Understanding Glutamate: Key Neurotransmitter Insights
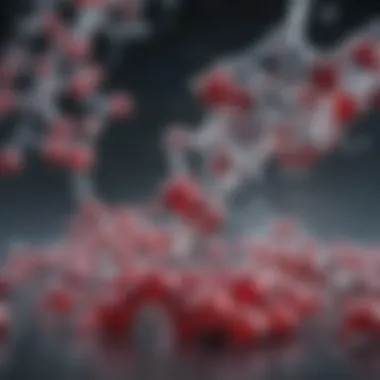
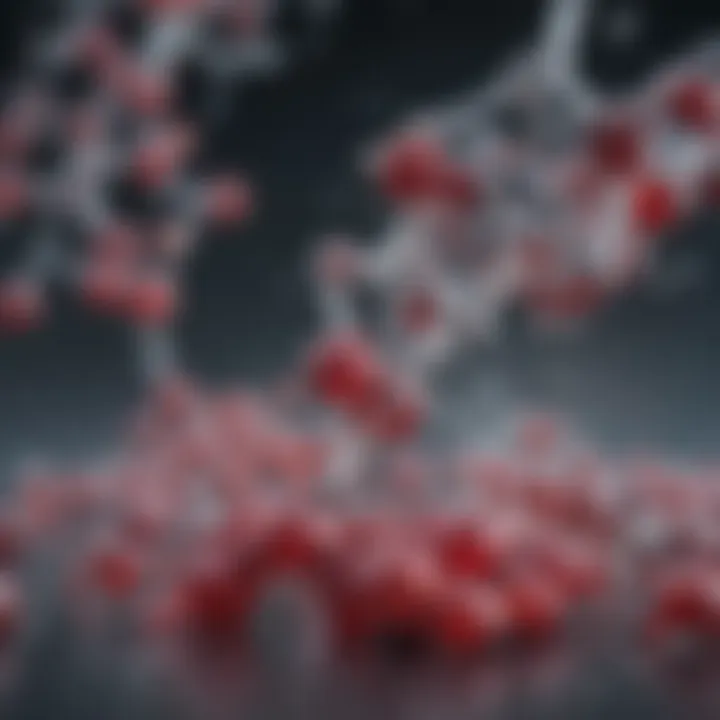
Intro
Glutamate is recognized as a primary neurotransmitter within the central nervous system (CNS). It plays essential roles in numerous physiological and pathological processes. As a key player in neurotransmission, glutamate facilitates communication between neurons and contributes to various functions, such as neural development and memory formation. Understanding glutamate's roles and mechanisms not only sheds light on fundamental neurobiological concepts but also informs the implications of its dysfunction in neurological disorders. This section aims to provide a comprehensive overview of glutamate's significance in both health and disease.
Research Overview
Methodological Approaches
Research on glutamate encompasses a plethora of methodologies to uncover its multifaceted roles. Techniques such as electrophysiology, molecular biology, and imaging are commonly employed.
- Electrophysiology allows for direct measurement of neural activity and synaptic transmission influenced by glutamate. Through this method, researchers can analyze the excitatory post-synaptic potentials (EPSPs) that glutamate generates in neurons.
- Molecular biology techniques, including gene knockout studies, help in understanding the specific roles of glutamate receptors, such as AMPA and NMDA receptors, in various neuronal contexts.
- Imaging techniques such as functional magnetic resonance imaging (fMRI) provide valuable insights into glutamate activity in live subjects, further linking it to cognitive processes.
Significance and Implications
The significance of glutamate in neurobiology cannot be understated. As a primary excitatory neurotransmitter, it is crucial for transmitting signals that guide numerous brain functions, including learning, memory, and overall cognitive processes. Additionally, heightened levels of glutamate can lead to excitotoxicity, a condition that results from overactivation of glutamate receptors and contributes to the pathology of several neurodegenerative diseases such as Alzheimer’s and Parkinson’s disease.
"Glutamate is central to understanding brain function and dysfunction, offering pathways for developing novel therapeutic interventions."
Examining glutamate's actions in various conditions can illuminate potential targets for treatment, establishing its relevance in maintaining neural health and addressing brain disorders.
Current Trends in Science
Innovative Techniques and Tools
Advancements in scientific techniques are pivotal for elucidating glutamate’s roles in vivo and in vitro. Technologies such as optogenetics enable precise control of glutamate signaling in specific neuron populations. This approach allows researchers to dissect the timing and spatial dynamics of glutamate release and receptor engagement, thereby refining our understanding of its role in acute and chronic conditions.
