Understanding the Complexities of Polypeptide Synthesis
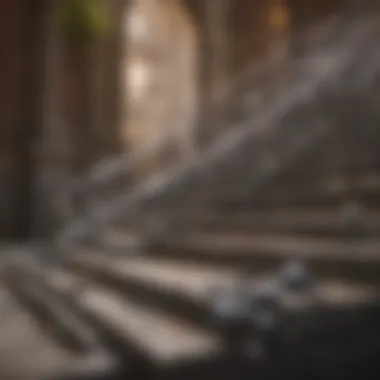
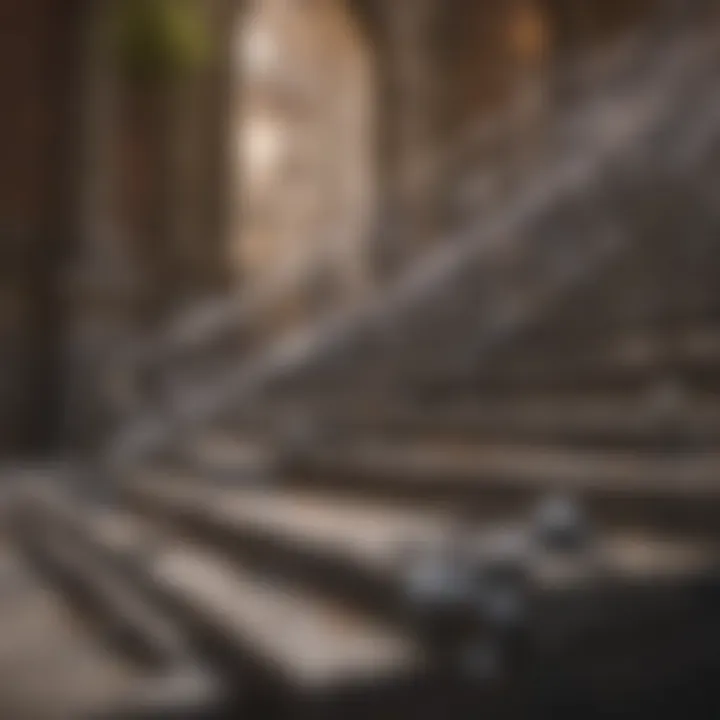
Intro
Polypeptide synthesis is a fundamental biological process that is central to molecular biology and protein biochemistry. The transformation of genetic information into functional proteins involves a series of complex steps, each critical to ensuring proper biological function. Understanding how this process works is essential for students, researchers, and professionals alike as it lays the groundwork for advances in biotechnology and medicine.
This article aims to provide a detailed overview of the polypeptide synthesis process, focusing on crucial mechanisms like transcription and translation. Two key players in this procedure are the ribosomes and transfer RNA (tRNA), which help to translate the genetic code into polypeptides. Furthermore, post-translational modifications also play a vital role in the functionality of proteins.
As we explore the nuances of polypeptide synthesis, we will delve into the methodologies employed in current research, the significance of these processes, and the implications they carry within various fields. Ultimately, this narrative intends to enhance our understanding of how polypeptide synthesis is not merely a laboratory phenomenon but a process that underpins life itself.
Prolusion to Polypeptide Synthesis
Polypeptide synthesis represents a fundamental pillar in the field of molecular biology. This intricate process underlies the translation of genetic information encoded in DNA into functioning proteins, which are vital for numerous biological activities. Understanding polypeptide synthesis is crucial not just for academic exploration but also for practical applications in biotechnology and medicine.
Definition of Polypeptides
Polypeptides are chains of amino acids linked by peptide bonds. They vary in length and complexity, forming the building blocks for proteins. Each polypeptide is synthesized according to the sequence determined by the corresponding gene in the DNA. The precise order of amino acids in a polypeptide dictates its structure and function in biological systems.
Importance of Polypeptides in Biology
Polypeptides play a significant role in many cellular functions. They serve as catalysts in biochemical reactions, known as enzymes, and they are involved in the immune response, cellular signaling, and structural integrity of cells and tissues. Their diverse functions are critical in maintaining homeostasis and responsiveness to environmental changes.
Moreover, abnormalities in polypeptide synthesis can lead to diseases. For instance, a failure in proper protein folding can result in conditions like cystic fibrosis or sickle cell anemia. Understanding the synthesis process is thus essential for developing therapeutics aimed at correcting or compensating for these dysfunctions.
