Understanding Microchannels: An In-Depth Exploration
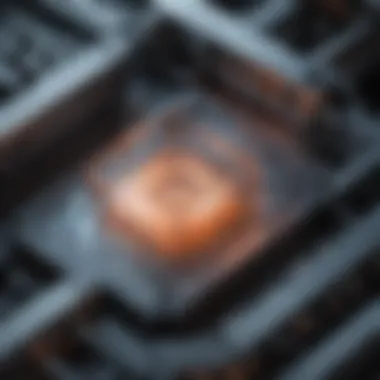
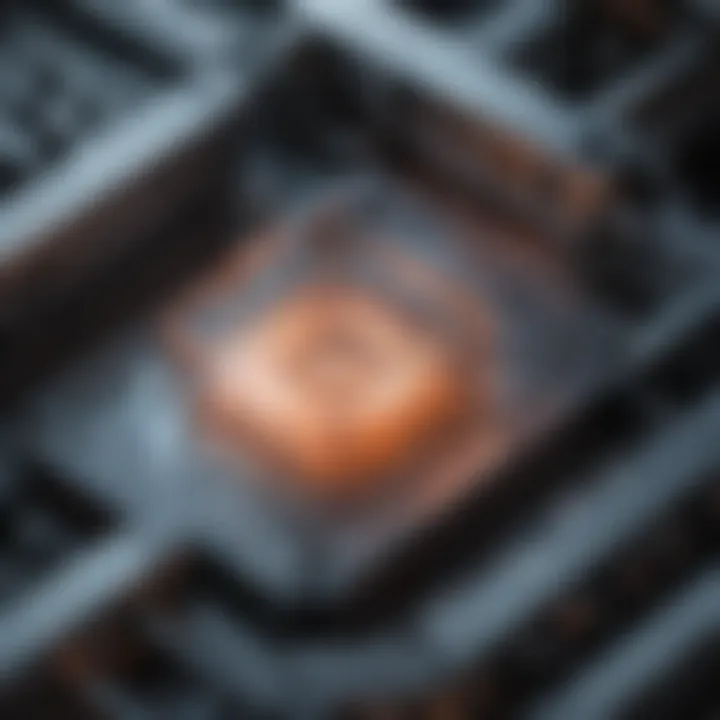
Intro
Microchannels represent a fascinating area of study in fluid dynamics and engineering. As small-scale structures, they play a crucial role in various scientific disciplines, facilitating processes that are essential for advancements in technology and healthcare. This article aims to shed light on the intricacies of microchannels, covering their design, applications, and the challenges that lay ahead. Understanding microchannels will not only enhance our grasp of physical sciences but also inspire innovations in fields like biomedical engineering, environmental science, and nanotechnology.
Research Overview
Microchannels are defined by their precise, micrometer-sized dimensions that control fluid flow. This section examines the methodological approaches to studying microchannels, as well as their significance in today’s scientific landscape.
Methodological Approaches
The study of microchannels often involves a combination of experimental and computational techniques. Researchers utilize high-resolution imaging methods to visualize flow characteristics within these channels. Techniques such as micro-particle image velocimetry (µ-PIV) and laser-induced fluorescence (LIF) allow for detailed observation of fluid dynamics. Moreover, computer simulations, including computational fluid dynamics (CFD), provide powerful tools for predicting flow behaviors under varied operational conditions.
- Experimental Techniques:
- Computational Techniques:
- High-speed imaging
- Micro-PIV
- LIF
- CFD simulations
- Finite element analysis
Significance and Implications
The implications of microchannels extend beyond simple fluid transport. They enable innovations in diagnostic tools, such as lab-on-a-chip devices, which streamline complex processes. This compactness allows for significant reductions in reagent quantities and analysis time. Microchannels are also vital in biotechnology, aiding in the development of more efficient drug delivery systems.
"Understanding and harnessing microchannel technology can lead to breakthroughs in medical diagnostics and treatment."
Current Trends in Science
In recent years, the study of microchannels has gained momentum, driven by advancements in material science and engineering techniques. This section discusses the innovative tools and interdisciplinary connections shaping the research landscape.