Interdisciplinary Connections
The field of glutamate research increasingly intersects with various domains, including pharmacology, genetics, and computer modeling. Collaboration among disciplines enhances the understanding of complex processes involving glutamate. For instance, the use of computational models can simulate synaptic interactions and predict outcomes of glutamatergic modulation, leading to insightful approaches in therapy for neurodegenerative conditions.
In summary, glutamate’s multipurpose role extends across key neurobiological functions, making it a prominent focus of ongoing research. Enhanced understanding through diverse methodologies continues to underscore its importance in both healthy brain activity and the intricacies of neurological disorders.
Preamble to Glutamate
Understanding glutamate is vital to appreciating its role in the central nervous system. As the primary excitatory neurotransmitter, glutamate significantly influences various neural processes. It is involved in synaptic transmission, neural plasticity, and many cognitive functions. By analyzing glutamate, we can uncover important aspects of brain function and its associated disorders.
Definition of Neurotransmitters
Neurotransmitters are chemicals in the brain that transmit signals across synapses. They play a crucial part in communication between neurons. Each neurotransmitter has a unique role, with some being excitatory while others are inhibitory. Glutamate, in particular, is well-known for its excitatory properties. This means that when glutamate binds to its receptors, it increases the likelihood of a neuron firing an action potential. This function makes it essential for brain activities such as learning, memory, and attention.
Overview of Glutamate
Glutamate is an amino acid that occurs naturally in the body. It is one of the most abundant neurotransmitters in the brain. Glutamate acts on multiple types of receptors, allowing for a wide range of actions and effects. Most notably, it influences synaptic plasticity, which is the ability of synapses to strengthen or weaken over time. This property is fundamental for processes like long-term potentiation, which is critical for learning and memory.
"Glutamate's versatility allows it to play a role in many brain functions, from basic motor coordination to complex cognitive processes."
Furthermore, glutamate levels must be tightly regulated. Imbalances in glutamate can lead to various neurological issues, including excitotoxicity, which can damage neurons. Thus, the sheer importance of glutamate extends beyond its basic neurotransmitter functions. It bridges normal neural activities and the pathological states of the brain.
Biochemical Properties of Glutamate
Understanding the biochemical properties of glutamate is essential in comprehending its diverse roles as a neurotransmitter. Glutamate is the most prevalent excitatory neurotransmitter in the central nervous system. This section elucidates its chemical structure and the mechanisms involved in its synthesis and release. These factors are pivotal, as they influence glutamate's functionality in synaptic transmission and its overarching impact on neural communication.
Chemical Structure of Glutamate
Glutamate is classified as an amino acid, specifically, it is a non-essential amino acid. Its chemical formula is C5H9NO4. The structure consists of a central carbon atom bonded to an amino group, a carboxyl group, a hydrogen atom, and a varying side chain. This side chain is an important characteristic that can influence its interactions with receptors.
The presence of two carboxyl groups in its structure allows glutamate to exist in different ionic forms, depending on the surrounding pH. This property is crucial for its role in neurotransmission since it impacts how glutamate interacts with its corresponding receptors. Additionally, this structure contributes to the neurotransmitter's function as both a building block for proteins and a signaling molecule in the brain.
