Understanding Motion Direct: An In-Depth Analysis
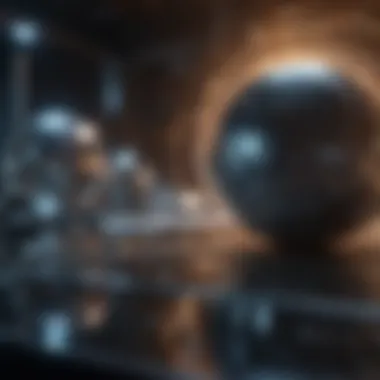
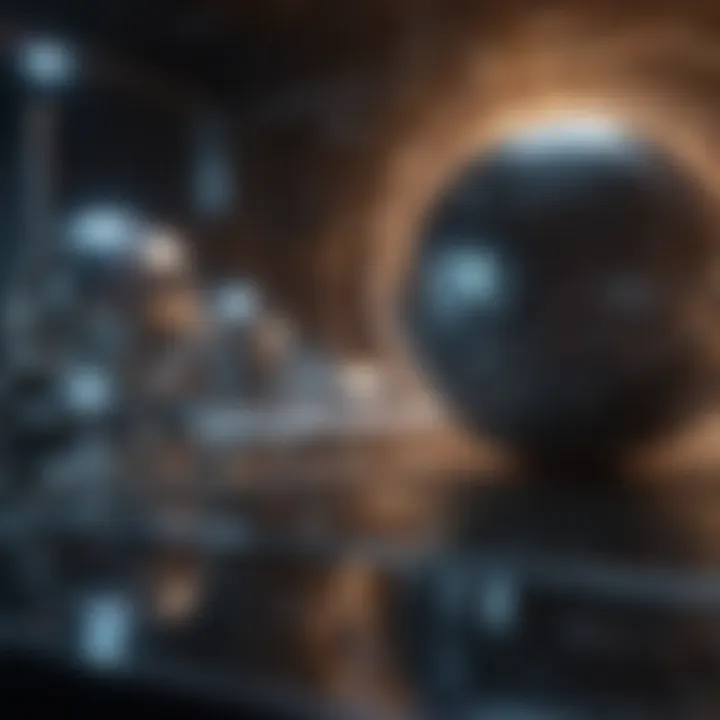
Intro
This article presents a thorough examination of motion direct. The concept plays a pivotal role not only in physics but also in engineering and technology. Understanding the principles of motion directly aids in various scientific domains. In this exploration, we will look closely at the theories that underpin these principles and their real-world implications. Readers can expect to gain valuable knowledge that bridges theoretical understanding with practical application.
Research Overview
In order to comprehend motion direct fully, an overview of research methodologies becomes essential. This section outlines different approaches used in studying motion direct and highlights its significance across multiple fields.
Methodological Approaches
Research in motion direct employs various methods to explore its dynamics. Some common approaches include:
- Experimental Research: Conducting controlled experiments to observe how objects move under different conditions.
- Theoretical Modeling: Developing mathematical models to predict the behavior of moving objects.
- Simulation Techniques: Utilizing computer simulations to visualize motion and test hypotheses.
- Field Studies: Observing motion in natural settings to gather data relevant to real-world scenarios.
These methodologies allow researchers to analyze motion from different angles, enhancing our understanding of its mechanics and applications.
Significance and Implications
The implications of motion direct extend beyond academic interest. It influences various practical fields:
- Physics: Understanding the laws of motion is fundamental to the discipline.
- Engineering: Motion principles directly affect design and testing of machines and structures.
- Technology Development: Innovations in robotics and automation rely on motion understanding.
- Environmental Research: Knowing how motion affects fluid dynamics helps in studying ecosystems.
The significance of motion direct informs a broad range of applications in contemporary science.
Current Trends in Science
The exploration of motion direct reveals current trends shaping scientific research today. Emerging techniques and interdisciplinary connections greatly enhance our comprehension.
Innovative Techniques and Tools
With advancements in technology, new tools have surfaced that facilitate the study of motion direct:
- High-Speed Cameras: Allow detailed observation of fast-moving objects, crucial for analysis.