Innovative Techniques and Tools
The emergence of advanced additive manufacturing technologies has expanded the possibilities for designing microchannels. Techniques such as 3D printing and soft lithography enable the creation of complex channel geometries. These methods facilitate customization, allowing researchers to tailor microchannels for specific applications, such as enhancing heat transfer or optimizing reaction kinetics in chemical processes.
- 3D Printing:
- Soft Lithography:
- Rapid prototyping of microchannel designs
- Custom geometries tailored to applications
- Creating flexible microchannel systems
- Ideal for biomedical applications
Interdisciplinary Connections
The significance of microchannels resonates across various disciplines, including chemistry, physics, and engineering. Their applications in analytical chemistry highlight how these structures can enhance detection limits and improve separation efficiency. Collaboration among disciplines can foster new insights and accelerate development, leading to solutions for complex challenges faced in environmental science and healthcare.
Prologue to Microchannels
Microchannels are increasingly pivotal in various fields of science and engineering. Their designs are unique, promoting fluid flow and heat transfer in efficient ways. The exploration of microchannels plays a vital role in enhancing technologies ranging from biomedical applications to environmental monitoring. Understanding microchannels provides insights not only into their functionalities but also their implications in innovation and technological advancements.
Definition and Overview
Microchannels are defined as tiny channels with dimensions typically in the range of microns to a few millimeters. These structures facilitate the precise control of fluid movements and reactions, making them essential in various applications. Their geometries can be tailored based on the requirements of specific processes. The uniqueness of microchannels lies in their ability to enhance surface area while minimizing volume, which is crucial in numerous scientific dependencies. Key benefits of microchannels include improved heat transfer efficiency and reduced energy consumption. They are often employed in systems where space and energy are at a premium.
Historical Context
The concept of microchannels emerged from the need for efficient systems in chemical processing and thermal management. Their genesis can be traced back to advancements in microfabrication techniques during the late 20th century. Initially, microchannels found applications in microelectronics and cooling systems. Over time, researchers began to recognize their potential in biomedicine and environmental science. This broader interest led to innovations in designs and materials used in fabricating microchannels, ultimately expanding their applications.
Understanding the journey of microchannels helps contextualize their current significance and future potential. The exploration of their historical development reveals shifts in technology and application, setting the stage for ongoing research and improvements.
Microchannels combine versatility with efficiency, shaping future technologies and methodologies.
Fundamental Principles
The fundamental principles of microchannels are essential for understanding their design, functionality, and applications. Microchannels play a crucial role in various fields, such as biomedical engineering and chemical processing, where efficiency and precision are paramount. Grasping these principles allows researchers and practitioners to better harness the advantages of microchannel technology.
Engineering Basics
Microchannels are typically defined by their small dimensions, often ranging from a few micrometers to a few millimeters. Their design focuses on maximizing surface area while minimizing volume, leading to enhanced interaction between fluids and surfaces.
Understanding the engineering basics involves grasping key concepts such as channel geometry, flow rates, and surface properties. Different shapes including rectangular, circular, or complex geometries can alter fluid behavior within the channel.