Synthesis and Release Mechanisms
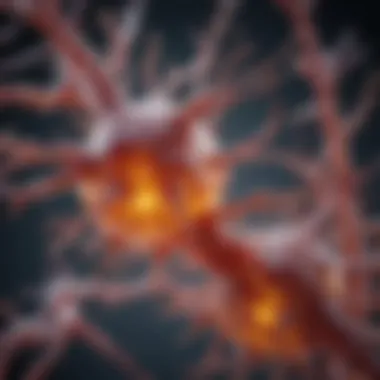
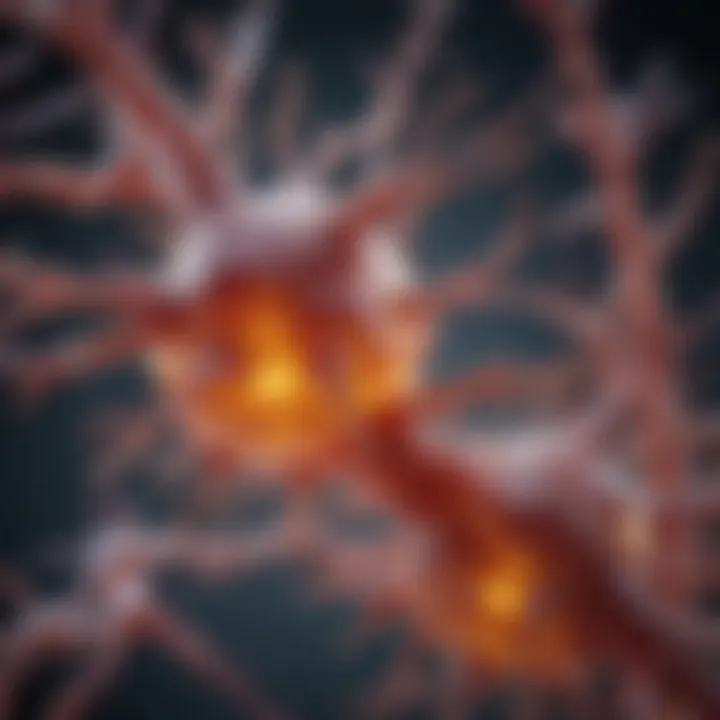
The synthesis of glutamate occurs primarily from glucose metabolism and an active process called transamination. During this process, the amino group from another amino acid, usually from alpha-ketoglutarate, is transferred to a carbon skeleton, forming glutamate. In the brain, glutamine serves as a precursor and is converted to glutamate via the enzyme glutaminase.
Once synthesized, glutamate is stored in synaptic vesicles within presynaptic neurons. Upon neuronal activation, vesicles merge with the presynaptic membrane, releasing glutamate into the synaptic cleft. This release process is calcium-dependent, meaning that an influx of calcium ions triggers the vesicles to fuse with the membrane.
Glutamate's release and uptake are tightly regulated, emphasizing the need for balance. Excess glutamate can lead to neurotoxicity, underlining the importance of meticulous control over its levels in the brain.
After its release, glutamate binds to postsynaptic receptors, initiating a series of events that lead to excitatory neurotransmission. Following this action, glutamate's reuptake into neurons and astrocytes through specific transporters is necessary to terminate its action and maintain homeostasis. This cycle of synthesis, release, and uptake illustrates the finely tuned operation of glutamate in neural communication.
Mechanism of Action
The mechanism of action of glutamate is fundamental to understanding its role as a key neurotransmitter. This topic encompasses how glutamate interacts with receptors and engages secondary messenger systems, which play critical roles in translating chemical signals into physiological responses. Understanding these mechanisms is vital for grasping how glutamate contributes to vital neural functions, including excitatory neurotransmission and synaptic plasticity.
Glutamate Receptors
Glutamate receptors are the primary sites through which glutamate exerts its effects. These receptors are broadly categorized into two main classes: ionotropic and metabotropic receptors.
- Ionotropic receptors are ligand-gated ion channels that mediate fast synaptic transmission. They include three main types: NMDA (N-Methyl-D-Aspartate) receptors, AMPA (Alpha-Amino-3-Hydroxy-5-Methyl-4-Isoxazolepropionic Acid) receptors, and KA (Kainate) receptors. Activation of these receptors allows for the rapid influx of sodium ions, which depolarizes the neuron, increasing the likelihood of action potential generation. NMDA receptors, in particular, are essential for synaptic plasticity and memory formation, responding differently to glutamate based on the voltage across the membrane.
- Metabotropic receptors are G-protein coupled receptors that launch slower, longer-lasting responses through secondary messengers. These receptors are involved in modulating neurotransmission and neuroplasticity. They influence various neuronal processes such as learning, memory, and even neuroprotection under certain conditions.
The intricate nature of glutamate receptor signaling underscores its multifaceted role, especially in conditions like learning and memory where rapid and adaptable synaptic changes are necessary.
Secondary Messenger Systems
After glutamate binds to its receptors, a cascade of intracellular signaling follows. Here, secondary messenger systems are crucial in amplifying and relaying the signals initiated by receptor activation.
- Calcium Signaling: One of the most important secondary messengers that emerges from NMDA receptor activation is calcium (Ca2+). Calcium ions play pivotal roles in various cellular functions, including synaptic plasticity and gene expression. Ca2+ influx subsequently triggers further signaling pathways, affecting numerous cellular mechanisms.