- Motion Capture Systems: Used in animations and games, these systems help in studying human motion.
- Data Analysis Software: Programs like MATLAB assist in processing motion data efficiently.
These tools enable researchers to gather more precise data and refine their predictions regarding motion.
Interdisciplinary Connections
The study of motion direct does not exist in isolation; it connects with various fields:
- Physics and Mechanics: The foundational principles continue to govern many aspects of motion.
- Computer Science: Algorithms developed for motion analysis provide insights into artificial intelligence.
- Biology: Examining the motion of animals aids in understanding evolutionary biology.
This interdisciplinary collaboration enriches the research landscape and nurtures diverse applications.
"Understanding motion is not just confined to teorical knowledge; it's a skill that bridges various domains, from engineering to ecological modeling."
In summary, motion direct is a significant topic that has implications across various scientific fields. By understanding its principles, researchers can apply concepts effectively in technology and real-world scenarios.
Prologue to Motion Direct
The concept of Motion Direct is significant as it lays the groundwork for understanding how various objects interact within our physical universe. It investigates how movement occurs, under which conditions, and the forces at play, making it relevant in both theoretical and applied science. This section aims to provide clarity around Motion Direct, illustrating its various dimensions and significance.
This introduction establishes a framework that will be explored in subsequent sections. By dissecting Motion Direct, students, researchers, educators, and professionals can begin to appreciate the practical implications it has across numerous fields. The exploration of Motion Direct encompasses the science of how and why objects move, and this understanding is essential in fields like engineering, technology, and physics.
Definition and Context
Motion Direct refers to the unimpeded movement of objects in a straight line, influenced by various physical forces. The notion provides a basis for studying kinematics, which focuses on the geometry of the motion, while dynamics relates to the forces that result in such motion. In essence, it complements a broader understanding of classical mechanics.
Understanding Motion Direct is crucial for numerous applications. For example, it is a key principle in engineering, where the design of structures or machines heavily relies on predictions of motion. The direct relationship between force and motion showcases how energy is transferred and conserved in mechanical systems.
Furthermore, it sets the stage for advanced studies in other realms like quantum mechanics and relativity, where different motions are analyzed under unique conditions.
Historical Background
The exploration of Motion Direct has deep historical roots, primarily established through the work of notable figures like Sir Isaac Newton. In the late 17th century, Newton formulated his three laws of motion, which serve as a foundation for classical mechanics. These laws describe how an object in motion remains in motion unless acted upon by an external force, thereby stressing the importance of understanding inertia and force interaction.
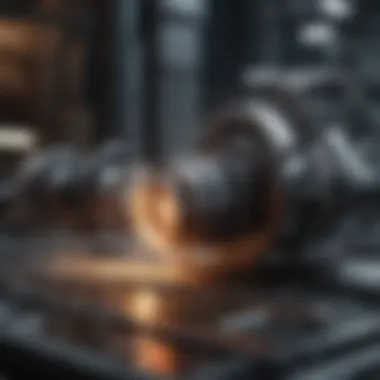
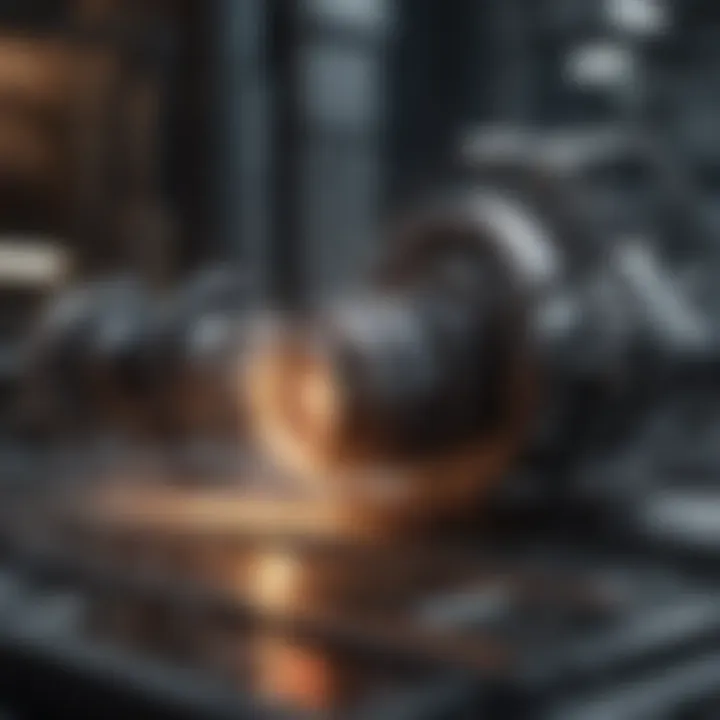
Over the centuries, further advancements have built upon this foundation. The 19th century saw the emergence of key concepts like kinematics and dynamics, offering deeper insights into how motion operates in various systems. Innovative thinkers in physics began to refine mathematical equations to describe motion quantitatively, leading to the establishment of modern physics.