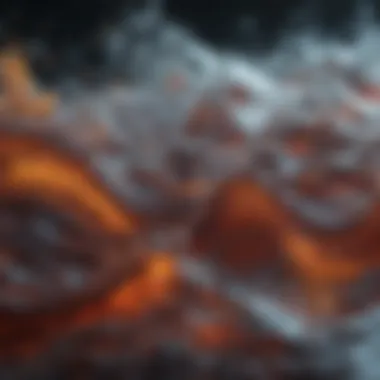
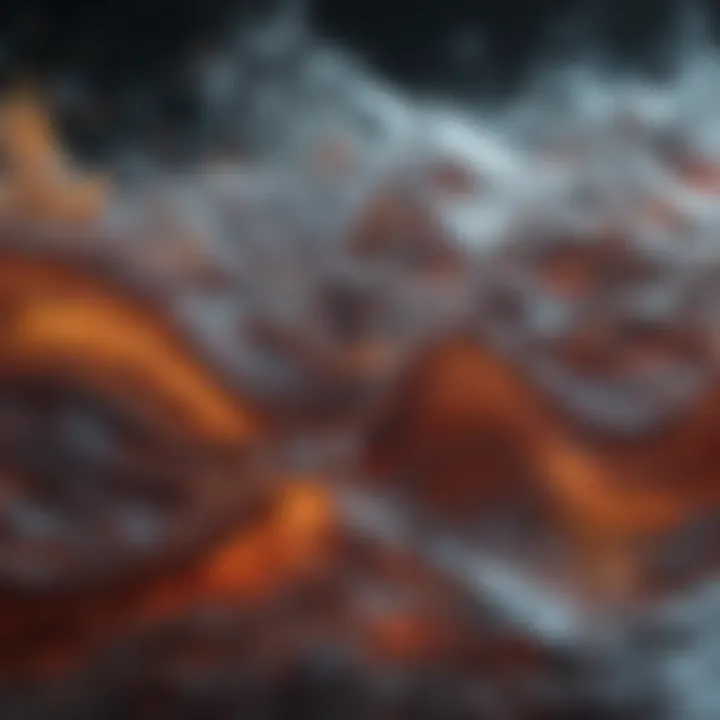
A critical aspect of the engineering process is material selection. The materials used must withstand the conditions of their application, be it chemical interactions or high temperatures. Polymers, metals, and ceramics are commonly utilized, with each offering unique properties conducive to specific applications.
Fluid Dynamics in Microchannels
Fluid dynamics is a fundamental area within the study of microchannels. The movement of fluids in such confined spaces differs significantly from that observed in larger systems. One critical aspect is the dominance of laminar flow in microchannels. In laminar flow, fluid moves in parallel layers, minimizing turbulence and allowing for predictable behavior, which is vital for analytical precision.
Key principles in fluid dynamics include continuity, momentum, and energy equations. These principles facilitate a deep understanding of how fluids behave under various conditions. For example, Poiseuille's law describes the relationship between flow rate and pressure drop in a channel, emphasizing the impact of channel diameter and fluid viscosity on performance.
Furthermore, the Nusselt number provides insight into heat transfer characteristics within microchannels, enabling designers to predict the efficiency of thermal management systems.
Heat Transfer Mechanisms
Heat transfer in microchannels is another critical subject. The small size of these channels results in high surface-to-volume ratios, promoting efficient heat transfer. Microchannels can operate on principles of conduction, convection, and radiation, but primarily rely on convection due to the flow of fluids within them.
In forced convection, the motion of the fluid enhances heat transfer rates, which is crucial in applications like electronic cooling systems. Understanding the mechanisms, such as single-phase or two-phase flow, aids in designing systems that optimize thermal performance.
Overall, the integration of heat transfer theory with fluid dynamics forms the backbone of effective microchannel design. This knowledge is vital for advancing applications across various scientific and engineering disciplines, ensuring that systems operate at maximum efficiency.
Understanding both fluid dynamics and heat transfer is necessary for innovative designs in microchannel technology.
Thus, mastering these fundamental principles equips engineers and scientists with the tools needed to innovate and improve microchannel applications.
Types of Microchannels
Microchannels come in various shapes and configurations. Each type serves distinct purposes, impacting their applications across different fields. Understanding these types is crucial for selecting the right microchannel design as it significantly influences fluid flow characteristics, heat transfer efficiency, and overall system performance. The design's geometry can dramatically affect how fluids behave at such small scales. This section will review the main types of microchannels: rectangular, circular, and those with complex geometries.
Rectangular Microchannels
Rectangular microchannels are among the most commonly fabricated types in microfluidics. They are often preferred due to their simplicity in design and ease of manufacturing. The rectangular shape allows for a larger surface area per unit volume, promoting efficient heat transfer. Their straightforward geometry makes them suitable for high-precision applications, such as biomedical devices and chemical reactors. Additionally, these channels can accommodate various flow rates, which is vital for applications requiring precise control over fluid dynamics.