- cAMP Pathway: Cyclic adenosine monophosphate (cAMP) is another significant secondary messenger linked to metabotropic receptor activation. cAMP modulates various physiological processes, including the activity of protein kinase A (PKA). This leads to phosphorylation of target proteins, which can alter neuronal excitability and synaptic strength.
"The dynamic interplay of glutamate receptors and secondary messenger systems illustrates a complex signaling network essential for maintaining neural health and function."
- Phosphoinositides: Another important pathway involves phosphoinositides, which stimulate the release of calcium from the endoplasmic reticulum and activate protein kinase C (PKC). PKC is involved in several processes, such as synaptic modulation and neuroprotection.
In summary, the mechanisms by which glutamate interacts with its receptors and activates secondary messenger systems play a significant role in neurotransmission and plasticity. Understanding these processes offers insights into how glutamate influences not only normal brain function but also pathological states.
Role in Neurotransmission
Understanding the role of glutamate in neurotransmission is essential due to its position as the primary excitatory neurotransmitter in the central nervous system. Its functions extend into vital neural processes such as learning, memory, and overall brain health. This section delves into the specific elements that define glutamate's role in neurotransmission, highlighting the benefits and considerations essential for a comprehensive grasp of this neurotransmitter's significance.
Excitatory Neurotransmission
Glutamate plays a crucial role in excitatory neurotransmission, which is the process of transmitting signals across a synapse that facilitates communication between neurons. When glutamate is released from the presynaptic neuron, it binds to receptors on the postsynaptic neuron. This binding generates an excitatory postsynaptic potential, making it more likely that the postsynaptic neuron will fire.
There are several types of glutamate receptors, including NMDA, AMPA, and kainate receptors. Each receptor type contributes differently to synaptic transmission:
- NMDA receptors are known for their role in synaptic plasticity and memory function. They require both glutamate binding and the presence of depolarization to activate.
- AMPA receptors mediate fast synaptic transmission and are important for rapid signaling.
- Kainate receptors have unique properties and also contribute to excitatory transmission, albeit with different kinetics.
This interplay results in a complex system of excitatory activity that facilitates learning and adaptation in the brain.
Integration with Other Neurotransmitters
Glutamate does not operate within a vacuum; it interacts closely with other neurotransmitters. This integration is vital for creating balanced signaling throughout the brain. For example, glutamate functions alongside gamma-aminobutyric acid (GABA), the principal inhibitory neurotransmitter. The balance between excitatory and inhibitory signals is fundamental to maintaining homeostasis in neural circuits.
Additionally, glutamate influences the activity of several other neurotransmitters:
- Dopamine: In various regions of the brain, such as the prefrontal cortex, glutamate can modulate dopaminergic activity, affecting mood and cognition.
- Serotonin: Research indicates that the serotonergic system interacts with glutamate pathways, playing a role in mood regulation and depression.
- Neuropeptides: Glutamate also impacts the release of neuropeptides that can modify synaptic transmission and neuroplasticity.
Understanding these interactions is crucial, as disturbances in glutamate signaling or in its relations with other neurotransmitters can lead to various neurodevelopmental and psychological disorders.
"The balance of excitatory and inhibitory neurotransmission is essential for maintaining overall brain function and stability."
In summary, the role of glutamate in neurotransmission is foundational to numerous neural operations, including learning, memory, and emotional regulation. Its integration with other neurotransmitters underscores its complexity and importance in neurobiology. This understanding aids in the ongoing research into treatment strategies for disorders stemming from neurotransmitter imbalances.