Motion Direct continues to evolve, with contemporary research focusing on developing models that bridge the gap between classical mechanics and newer fields, such as relativistic and quantum physics. Understanding this evolution is vital as it highlights the progress and potential future developments in the study of motion throughout the scientific community.
Theoretical Framework of Motion
Understanding the theoretical framework of motion is crucial for anyone studying the principles of physics and engineering. This framework offers a structured way to interpret the behaviours of objects in motion, enabling analysis and predictions based on established laws. By examining the foundation of motion theory, students and researchers can draw connections between concepts and apply their knowledge in various fields.
One of the primary benefits of studying the theoretical framework of motion is its significant role in problem-solving. When faced with questions regarding physical phenomena, understanding these theories provides a systematic approach to derive solutions. This is notably valuable in disciplines such as engineering, where design and functionality often depend on precise motion calculations.
Moreover, the theoretical framework lays the groundwork for advanced studies in dynamics and kinematics. Without a solid grasp of these concepts, one may struggle to comprehend more complex systems involved in robotics or aerospace engineering. Therefore, it is important to acknowledge this foundational aspect as one builds knowledge in the broader context of motion studies.
Newton’s Laws of Motion
Sir Isaac Newton’s laws of motion are foundational to classical mechanics. They provide a solid basis for understanding how forces interact with objects to influence their motion, forming the cornerstones of the field. Each law addresses different aspects of motion:
- First Law (Inertia): An object at rest stays at rest, and an object in motion stays in motion at a constant velocity unless acted upon by a net external force. This establishes the concept of inertia, describing how objects resist changes in their state of motion.
- Second Law (F=ma): The acceleration of an object is directly proportional to the net force acting on it and inversely proportional to its mass. This equation helps quantify how forces cause motion, linking mass, force, and acceleration.
- Third Law (Action-Reaction): For every action, there is an equal and opposite reaction. This law explains the interactions between objects and why they move in response to each other.
Understanding Newton’s laws is essential for analyzing mechanical systems and predicting how they will behave under various forces. They apply to numerous real-world scenarios, from the simplest object to complex machinery.
Kinematics and Dynamics
Kinematics and dynamics are integral parts of motion studies, complementing each other. Kinematics focuses on describing motion without considering the forces that cause it. It looks into parameters like displacement, velocity, and acceleration. In contrast, dynamics delves into the forces and torques that impact motion, answering the ‘why’ behind the behaviour of objects.
- Kinematics enables the visualization of motion through equations that describe the relationship between time, position, and speed. By applying kinematic equations, one can calculate distances and times without needing to know the forces in play.
- Dynamics, however, integrates the causes of motion into the analysis. It employs the principles outlined in Newton's laws to explain how forces like gravity, friction, and tension affect motion.
Both kinematics and dynamics are vital for a comprehensive understanding of motion, making them necessary components of physics education and engineering applications. For detailed exploration of motion, consider reviewing resources like Wikipedia or Britannica that provide more in-depth discussions.