Key advantages of rectangular microchannels include:
- Flexibility in Design: They can be easily adapted to different sizes and arrangements, fitting specific experimental setups or industrial needs.
- Enhanced Heat Transfer: The geometry allows for better thermal management, a critical factor in many applications.
However, there are also some challenges. For instance, the risk of clogging is more pronounced in straight rectangular channels, especially when dealing with particulates in fluids. This limitation necessitates careful consideration in design choices based on the intended application.
Circular Microchannels
Circular microchannels provide an alternative design that can result in different fluid dynamic behaviors compared to rectangular types. The circular cross-section minimizes wall effects, resulting in a more uniform flow profile. This property makes them particularly useful in applications where mixing and reaction rates are essential, such as in continuous flow reactors.
Benefits of circular microchannels include:
- Reduced Pressure Drop: The smooth, rounded geometry allows for lower resistance to fluid flow, which is vital for applications requiring high flow rates without excessive energy consumption.
- Improved Mixing: Circular channels often enhance mixing properties due to their shape, making them suitable for chemical processing applications.
Some considerations with circular microchannels entail their slightly more complex fabrication techniques compared to rectangular ones. The manufacturing methods and the materials may impact the final performance of these channels.
Complex Geometries
As technology advances, the need for more intricate designs becomes clear. Complex geometries in microchannels allow for tailored fluid dynamics properties, enhancing the capability to control various processes in real-time. These designs can integrate features like bends, branches, and coiled structures to facilitate specific flow patterns or reaction conditions.
Advantages of complex geometries:
- Customization: They can be tailored to meet precise operational requirements by modifying the flow path or introducing localized effects.
- Enhanced Performance: A well-designed complex channel can significantly improve mixing and heat transfer over traditional channel shapes.
However, the fabrication of such microchannels often poses challenges. The complexity can result in higher costs and more intricate production processes. Additionally, the risk of fouling and clogging may increase with added features. Careful design and material selection can mitigate these potential issues.
Fabrication Techniques
The fabrication of microchannels is a critical aspect of their development and application. It involves various methods that convert design concepts into physical microchannel structures. Each technique possesses unique advantages and challenges. Understanding these techniques is essential for researchers and professionals interested in the microchannel field.
Effective fabrication influences the performance of microchannels in terms of fluid dynamics, heat transfer, and chemical reactions. Choosing the right fabrication technique can optimize these parameters. Some methods allow for higher precision, while others provide quicker production times or lower costs. Let’s delve deeper into three key fabrication techniques used in microchannel creation: photolithography, soft lithography, and micro-milling with 3D printing.
Photolithography
Photolithography has long been the cornerstone of microchannel fabrication. This method uses light to transfer geometric patterns onto a substrate. It starts with applying a light-sensitive material called photoresist onto the surface. Specific areas are then exposed to ultraviolet (UV) light, which causes chemical changes in the exposed parts. Following exposure, the substrate is developed, removing either the exposed or unexposed photoresist depending on the type of resist used.
One advantage of photolithography is the ability to create intricate microchannel designs with high precision. This is particularly valuable when the dimensions are in the sub-micron range. Additionally, photolithography allows for batch processing, making it efficient for producing large numbers of microchannels simultaneously.