Glutamate and Neural Development

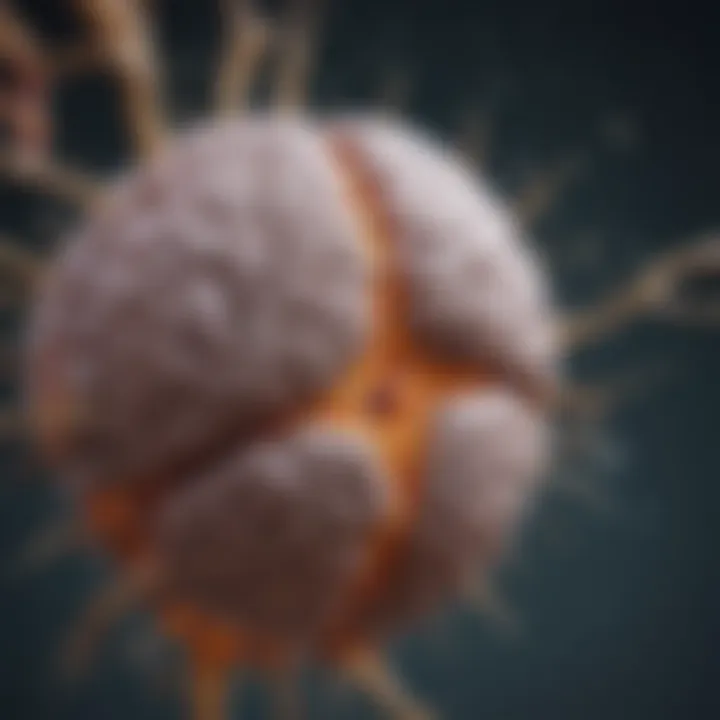
Understanding glutamate's role in neural development is essential for appreciating its significance in the central nervous system. Glutamate not only serves as a neurotransmitter but also has critical involvement in various biological processes that shape the nervous system during development. This section elaborates on how glutamate influences neurogenesis and synaptogenesis, two fundamental processes that contribute to the formation and function of neural networks.
Role in Neurogenesis
Neurogenesis is the process by which new neurons are generated from neural stem or progenitor cells. Glutamate plays a pivotal role in this process by regulating cellular signaling pathways that are essential for cell proliferation and differentiation. During brain development, glutamate levels must be carefully managed; excessive or insufficient glutamate can adversely affect neurogenesis, leading to potential developmental disorders.
Research indicates that glutamatergic signaling promotes the survival of neural progenitor cells. Moreover, the activation of glutamate receptors, particularly NMDA receptors, initiates a cascade of intracellular events that enhance the proliferation of these precursor cells. As the neurotransmitter orchestrates these processes, its modulation can have lasting impacts on brain structure and function.
It's also notable that glutamate is involved in the grooming of neural circuits. By fostering the growth of new neurons and their connections, glutamate contributes significantly to the functioning of the hippocampus, a region associated with learning and memory. Deficits in this process may lead to cognitive impairments, showcasing the importance of this neurotransmitter in healthy brain development.
Influence on Synaptogenesis
Synaptogenesis, the formation of synapses between neurons, is another critical factor in neural development facilitated by glutamate. The process is intricately linked to the activity level of glutamate receptors. Once neurons have differentiated, they form synapses, allowing for communication with other neurons. Glutamate not only promotes synapse formation but also affects the types of synapses that develop.
The presence of glutamate can trigger synaptic plasticity, enabling adjustments in synaptic strength based on activity. This is achieved through long-term potentiation (LTP), an essential mechanism for learning and memory. By enhancing synaptic connections, glutamate supports the dynamic process of refining neural networks, which is vital during developmental phases, especially in early life.
Glutamate also influences the structural changes of synapses, promoting dendritic spine growth, which are small protrusions on neurons where synapses are formed. This growth is critical for increasing the synaptic surface area and allows more efficient communication between cells. In a developing brain, the balance of glutamate signaling is crucial, as both excess glutamate and its deficiency can disrupt the intricacies of synaptic formation, leading to widespread effects on cognitive function.
"Thus, understanding glutamate's dual role as a neurotransmitter and a key player in developmental processes is essential for grasping its overall significance in neural function."
In summary, glutamate is integral to both neurogenesis and synaptogenesis. Its regulation is vital for the proper development of the nervous system and impacts cognitive functions later in life. Continued research in this area may shed light on therapeutic approaches for developmental neurobiological disorders that arise from glutamate dysregulation.
Learning and Memory
Understanding the role of glutamate in learning and memory is crucial. Glutamate is not just an ordinary neurotransmitter; it serves as a fundamental building block for cognitive processes. The interactions in the neural circuits involving glutamate have profound implications for learning abilities and memory retention.
One significant aspect is how glutamate contributes to synaptic plasticity. This is the ability of synapses to strengthen or weaken over time, based on increases or decreases in their activity. Synaptic plasticity is essential for encoding new information and is intricately linked to various forms of memory, including declarative and procedural memory.
Mechanisms of Synaptic Plasticity
Synaptic plasticity is facilitated primarily through processes like long-term potentiation (LTP) and long-term depression (LTD). These mechanisms are vital for neural learning and the adaptation of synaptic strength based on experience. During LTP, a burst of glutamate binds to its receptors, causing an influx of calcium ions into the postsynaptic neuron. This leads to a cascade of intracellular events that strengthen the synaptic connection.