Types of Motion
In discussing motion, it is crucial to differentiate among its various types. Understanding these differences allows for a more nuanced exploration of motion direct. Each form of motion has unique characteristics and applications that influence behavior in physics and engineering. By focusing on linear, rotational, and oscillatory motion, one gains insights that extend beyond theoretical frameworks. It can uncover practical implications in technology and research.
Linear Motion
Linear motion refers to movement along a straight line. It is one of the simplest types of motion and is foundational for the study of dynamics. In linear motion, an object can have constant speed or accelerate, affecting its velocity. The concepts of distance, displacement, speed, velocity, and acceleration all play an important role.
For instance, when a car travels down a highway, it illustrates linear motion. This motion is often calculated using equations derived from Newton’s laws. Understanding linear motion facilitates forecasting, essential for engineers in designing transportation systems. Moreover, it serves purposes in various scenarios, from everyday movement to complex simulations in computational physics.
Rotational Motion
Rotational motion involves objects that rotate around an axis. Common examples include the spinning of a wheel or the rotation of the Earth. This type of motion introduces parameters such as angular velocity and torque, which are essential for comprehending the dynamics of rotating systems.
Rotational motion must be examined in contexts such as machinery design and celestial mechanics. For professionals in mechanical engineering, grasping concepts of rotational motion can significantly enhance the efficiency of mechanical systems. Additionally, knowledge in this area allows for improvements in product design, ensuring stability and effectiveness in operation.
Oscillatory Motion
Oscillatory motion is characterized by movement back and forth around an equilibrium position. Examples include a swinging pendulum or the vibration of strings in musical instruments. This type of motion can often be described by sinusoidal functions, reflecting the periodic nature of oscillation.
It's vital to understand oscillatory motion for fields such as acoustics, engineering, and even medicine. In engineering, for example, it can influence the design of structures to withstand vibrations during earthquakes. In medicine, oscillatory motion helps in diagnosing certain conditions, such as monitoring heart rhythms. By recognizing its importance, researchers can develop innovative solutions, maximizing the utility of oscillatory systems.
Understanding the various types of motion is not merely an academic exercise but a necessary investigation into how physical laws manifest in practical applications.
Mathematics of Motion
Mathematics acts as the backbone of understanding motion in a scientific context. It serves not only as a language to express theories but also as a tool to predict outcomes and model real-world scenarios. Without mathematics, many principles governing motion would be merely abstract concepts. The elegance of mathematics allows these concepts to be quantified, leading to concrete applications in physics, engineering, and technology.
The intricate relationship between mathematics and motion illustrates the effectiveness of mathematical models in capturing the dynamics of moving objects. These models help in understanding how forces affect motion and how various parameters interact under different conditions. By employing mathematical principles, practitioners can analyze motion with precision and accuracy.
Several specific elements contribute to the importance of mathematics in motion studies:
- Descriptive Abilities: Mathematical equations describe motion succinctly, providing clear relationships among variables.
- Predictive Power: By using mathematical models, it is possible to predict future behavior of moving objects under varying conditions.
- Optimization: Mathematics bears the capacity to enhance efficiency in processes, especially in engineering applications, where minimizing energy consumption is crucial.
The use of mathematics extends across disciplines. In engineering, it can optimize design parameters, while in physics, it helps explore phenomena like gravity and inertia. Recognizing the role of mathematics in motion lays the foundation for further explorations into its application and implications.