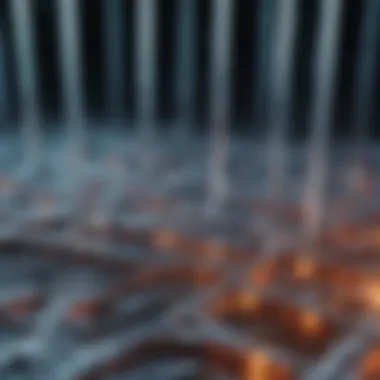
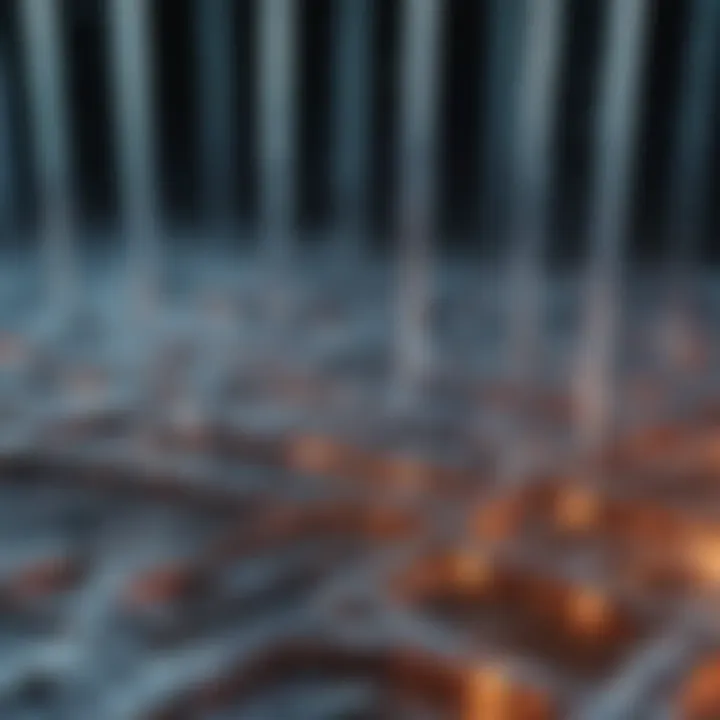
Soft Lithography
Soft lithography is another prominent technique used for microchannel fabrication. This method utilizes elastomeric materials, typically polydimethylsiloxane (PDMS), to create molds from original templates. The process involves casting the PDMS against a master that holds the desired channel patterns. Once cured, this elastomeric mold can be used to replicate microchannel structures on various substrates.
One of the main benefits of soft lithography is its versatility. It can be applied to different materials, including glass, silicon, and plastics. Also, this technique often requires less sophisticated equipment compared to photolithography, making it more accessible for laboratories with limited resources. However, while soft lithography is excellent for prototyping, it may not achieve the same level of precision as photolithography.
Micro-Milling and 3D Printing
Micro-milling and 3D printing are emerging fabrication techniques that provide additional options for constructing microchannels. Micro-milling involves mechanically removing material using small cutting tools, allowing for precise manufacturing of microstructures directly on solid substrates. This method achieves high geometric fidelity and can handle a range of materials from metals to polymers.
On the other hand, 3D printing provides a rapid prototyping solution. It allows for the creation of complex geometries in a layer-by-layer fashion. This is particularly advantageous for customized applications or geometries that are difficult to achieve using traditional methods. Furthermore, 3D printing can reduce fabrication time and material waste, making it an attractive option for many researchers.
"The choice of fabrication technique can significantly influence the success of microchannel applications. Understanding the strengths and limitations of each method is vital for optimizing design and functionality."
Applications of Microchannels
Microchannels have become a significant area of research and application across various fields. Their ability to manipulate fluids at the microscale opens up opportunities for enhanced efficiency and performance. In diverse domains like biomedicine, chemical processing, and environmental monitoring, microchannels provide unique advantages. They facilitate precise control over fluid dynamics, allowing for improved heat transfer and reaction rates in numerous applications.
In biomedical areas, the ability to perform diagnostics quickly is crucial. For chemical processing, continuous flow systems allow reactions to occur with high efficiency. Environmental monitoring benefits from microchannels through precision sampling and analysis. Thus, the applications of microchannels not only highlight their versatility but also point to their transformative potential in modern science and technology.
Biomedical Applications
Point-of-care Diagnostics
Point-of-care diagnostics utilizes microchannels to provide rapid test results near the patient. This technique is critical in emergencies or settings where lab access is limited. The key characteristic of point-of-care diagnostics lies in its ability to simplify procedures, often integrating sample collection, analysis, and result interpretation into a single device.
One major advantage is the reduced time for decision-making, critical for treatment initiation. These systems also require smaller sample volumes, which is advantageous for pediatric or geriatric patients. However, the resolution of sensitive tests can vary, leading to a potential trade-off between speed and accuracy despite their growing popularity in healthcare settings.