On the flip side, LTD involves a prolonged low-frequency stimulation of synapses, resulting in a decrease in synaptic strength. Both mechanisms allow the brain to encode information efficiently. The balance between LTP and LTD optimization is necessary for accurate learning and memory formation, as it adjusts how signals are processed.
Roles of Long-Term Potentiation (LTP)
Long-Term Potentiation (LTP) plays a significant role in learning and memory. LTP can be thought of as the brain’s way of marking important information for easy retrieval in the future. For instance, when a person learns something new, repeated activation of a specific synapse reinforces its strength.
Research indicates that LTP is involved not just in basic memory formation but also in higher cognitive functions. Studies show that various conditions, such as enriched environments or repeated learning experiences, can enhance LTP. This suggests that our environment and experiences significantly shape cognitive capacities through the modulation of glutamate pathways.
In summary, glutamate's influence extends beyond simple synaptic transmission. It is a key player in shaping how memories are formed and recalled. Understanding glutamate’s role in these processes has implications for educators and researchers involved in cognitive science, as it provides insights into optimizing learning environments and therapies for memory-related disorders.
Glutamate and Pathological States
The topic of glutamate in pathological contexts is of significant relevance. Understanding how glutamate contributes to various disorders provides insight into potential treatments and therapeutic strategies. This section delves into the detrimental effects of glutamate overactivation and its association with numerous neurodegenerative conditions. In doing so, it highlights the critical balance required in glutamatergic signaling, emphasizing both risks associated with its dysregulation and the potential for intervention.
Overactivation and Neurotoxicity
Glutamate is the principal excitatory neurotransmitter in the central nervous system. Although its role is crucial in normal neuronal function, excessive activation of glutamate receptors can lead to neurotoxicity. This phenomenon, often termed excitotoxicity, primarily occurs when there is an overabundance of glutamate in the synaptic cleft. Such an imbalance experiences several forms of neuronal damage, due primarily to the excessive influx of calcium ions into neurons. High levels of calcium can trigger cell death pathways, ultimately leading to apoptosis or necrosis.
The conditions under which glutamate overactivation occurs are diverse. One of the most well-documented scenarios is during ischemia, where the blood supply to an area of the brain is compromised. Under these conditions, neuronal cells undergo stress, prompting the release of more glutamate, worsening the excitotoxic cascade. Other triggers include various forms of trauma, seizures, or even prolonged stress, situating the individual at risk of permanent damage.
"Neurotoxicity associated with glutamate requires urgent attention, as it implies lasting deficits in brain function and cognitive abilities."
The systematic understanding of excitotoxicity not only helps identify at-risk populations but also opens avenues for targeted therapies. Compounds that act as glutamate antagonists, like ketamine and memantine, have shown efficacy in alleviating symptoms in specific disorders. Although these agents offer promise, ongoing research is essential to delineate their risks and benefits thoroughly.
Association with Neurodegenerative Diseases
Neurodegenerative diseases such as Alzheimer's, Parkinson's, and Huntington's are noticeably associated with glutamate dysregulation. In these conditions, the normal processes governing glutamate homeostasis are disrupted. It results in either insufficient or excessive levels of glutamate, which can significantly impact neuronal survival and functionality.
In Alzheimer's disease, for instance, the pathogenesis involves the accumulation of amyloid-beta plaques. These plaques are believed to interfere with normal glutamatergic signaling, leading to increased neuronal excitability and damage. The progressive degeneration of cholinergic neurons further exacerbates the issue, creating a vicious cycle that enhances glutamate release and its subsequent neurotoxic effects.