Equations of Motion
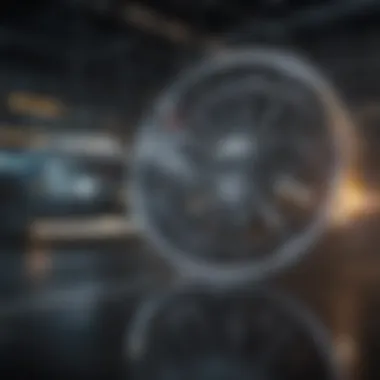
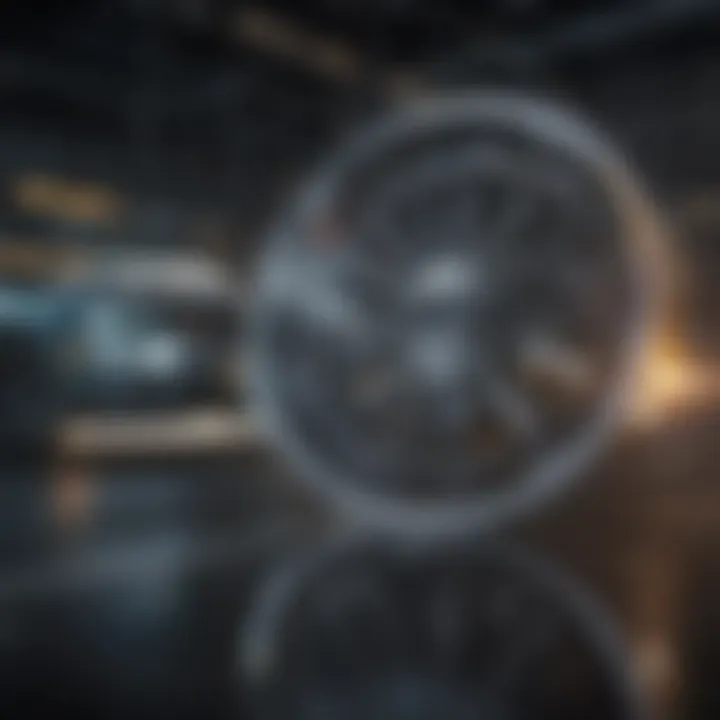
Equations of motion are a fundamental aspect of physics and engineering that describe the relationship between an object’s position, velocity, acceleration, and time. These equations form the basis for analyzing linear motion under uniform acceleration.
The three primary equations of motion are:
- First Equation: [ v = u + at ]
This equation shows how final velocity (v) is derived from initial velocity (u), acceleration (a), and time (t). - Second Equation: [ s = ut + \frac12at^2 ]
Here, displacement (s) is established through initial velocity, time, and the term representing acceleration. - Third Equation: [ v^2 = u^2 + 2as ]
This equation connects velocity with displacement and acceleration, often used when time is not available.
These equations facilitate not just theoretical understanding, but practical applications in various fields. From designing transportation systems to calculating trajectories in sports science, equations of motion are essential.
Vector Analysis
Vector analysis plays a critical role in understanding motion because it allows for a more comprehensive interpretation of multiple dimensions in which an object might be moving. Unlike scalar quantities, vectors carry both magnitude and direction, making them particularly useful in motion studies.
In motion, vectors help in:
- Describing Forces: Forces acting on an object can be represented as vectors. This representation assists in determining net force and acceleration.
- Analyzing Trajectories: Motion often occurs in multiple dimensions. Vector analysis helps dissect the components of motion, such as horizontal and vertical movements, enhancing clarity in complex scenarios.
- Resolving Problems: Vector components can be recombined or manipulated mathematically to resolve motion-related challenges, such as collisions or projectile trajectories.
For instance, the notation for vector quantity can be presented in terms of unit vectors. If ( extbfA ) is a vector, it can be expressed as a sum of its components:
Understanding vector analysis is key for students and professionals in fields ranging from physics and engineering to computer graphics and robotics. It bridges the gap between theory and application, expanding the realm of possibilities in motion studies.
Applications of Motion Direct
Applications of Motion Direct serve as a cornerstone in both theoretical and practical aspects of various scientific disciplines. These applications traverse across engineering, technology, and automation sectors, emphasizing their critical role in innovation and advancement. Understanding how motion principles apply to real-world situations can offer profound insights into optimizing performance, enhancing safety, and pushing the boundaries of what is technologically possible.
Engineering Applications
Engineering fields benefit greatly from the principles of motion directly. From civil engineering, where the stability of structures is paramount, to mechanical engineering involving machinery design, the comprehension of motion is essential. Engineers apply concepts such as velocity, acceleration, and force to design more efficient systems. The ability to model and predict the behavior of materials under motion helps in making informed decisions that ensure safety and efficiency.
Key engineering applications include:
- Structural Analysis: Understanding how forces act on structures helps in developing safe designs.