Drug Delivery Systems
Drug delivery systems employing microchannels enable targeted therapies with controlled release mechanisms. These systems showcase the potential to enhance drug effectiveness and minimize side effects. A primary feature of drug delivery via microchannels is the ability to achieve localized therapeutic concentrations without systemic exposure.
This specificity makes microchannels popular in personalized medicine, supporting tailored treatments based on individual responses. Nonetheless, challenges exist, such as maintaining stability of drugs during transportation and ensuring consistent dosage. Understanding these factors is vital to optimizing their application in clinical settings.
Chemical Processing
Continuous Flow Reactors
Continuous flow reactors utilize microchannels to maintain reactions in a steady state, allowing for higher conversion rates and improved heat management. These reactors facilitate more manageable temperature and pressure conditions compared to batch processes. A significant aspect is their ability to scale up processes efficiently, which is a critical consideration in industrial applications.
The flexibility in adjusting reaction conditions in real time can lead to more efficient chemical processes. However, the design of such systems may present complexities, requiring rigorous optimization to ensure desired outcomes.
Microreactors
Microreactors are compact systems that enable the synthesis of chemicals at a microscale. They are notable for their enhanced control over mixing and reaction conditions. The key characteristic of microreactors is their ability to execute multi-step reactions with minimal space and resources.
This efficiency makes microreactors particularly beneficial for fine chemicals and pharmaceutical production. However, scaling from lab to commercial production can pose challenges. The integration of microreactors into existing workflows needs careful evaluation.
Environmental Monitoring
Microchannels play a pivotal role in environmental monitoring, enabling precise analysis of contaminants or chemical properties in samples. Their small dimensions allow for the rapid transport and analysis of minute quantities of fluids, which is vital for detecting pollutants quickly. This precision can lead to faster responses to environmental issues. Microchannels also enable the design of portable monitoring systems, making field analysis feasible.
Overall, applications of microchannels represent a significant area of growth and innovation. From healthcare to industrial and environmental uses, their capacity to enhance performance and efficiency continues to shape the future of these fields.
Challenges in Microchannel Technology
Microchannels present unique advantages, but they also come with considerable challenges. Recognizing these obstacles is crucial for advancing microchannel applications and ensuring their effectiveness across diverse fields. Addressing issues such as clogging, fouling, and material limitations is essential for optimizing their designs and enhancing performance.
Clogging and Fouling
Clogging occurs when particles or substances accumulate within the microchannels, obstructing fluid flow. A common factor in clogging is the small dimensions of microchannels that can lead to easily blocked pathways. This issue can significantly disrupt processes in biomedical applications, such as drug delivery systems, where continuous flow is imperative.
Fouling further compounds the problem. It refers to the unwanted accumulation of materials on the channel surfaces. Fouling can affect heat transfer and chemical reactions, forcing adjustments in operational parameters. Factors that contribute to fouling include the nature of the fluids being transported and the surface properties of the microchannels.