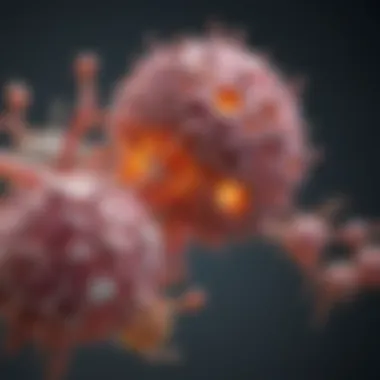
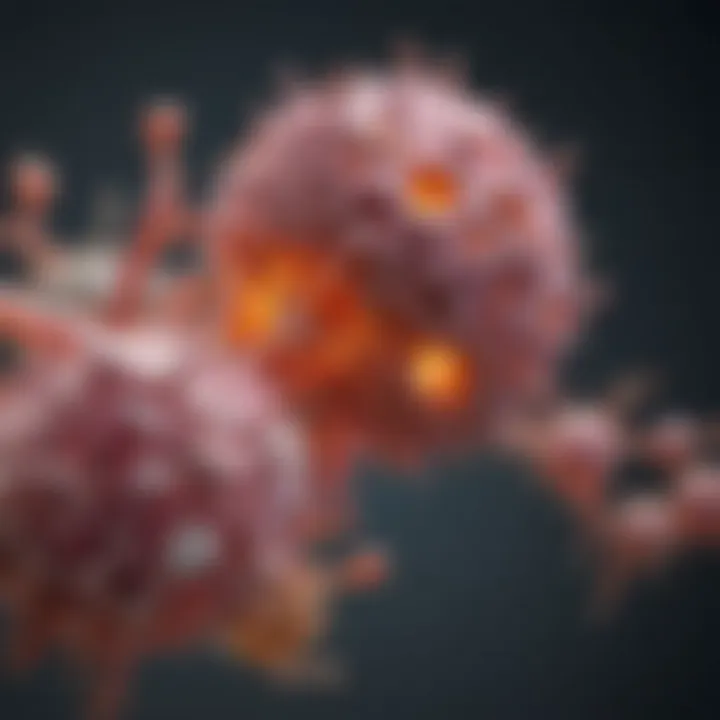
Similarly, in Parkinson's disease, the death of dopaminergic neurons elevates glutamate levels, contributing to motor deficits. The neurotoxic role of glutamate has led to the exploration of glutamate-modulating therapies for these diseases. These treatments are in varying stages of research and clinical application.
Understanding the pathophysiological mechanisms underlying these diseases informs the development of new strategies. Highlighting the significance of maintaining proper glutamate levels underscores the necessity for ongoing investigations into the interplay between glutamate and neurodegeneration.
Glutamate in Mental Disorders
Understanding the role of glutamate in mental disorders provides valuable insights into the complexities of these conditions. Its influence extends not only to neurotransmission but also to how neural circuits are oriented during various mental health challenges. Glutamate dysregulation can affect mood, perception, and cognitive functions, thus becoming a focal point in the study of several mental health diseases.
Role in Schizophrenia
Schizophrenia is a severe mental disorder characterized by disruptions in thought processes, perceptions, and emotional responsiveness. Research indicates that glutamate is significantly involved in the pathophysiology of this condition. Abnormal glutamate signaling, particularly at the N-Methyl-D-Aspartate (NMDA) receptor, is proposed to contribute to the symptoms of schizophrenia.
Evidence suggests that hypofunction of NMDA receptors can lead to the cognitive deficits and disorganized thinking often seen in patients. The glutamate hypothesis posits that reduced glutamatergic function results in an excitatory-inhibitory imbalance in the brain. This imbalance contributes to hallucinations and delusions.
"Many researchers are now focusing on developing medications that target glutamatergic pathways to improve treatments for schizophrenia."
Also, the potential of glutamate modulators, like glycine and D-serine, is under investigation for their ability to enhance NMDA receptor function. These agents might hold promise for alleviating some of the cognitive symptoms that no traditional antipsychotic agents address effectively.
Implications in Depression
Depression is another mental disorder closely associated with glutamate. Studies reveal that changes in glutamate levels can influence mood and emotional regulation. Elevated glutamate in the cerebrospinal fluid has been observed in some individuals with depression.
Emerging evidence points to glutamate’s role in synaptic plasticity and neurogenesis, processes that are often disrupted in depression. Medications that enhance glutamate transmission, such as ketamine, have gained attention for their rapid antidepressant effects.
By antagonizing NMDA receptors, ketamine is thought to facilitate an increase in synaptic glutamate, leading to improved mood and cognitive function. This mechanism has opened new avenues for understanding depression and its treatment, often requiring a shift away from conventional methods.
As ongoing research continues to refine our understanding, the therapeutic targets of glutamate modulation appear increasingly relevant. Addressing glutamate dysregulation may pave the way for effective treatments for both schizophrenia and depression, ultimately improving outcomes for patients who struggle with these debilitating disorders.