- Mechanical Design: Motion analysis informs the design of gears, levers, and other components in machines.
- Fluid Mechanics: Analyzing motion in fluids is vital in processes ranging from water supply to aerospace engineering.
Technological Innovations
As technology advances, the necessity for sophisticated applications of motion becomes increasingly apparent. Motion direct principles are central in designing and optimizing various devices and systems. Innovations in materials science, transport technology, and communication systems all hinge on a robust understanding of motion.
For instance:
- Smartphone Devices: Motion sensors in smartphones facilitate features like screen rotation and orientation detection.
- Transportation Systems: The design of new vehicles benefits from simulations of motion to enhance fuel efficiency and safety.
- Wearables: Fitness trackers use motion algorithms to assess activity levels and improve user health insights.
Robotics and Automation
Robotics and automation represent one of the most advanced applications of motion direct. Here, precision and predictability of motion are paramount. Robots are programmed to execute tasks with a high degree of accuracy, and this necessitates an intricate understanding of how motion works in dynamic environments.
In this realm, motion theories guide the development of:
- Automated Manufacturing: Robots operate in factories to enhance production efficiency and accuracy.
- Drone Technology: Motion principles help in optimizing flight paths and stability in various weather conditions.
- Healthcare Robotics: Robots performing surgeries require precise movement to ensure patient safety.
"The integration of motion principles into robotics not only enhances efficiency but also paves the way for pioneering advancements in automation."
Motion in Real-World Scenarios
Understanding motion in real-world scenarios serves an essential function in bridging theoretical concepts with practical applications. Motion direct manifests in everyday occurrences, influencing various fields such as sports science and astronomy. Grasping these concepts can enhance our appreciation of how motion affects both the micro and macro aspects of our environment.
The integration of motion principles can lead to improved efficiency, performance, and innovation. Various elements, such as accuracy, prediction, and optimization, come into play when applying motion theories to real-world scenarios. For instance, analyzing athletes' movements can lead to better training techniques, injury prevention, and game strategies in sports. Likewise, examining celestial motion offers insights into navigation and space exploration, enabling advancements in these domains.
Sports Science
The realm of sports science exemplifies how motion theories directly apply to human performance. Biomechanics, the study of the structure and function of biological systems, focuses greatly on how athletes move. Each action, whether sprinting or jumping, involves complex interactions between muscles, joints, and forces. Understanding these interactions allows trainers and scientists to gather data on optimal techniques and performance metrics.
With wearable technology, athletes can track motion data in real-time. Metrics such as speed, angle of movement, and force can indicate an athlete's performance level. This real-time analysis enables coaches to adjust training regimens accordingly. For example, high-speed cameras can analyze the angle of a sprinter's knee lift, showing precisely how one small adjustment could improve their time significantly.
"In sports, the ability to analyze motion can mean the difference between victory and defeat."
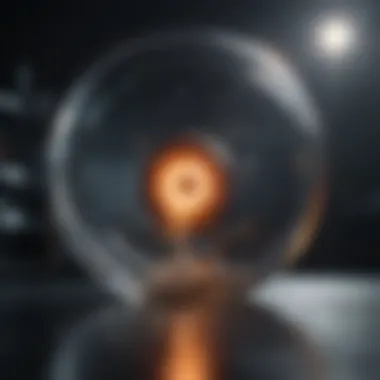
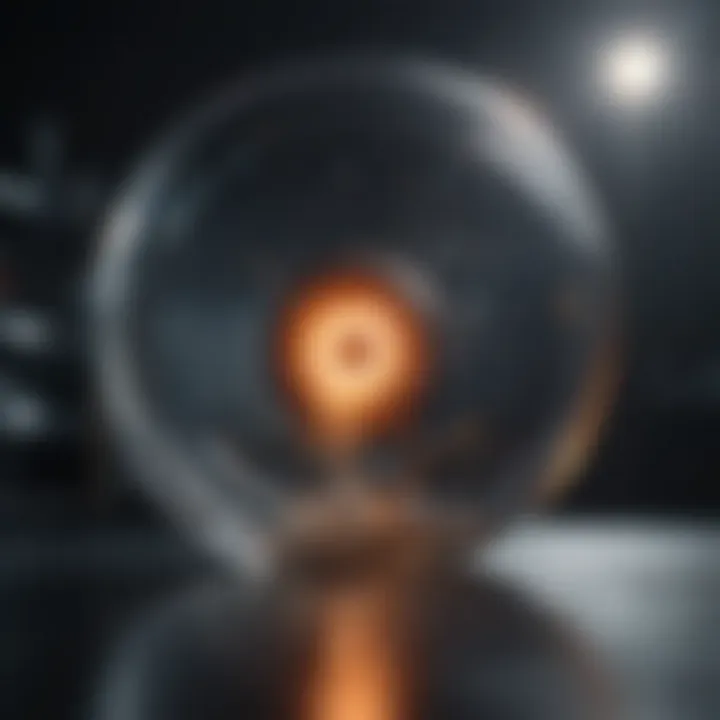
Astronomy and Space Exploration
