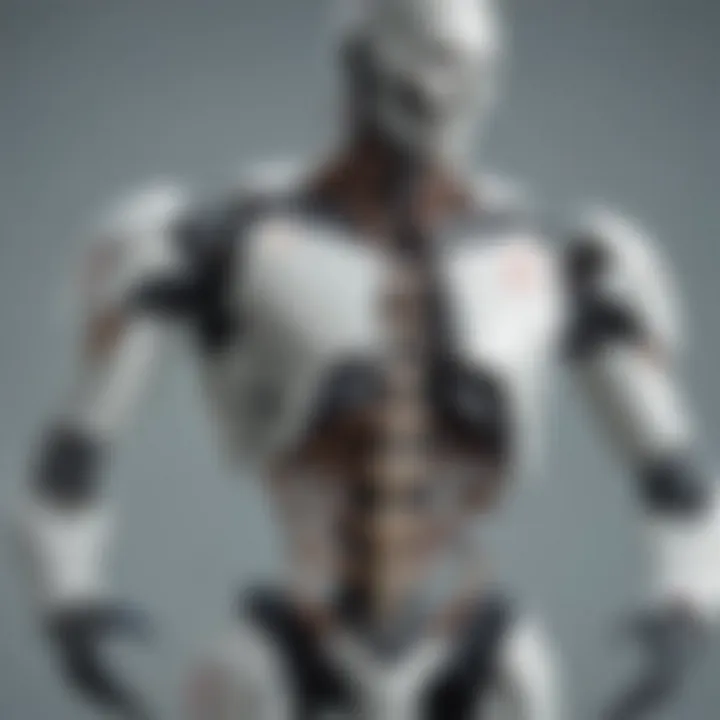
Construction
Exoskeletons in construction assist workers with lifting and moving heavy materials. They provide physical support, enhancing the safety and well-being of laborers. Their contribution to worker safety is crucial, as construction sites often pose significant risks. The unique aspect of exoskeletons here is their ability to reduce the potential for musculoskeletal injuries. However, adaptability to different physical environments can be a challenge during implementation.
Healthcare
In healthcare, exoskeletons play a vital role in rehabilitation. They enable patients with mobility impairments to regain movement. One of the key advantages is the potential for improved physiotherapy techniques. By offering real-time feedback and support, they foster faster recovery. Yet, they can be expensive and may not be covered by health insurance in some regions, which poses a barrier to broader adoption.
Challenges in Development
Cost Considerations
The development and implementation of artificial exoskeletons often face high cost obstacles. Research and production require significant investment, making them less accessible for smaller companies or individual users. A crucial consideration is the balance between cost and effectiveness. While cutting-edge technologies can be expensive, they must also deliver tangible benefits to justify the investment.
User Safety
User safety is paramount in the development of exoskeletons. As these devices augment physical capabilities, they bring potential risks, such as accidents or unexpected movements. A key aspect is the need for thorough testing and regulatory approval before widespread use. Ensuring that users can operate these devices without injury or harm is essential to their adoption in various fields.
Regulatory Issues
Navigating the regulatory landscape can be complex for artificial exoskeletons. Regulations vary widely by country and application, which can impede development timelines. A notable characteristic is that securing approvals can be lengthy, hindering innovation. Balancing the need for oversight and encouraging technological advancements is a challenge that developers often face.
Exoskeletons represent a promising technology that can significantly enhance human capacity, but addressing the challenges is crucial for their successful integration.
The Role of Exoskeletons in Medicine
The integration of exoskeletons in medicine represents a transformative advancement that enhances patient care and improves rehabilitation effectiveness. The potential of these devices can significantly influence various rehabilitation practices. Exoskeletons facilitate movement for individuals with mobility impairments, offering innovative solutions that were previously limited. This section emphasizes the myriad ways exoskeletons can benefit medical applications.
Rehabilitation Technologies
Rehabilitation technologies utilizing exoskeletons have gathered momentum in recent years. Numerous advancements in this field target individuals recovering from strokes, spinal cord injuries, or other mobility-related disorders. Exoskeletons like the EksoGT or ReWalk provide mechanical assistance designed to support patients during their rehabilitation sessions. They promote natural walking patterns, which is important for relearning motor skills.
One of the primary benefits is the ability to provide consistent gait training. Through repetitive motions, the patient can rebuild muscle memory and strength. Furthermore, exoskeletons can be programmed to adapt to the user's progress. Lower limbs' support helps reduce the need for external help, which enhances both mental and physical wellbeing. Research indicates that using exoskeletons in rehabilitation can shorten recovery times and improve overall patient outcomes.
Mobility Assistance
Exoskeletons also serve crucial roles in mobility assistance for those who may not fully regain their ability to walk independently. Devices such as the Honda Walking Assist focus on providing support during daily activities. These devices can be particularly beneficial for the elderly or those with progressive diseases. By enabling movement, exoskeletons can reduce dependency on caregivers and improve quality of life.