Therapeutic Approaches Targeting Glutamate
The therapeutic approaches targeting glutamate are essential in addressing a range of neurological and psychiatric disorders. Glutamate is the primary excitatory neurotransmitter in the central nervous system. Its dysfunction can contribute to various conditions, including epilepsy, schizophrenia, and Alzheimer’s disease. By understanding and manipulating glutamatergic systems, researchers aim to develop effective treatments. This section examines specific therapeutic strategies, highlighting the significance of glutamate antagonists and exploring future directions for treatment.
Glutamate Antagonists
Glutamate antagonists present a unique approach in treating conditions associated with excess glutamate activity. These substances inhibit the action of glutamate at its receptors, aiming to reduce excitotoxicity—a process where excessive glutamate leads to neuronal damage.
Examples include:
- Memantine: Used primarily in Alzheimer’s disease. Memantine blocks NMDA receptors, slowing down cognitive decline by preventing excessive calcium influx into cells.
- Ketamine: Originally an anesthetic, it has emerged as a rapid-acting antidepressant. Its NMDA antagonism may help restore synaptic function and alleviate depressive symptoms in treatment-resistant cases.
- Riluzole: Mainly for amyotrophic lateral sclerosis (ALS). Riluzole decreases glutamate release and protects neurons.
These antagonists serve as a critical tool in the management of conditions where glutamate plays a pathological role. However, careful consideration is necessary. Potential side effects, such as cognitive disturbances or anesthetic properties, require monitoring during treatment.
Future Directions in Treatment
As our understanding of glutamate's role in the nervous system evolves, new therapeutic avenues are emerging. The goal is to develop more targeted and effective treatments with fewer side effects. Research is currently focusing on:
- Novel Receptor Modulators: Exploring compounds that selectively modulate specific glutamate receptors, which may provide therapeutic benefits without the drawbacks associated with broad antagonists.
- Combination Therapies: Integrating glutamate modulation with other treatments, such as cognitive-behavioral therapies, or using it alongside other pharmacological agents, to create holistic treatment plans for mental disorders.
- Biomarker Development: Identifying biomarkers related to glutamate levels can aid in tailoring treatments to individual patient needs, potentially improving outcomes.
- Longitudinal Studies: Conducting long-term studies to assess the efficacy and safety of glutamate-targeting therapies allows for deeper understanding and better optimization of treatments.
The ongoing research in these areas is promising. The aim is not only to alleviate symptoms associated with glutamate dysregulation but also to enhance the overall quality of life for patients affected by neurological conditions. Continued focus on the mechanisms and effects of glutamate in health and disease will undoubtedly lead to better therapeutic strategies.
Closure
In the context of this article, the conclusion serves as a crucial synthesis of the multifaceted role of glutamate. It brings together the various threads explored throughout the discovery of glutamate as a key neurotransmitter. Understanding glutamate's biochemical properties, mechanisms of action, and its critical involvement in processes such as learning, memory, and neurodevelopment is essential for appreciating its overall significance in the central nervous system.
The exploration of glutamate also highlights the implications of its dysfunction. This is particularly evident in neurodegenerative diseases and mental disorders, where the delicate balance of excitatory transmission can lead to serious health issues. The conclusion emphasizes how advancing our understanding of glutamate may pave the way for novel therapeutic approaches and treatments that could target these disturbances.
Overall, the conclusion underscores that both basic and applied research on glutamate should remain at the forefront to fully elucidate its complexities and potential.
Summary of Key Points
- Glutamate is the principal excitatory neurotransmitter in the central nervous system.
- It plays a vital role in neural development, affecting neurogenesis and synaptogenesis.
- Glutamate mediates vital processes such as learning and memory through mechanisms like long-term potentiation.
- Its overactivation is linked to neurotoxicity and various neurodegenerative diseases.
- Glutamate is intricately involved in mental disorders, influencing conditions such as schizophrenia and depression.
- Therapeutic strategies targeting glutamate receptors and pathways represent promising areas of research.
Importance of Continued Research
Continued research into glutamate is critical for several reasons. First, elucidating its complex signaling pathways may yield insights into various neurological conditions. As the understanding of mechanisms of action deepens, so too does the potential for developing specific interventions that could alleviate conditions linked to glutamate dysregulation.
Furthermore, researching glutamate will contribute to a more comprehensive understanding of synaptic plasticity, thereby enriching knowledge about learning and memory processes. This has implications not only for treating disorders but also for enhancing cognitive function in healthy individuals.