In astronomy, the principles of motion direct reveal the underlying patterns that govern celestial bodies. The study of how objects move in space is crucial, particularly for planetary motions, satellite trajectories, and spacecraft navigation. For example, Newton's laws facilitate understanding gravitational forces between planets and their orbits.
Furthermore, the study of motion extends into space exploration. Precise calculations determine launch windows and trajectories for spacecraft, ensuring they reach their destinations efficiently. The use of simulation software, based on motion equations, allows scientists to predict and adjust spacecraft paths accurately. This predictive capability has been essential for successful missions, like the Mars rover landings, where exact landing coordinates were critical for mission success.
In summary, motion in real-world scenarios spans diverse fields, from sports to space. Understanding and applying these concepts not only enhances performance and efficiency but also promotes safety and innovation in technology.
Motion Challenges and Limitations
The study of motion is not without its challenges and limitations. Understanding these aspects is crucial as they often dictate the boundaries within which motion can be effectively analyzed and applied. This section focuses on two significant factors: friction and resistance, as well as relativistic effects, which play vital roles in the dynamics of motion.
Friction and Resistance
Friction is a force that opposes the relative motion of two surfaces in contact. It can have both positive and negative implications in motion. On one hand, friction is essential for enabling movement such as walking or driving. Without it, traction would be impossible, and vehicles would slide uncontrollably. However, friction also dissipates energy, leading to a loss in efficiency in mechanical systems. This can result in overheating and increased wear in machinery. Therefore, when analyzing motion, it is important to quantify the frictional forces at play.
Resistance, similar to friction, is a force that counters motion, but it is often discussed in the context of fluids. In fluid dynamics, an object moving through a fluid experiences drag, which is a type of resistance. This is critical in applications such as aerodynamics and hydrodynamics. Engineers must account for both types of resistance to design efficient systems, whether it is in airplanes, boats, or even in sports equipment.
"Understanding the forces of friction and resistance is key to optimizing performance in any system involving motion."
To address these factors, strategies such as lubrication, surface treatments, and streamlined designs can be applied. Evaluating the balance between friction and resistance is essential for improving motion efficiency across various applications.
Relativistic Effects
Relativistic effects arise when an object approaches the speed of light. According to Einstein’s theory of relativity, as an object moves faster, its mass effectively increases. This leads to significant changes in how motion is perceived and modeled at high velocities. For instance, time dilation occurs, meaning time slows down relative to an observer at rest. Such effects are not noticeable in everyday life but become critical in fields like astrophysics and particle physics.
In engineering and technology, understanding relativistic effects can be crucial for systems based on high-speed travel, such as in satellite communications and GPS technology. Failure to incorporate relativistic mechanics can lead to inaccuracies in navigation and timing systems.
The implications of these challenges and limitations are profound. They influence not only the theoretical understanding of motion but also redirect practical applications and innovations in technology and research. Whether in engineering design or advanced scientific research, acknowledging these challenges is an essential part of progressing in the study of motion.