To mitigate clogging and fouling, several strategies can be employed:
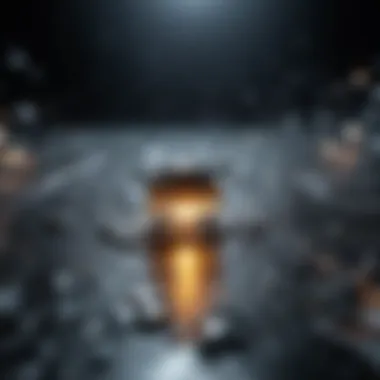
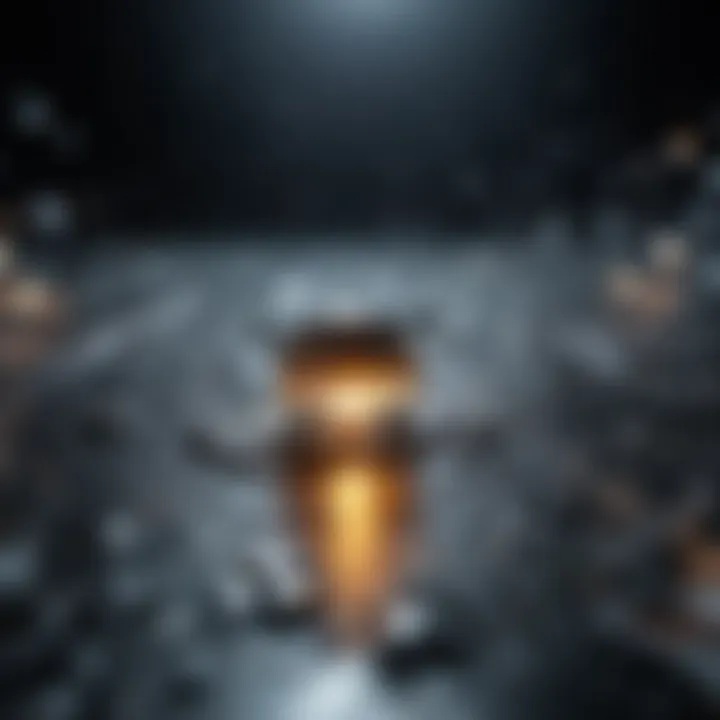
- Surface modifications: Enhancing the wettability of channel surfaces can reduce adhesion of particles.
- Nanofluid utilization: Introducing engineered fluids with improved transport properties may help maintain flow consistency.
- Periodic cleaning cycles: Implementing self-cleaning mechanisms can reduce downtime and maintain performance.
"Addressing fouling and clogging is central to harnessing the full potential of microchannel technology, especially in critical applications where reliability and efficiency are paramount."
Material Limitations
The selection of materials for constructing microchannels plays a pivotal role in their effectiveness and durability. Various materials like silicon, glass, and polymers are used in fabrication, each possessing distinct advantages and limitations. One major concern is the thermal stability of these materials. In applications involving significant heat transfer, the material must maintain structural integrity and performance.
Moreover, chemical compatibility poses another challenge. Microchannels often transport a variety of fluids including corrosive substances. It is essential to choose materials that withstand such conditions without degradation. As the field continues to evolve, the need for advanced materials has become apparent. New composites and hybrid materials are under investigation to enhance both mechanical properties and compatibility with diverse environments.
Some considerations regarding materials include:
- Thermal conductivity: Selecting materials that efficiently transfer heat can optimize thermal management in microchannels.
- Corrosion resistance: Developing materials that resist chemical attack is vital for longevity.
- Manufacturing processes: The chosen material should allow for precise fabrication techniques so that microchannel dimensions remain consistent.
In summary, recognizing and addressing the challenges related to clogging, fouling, and material limitations is essential for the future development of microchannel technology. By tackling these issues, engineers and researchers can push the boundaries of what microchannels can achieve in their respective fields.
Future Directions
The future of microchannel technology holds great potential, driven by innovation and the merging of various scientific disciplines. As researchers continuously explore new materials and applications, it is vital to understand the emerging trends and the key changes expected in this field. The integration of microchannels with the Internet of Things (IoT), advancements in material sciences, and their applications in nanotechnology represent critical directions of development. These areas not only enhance the performance of existing microchannel systems but also lead to entirely new possibilities that could redefine industries.
Integration with IoT
Connecting microchannel technology with the Internet of Things provides unprecedented control and monitoring capabilities. Smart microchannels fitted with sensors can collect real-time data on fluid dynamics, temperature, and pressure. This integration allows for greater automation and efficiency in processes across industrial and biomedical applications. For instance, in the health sector, microchannel devices that monitor blood flow in real-time can potentially offer crucial insights into patient conditions.
The benefits of such integration are multifold:
- Enhanced Data Collection: Real-time monitoring supports better decision-making based on accurate and immediate data.
- Process Optimization: IoT can help in fine-tuning the operating conditions of microchannel systems, enhancing their efficacy.
- Remote Monitoring: With IoT, users can manage and troubleshoot microchannel systems from a distance, saving time and resources.