Importantly, mobility assistance devices incorporate various technologies. Many of these devices feature sensors which detect the user’s motion intentions. This feedback allows the device to respond in real-time, leading to a more intuitive experience. Such technological integration fosters a degree of independence while enhancing safety.
"Exoskeletons in medicine embody the fusion of technology and compassion, revolutionizing how patients experience rehabilitation and mobility support."
In summary, the role of exoskeletons in medicine is multifaceted. From enhancing rehabilitation processes to fostering independence in mobility, these devices offer solutions that span a wide range of patient needs. Ongoing research and technological advances continue to drive innovations in this crucial area.
Future Trends in Exoskeleton Research
The field of exoskeleton research is evolving rapidly, spurred by technological advances and increasing demand in various sectors. This section explores significant future trends that could shape the next generation of exoskeletons. These trends focus not just on enhancing performance but also on improving user experience, sustainability, and integration into existing systems.
Innovations in Design
Design innovations are crucial for making exoskeletons more adaptable and user-friendly. Researchers are developing lighter and more flexible materials that enhance mobility while reducing fatigue for the user. Significant progress in biomimetic designs is helping architects of these devices create exoskeletons that mimic natural movements more closely. These innovations include:
- Modular Designs: Allowing customization for various body types and preferences. This means users can adjust components without needing entirely new systems.
- Wearable Sensors: Incorporating advanced sensors to monitor user movements and provide real-time feedback. Such technology helps in personalizing the operation of the exoskeleton according to user needs.
- Advanced Power Sources: Exploring alternatives to bulky batteries, like energy-harvesting technologies. These can convert body motion into energy, extending the operational time of exoskeletons significantly.
Each of these innovations pushes the boundaries of what exoskeletons can accomplish, making them more viable for everyday use.
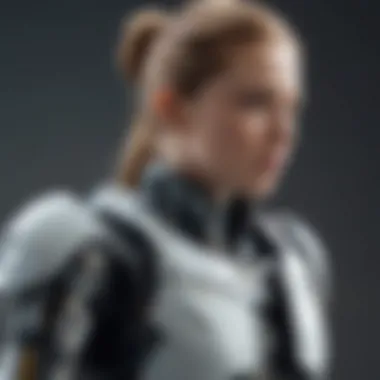
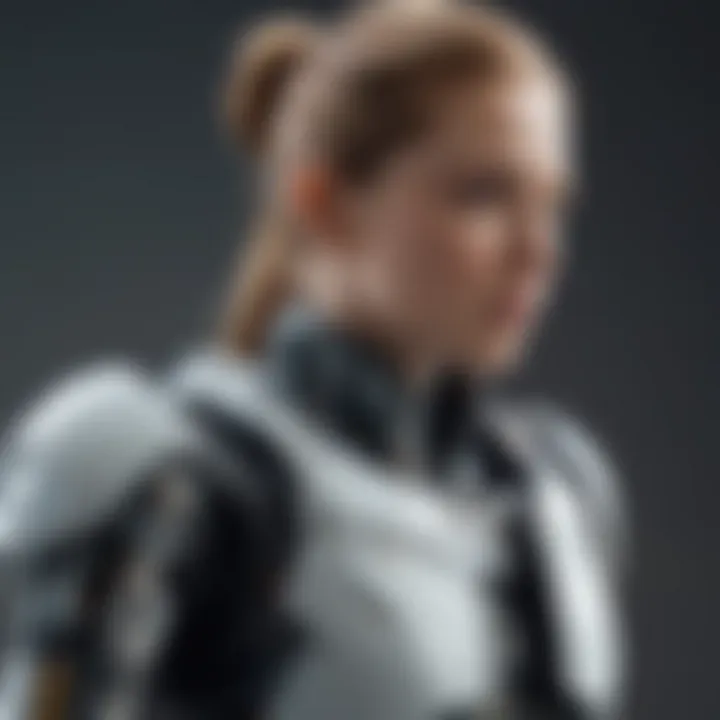
Integration with AI and Robotics
The integration of artificial intelligence and robotics into exoskeletons represents a significant frontier in research. This combination aims at creating smarter systems that can learn and adapt to the user's movements and needs over time. Some key elements of this integration include:
- Machine Learning Algorithms: These will enable exoskeletons to analyze the user’s patterns and adjust their support dynamically. This intelligence increases efficiency, offering tailored assistance during tasks.
- Predictive Analytics: Using data analytics, exoskeletons could predict the best movement strategies for users, minimizing the risk of injury during complex movements.
- Enhanced Interaction with Environments: Developing systems that allow exoskeletons to interact intelligently with their surroundings, optimizing performance in diverse settings, such as challenging terrains or specific work tasks.