Future Trends in Motion Studies
The study of motion is constantly evolving. As our understanding of the principles governing motion develops, so does the incorporation of new technologies and methodologies. This section explores the future trends in motion studies, emphasizing the importance of such advancements in enhancing research and practical applications across various fields.
Emerging Technologies
Technological advancements play a significant role in shaping future studies of motion. One notable area is the application of big data and artificial intelligence in analyzing motion patterns. With the explosion of data in various domains, researchers can now utilize machine learning algorithms to scrutinize vast amounts of motion data. For instance, in sports science, motion capture technology combined with AI can provide real-time feedback to athletes, helping to optimize performance and prevent injuries.
Another emerging technology is the increase in sensor technology. Wearable devices, equipped with accelerometers and gyroscopes, gather motion data more accurately than before. This precision opens new doors in fields like health monitoring and rehabilitation, where motion analysis is crucial. With better sensors, understanding nuances in human motion leads to improved therapies and personalized health strategies.
Moreover, advancements in simulation tools are also pivotal. Tools like MATLAB and Simulink assist researchers in creating accurate models of motion. These simulations help visualize complex interactions in mechanical systems, which may have previously been difficult to observe in a lab or real-world environment.
"Emerging technologies not only refine existing methodologies but also create new avenues for exploration and innovation in the study of motion."
Interdisciplinary Research
The future of motion studies lies significantly in interdisciplinary research. Fields like biomechanics, robotics, and environmental science increasingly overlap with traditional physics motion studies. This integration fosters a rich environment for collaborative projects. For instance, a biomechanics study may incorporate insights from robotics to develop better prosthetics that mimic natural motion more closely.
Furthermore, the dialogue between physics and psychology is growing stronger. Understanding the psychology of human and animal movements can enhance fields like animation and virtual reality. Researchers in these areas can develop experiences that feel more authentic by studying natural motion and incorporating psychological principles in design.
Interdisciplinary collaboration brings about comprehensive solutions to complex problems. When different fields unite, the study of motion can advance in unexpected and beneficial ways, addressing challenges not only in engineering and technology but also in health and environmental sciences.
In summary, as motion studies progress, the convergence of emerging technologies and interdisciplinary approaches will shape future directions. Keeping an eye on these trends is essential for students, researchers, educators, and professionals seeking to understand and utilize motion in their respective fields.
Culmination: The Importance of Motion Direct
The study of motion direct is central to understanding various scientific phenomena. To grasp motion direct is to engage with the quintessential principles that underlie physical interactions in the universe. This topic combines theoretical frameworks with practical applications, thus illustrating its relevance in real-world scenarios in numerous fields such as physics, engineering, robotics, and beyond.
Summary of Key Findings
This article has highlighted several key aspects of motion direct. First, it elucidates Newton’s laws, important for foundational understanding. Additionally, the types of motion—linear, rotational, and oscillatory—were defined, each showcasing different characteristics and behaviors. The mathematics of motion, including equations and vector analysis, was also addressed to show the quantitative measures of motion.
Furthermore, practical applications in engineering, technology, and environmental sciences demonstrate how these principles come to life. For instance, robotics relies heavily on understanding motion to create efficient and precise movements. Studies of motion provide insights for innovations in automated systems and contribute significantly to fields like space exploration.
Implications for Future Studies
Looking ahead, the relevance of motion direct is only increasing. Emerging technologies such as artificial intelligence and machine learning push the boundaries of how we understand motion. They integrate interdisciplinary research, providing new insights into complex motion behaviors. The importance of this topic extends to various sectors, including healthcare, where understanding human motion can lead to improved rehabilitation methods.
Research into motion direct should continue to evolve, focusing on not only theoretical development but also its integration with technology. Understanding the implications of motion in contexts like climate change or urban planning could lead to notable advancements. Ultimately, continual inquiry into motion direct encourages collaboration and innovation across disciplines, which is vital in solving complex contemporary challenges.
"Motion direct is not just a topic to study; it is a lens through which to view the interactions within our universe, bridging the gap between theory and practical application."
The insights gained from this exploration can have vast implications for future scientific inquiries and advancements, making it essential for students, researchers, and professionals alike.