As IoT technology continues to evolve, its applications within microchannels will likely expand significantly, creating smarter and more efficient solutions to complex problems.
Advancements in Materials
The material used in microchannel fabrication is paramount to their performance and longevity. Innovations in material sciences promise to create better, more durable substances that withstand erosive fluids and high temperatures. For example, the development of advanced polymers and composites can lead to microchannels that reduce fouling and clogging, thereby enhancing reliability in continuous flow conditions.
Additionally, new coatings can be employed to improve heat transfer performance and chemical resistance. Some critical advancements are:
- Functionalized Surfaces: Treating materials to create specific chemical interactions can lead to better fluid handling and reduced fouling rates.
- Nano-coatings: Utilizing nanotechnology to create thin films that improve performance without adding bulk can be a game-changer.
- Biocompatible Materials: In biomedical applications, ensuring that materials do not provoke an unwanted response in biological systems is essential. Innovations here greatly enhance potential applications.
Ultimately, advancements in materials will drive innovation, allowing for the design of more efficient, effective, and durable microchannel systems.
Microchannels in Nanotechnology
The relationship between microchannels and nanotechnology represents a frontier in engineering and applied sciences. Microchannels can serve as platforms for nanomanipulation, allowing for precise control of fluids at the nanoscale, which is essential in various applications, including drug delivery and chemical analysis.
Some important aspects of this relationship include:
- Nanofluidics: Understanding fluid behavior at the nanoscale opens pathways to new diagnostic tools and therapeutic modalities.
- Enhanced Reaction Kinetics: By manipulating reactions on the nanoscale within microchannels, researchers can achieve desired outcomes with increased speed and precision.
- Integration in Chips: The development of microfluidic chips that incorporate nanotechnology is leading to significant improvements in point-of-care diagnostics and lab-on-a-chip systems.
The synergy between microchannels and nanotechnology is paving the way for innovative applications poised to revolutionize approaches in many scientific fields, including biomedicine, environmental science, and materials testing.
In summary, exploring future directions in microchannel technology highlights the importance of ongoing research. Integration with IoT, advancements in materials, and the application of nanotechnology are shaping the landscape of possibilities ahead. The evolution of these elements assures significant contributions to various sectors, rendering microchannels a pivotal technology in contemporary scientific achievement.
The End
In concluding this exploration of microchannels, it is imperative to recognize the multifaceted nature of this technology. Microchannels are crucial in various domains, significantly influencing both scientific research and practical applications. The unique properties that emerge at the microscale facilitate enhanced fluid dynamics, allowing for improved heat transfer and efficient chemical reactions. Such characteristics lend themselves well to innovative designs in biomedical engineering, chemical processing, and even environmental monitoring.
Summary of Key Points
This article highlighted several essential aspects of microchannels:
- Definition and Overview: Microchannels are small channels that play a vital role in fluid transport and heat exchange.
- Fabrication Techniques: Various methods, including photolithography and soft lithography, are used to create microchannel structures.
- Applications: These systems have significant uses in biomedical contexts, such as point-of-care diagnostics and drug delivery.
- Challenges: Issues like clogging and material limitations present ongoing hurdles.
- Future Directions: Integration with the Internet of Things and advancements in materials open new avenues for research and application.
"The role of microchannels in advancing technology cannot be overstated. They serve as a bridge between fundamental science and practical applications."
Implications for Future Research
The future of microchannel technology is promising, yet challenges persist. Future research should prioritize the following areas:
- Enhanced Materials: Investigating new materials will perhaps lead to more efficient and durable microchannel systems, capable of withstanding harsh chemical environments.
- Integration with Emerging Technologies: Collaborating microchannels with sensor technology, AI, and nanotechnology could optimize performance and open up new applications.
- Sustainability Considerations: Future developments should also address environmental impact, focusing on reducing waste and improving energy efficiency in microchannel operations.
In summary, while significant progress has been made in understanding and utilizing microchannels, a deeper investigation into their potential is essential. This would not only expand their application spectrum but also reinforce their role as a cornerstone in scientific innovation.