"Integrating AI not only enhances functionality but also personalizes the experience for the user, making assistance more intuitive and effective."
As these technologies converge, we may witness exoskeletons that not only assist but also enhance human capabilities in profound, unforeseen ways.
Exoskeletons and Environmental Impact
The environmental impact of exoskeletons is an increasingly significant aspect of their development and application. With growing awareness of sustainability, the focus shifts to how these structures can align with ecological principles. Examining exoskeletons through the lens of environmental impact reveals both challenges and opportunities for innovation.
One critical element is the need for materials that minimize harm to the environment. As artificial exoskeletons are developed for various uses, including medical and industrial applications, the materials selected must exhibit not only durability but also biodegradability. By integrating environmental considerations into the design process, we can potentially reduce waste and the ecological footprint of these technologies.
Biodegradable Materials in Exoskeleton Design
Using biodegradable materials in the design of exoskeletons is crucial for reducing environmental impact. These materials, often derived from natural sources, can disintegrate over time when exposed to environmental conditions, preventing long-term pollution.
Some examples of biodegradable materials include:
- Polylactic Acid (PLA): Derived from corn starch, PLA is a common bioplastic that can be used in 3D printing of exoskeleton components.
- Natural Fibers: Materials such as hemp or flax can provide structural integrity while being more sustainable.
- Starch-based Polymers: These can offer flexibility and strength, making them suitable for various applications.
Incorporating such materials into exoskeleton design not only contributes to environmental sustainability but also opens avenues for innovation. Designers must consider performance against the backdrop of the ecological context, ensuring that functionality does not sacrifice environmental responsibility.
Sustainable Practices in Production
Sustainable practices in the production of exoskeletons play a pivotal role in mitigating their environmental impact. Employing environmentally friendly manufacturing processes can significantly reduce energy consumption, waste production, and reliance on non-renewable resources.
Some sustainable manufacturing practices include:
- Energy-efficient production: Utilizing renewable energy sources during manufacturing minimizes carbon emissions.
- Waste reduction strategies: Implementing lean manufacturing processes can efficiently manage raw materials and reduce scrap waste.
- Recycling and reusing materials: Creating systems that allow for the recycling of components at the end of their lifecycle helps close the sustainability loop.
By focusing on sustainable practices, manufacturers can contribute to responsible development of technologies that aim to improve human capacity without degrading the environment. As we advance in exoskeleton research and application, it will be essential to prioritize these practices to drive positive change in how we think about technology in relation to nature.
"The future of exoskeletons hinges not only on their utility but also on their compatibility with ecological sustainability."
In summary, understanding the environmental implications of exoskeletons informs better production techniques and material choices. It encourages a holistic approach to technology development that respects our planet.
Ending
The conclusion of this article is a vital segment that synthesizes the insights gathered throughout. It reflects on how exoskeletons, both biological and artificial, manifest remarkable structural and functional attributes. Understanding these aspects can profoundly impact various fields, including medicine, robotics, and environmental science.
Summary of Key Insights
The key insights from the article highlight the diverse nature of exoskeletons. Here are the essential points:
- Definition and Historical Context: The article began with clarifying what exoskeletons are and explored their historical evolution. Understanding this context is crucial for grasping their significance today.
- Biological Exoskeletons: We examined the characteristics of biological exoskeletons and provided noteworthy examples from nature. This aspect illustrates adaptation's role in ecological longevity.
- Artificial Exoskeletons: The discussion moved into the realm of artificial constructs. The distinctions between powered and passive exoskeletons are essential in comprehending their practical implementations in industries like healthcare and construction.
- Environmental Considerations: We also considered the ecological implications of developing exoskeletons, emphasizing the need for sustainable practices and the use of biodegradable materials.
- Future Trends: Lastly, the exploration of innovations and integration with AI frames a forward-looking perspective on how exoskeleton technology will continue to evolve.
"A comprehensive understanding of both biological and artificial exoskeletons is crucial for innovation in technology and sustainable practices in industries."
Call for Ongoing Research
Continuous research into exoskeleton technology is essential for several reasons. First, innovations must address the limitations faced in current designs. These include user adaptability, regulatory challenges, and cost issues. The field is dynamic, requiring interdisciplinary collaboration among biologists, engineers, and ethicists to navigate ethical considerations surrounding human augmentation.
Furthermore, investigating new materials and designs can lead to more effective and environmentally friendly exoskeletons. Engaging with the latest developments in artificial intelligence may enhance functionality, making these systems more intuitive and efficient.
To stimulate further exploration, the following areas deserve attention:
- Enhanced functionality in wearable exoskeletons for mobility assistance
- Sustainable production methods to minimize environmental footprints
- In-depth analysis of user experience to improve designs and functionalities