"Polypeptides are not merely structures; they are the instruments of life's orchestration, executing cellular directives with precision."
The comprehension of polypeptide synthesis is also instrumental for advances in synthetic biology. Innovations in this area provide opportunities for designing novel proteins with tailored functionalities for applications such as therapeutic proteins and vaccines.
Overview of Genetic Code
The genetic code is fundamental to understanding polypeptide synthesis. It serves as the blueprint for constructing proteins, which are essential for myriad biological functions. An appreciation of the genetic code allows researchers to grasp how genetic instructions translate into functional entities within cells.
Structure of DNA
DNA, or deoxyribonucleic acid, embodies the genetic information of an organism. Its structure is a double helix, comprising two strands that coil around one another. Each strand consists of nucleotides, which include a phosphate group, a sugar, and a nitrogenous base. The four bases are adenine, thymine, cytosine, and guanine. The sequence of these bases encodes the instructions for synthesizing proteins.
The specificity of base pairing (adenine with thymine, and cytosine with guanine) is vital in maintaining the integrity of genetic information.
The arrangement of these nucleotides creates codons—triplet sequences of bases that correspond to specific amino acids. This triplet nature is critical, as it allows for 64 possible codons to encode for 20 amino acids, underscoring the efficiency and adaptability of genetic coding.
Transcription: From DNA to mRNA
Transcription is the first step in transforming the genetic information embedded in DNA into messenger RNA (mRNA). During this process, the DNA strand unwinds, thanks to the action of the enzyme RNA polymerase. This enzyme synthesizes mRNA by reading the template DNA strand. The resulting mRNA is a complementary copy of the DNA sequence, where uracil replaces thymine.
This mRNA serves as a temporary copy of the genetic instructions, which will later direct the synthesis of polypeptides during translation. The mRNA molecule will then exit the nucleus and enter the cytoplasm, where the ribosomal machinery facilitates the assembly of proteins based on the sequence encoded in the mRNA.
Understanding this mechanism is crucial for grasping how genetic information is utilized by living organisms, establishing a foundation for further exploration of polypeptide synthesis.
Steps in Polypeptide Synthesis
Understanding the steps in polypeptide synthesis is essential for grasping how genetic information is transformed into functional proteins. This process is inherent to all living organisms and informs various biological functions. Each step contributes significantly to the overall efficiency and regulation of protein synthesis, making this topic central to molecular biology.
Initiation of Translation
Role of Ribosomes
The ribosome functions as the site where translation occurs. Its unique ability to coordinate the assembly of amino acids into a polypeptide chain is crucial. Ribosomes consist of ribosomal RNA and proteins, forming two subunits that come together during protein synthesis. Their structural complexity allows them to effectively facilitate interactions between mRNA and transfer RNA (tRNA).
A key characteristic of ribosomes is their ability to recognize the start codon on the mRNA, which signals the beginning of translation. This feature makes ribosomes essential as they set the stage for the entire process of polypeptide synthesis.
One unique aspect of ribosomes is their dual role in binding mRNA and tRNA, crucial for protein knowledge. However, ribosomal function can be affected by certain antibiotics. These antibiotics target ribosomal activity, highlighting both their importance in translation and vulnerabilities that can be exploited in medical treatment.
Binding of mRNA
Binding of mRNA to the ribosome marks the initiation step of translation. mRNA serves as the template that carries the genetic code for the polypeptide. The ribosome's A site is where the mRNA binds. An essential feature of this binding process is the recognition of specific sequences in the mRNA, aiding in the accurate translation of the genetic code.
This aspect of translation is beneficial because it guarantees the fidelity of protein synthesis. Any error in mRNA binding can lead to incorrect protein assembly. A unique feature is the potential for mutations in mRNA to either enhance or disrupt the efficiency of translation. Such alterations can have significant downstream effects on cellular function and organismal health.
Elongation Phase
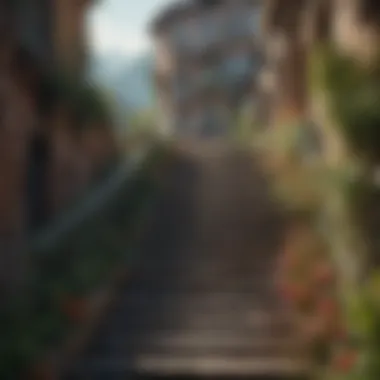
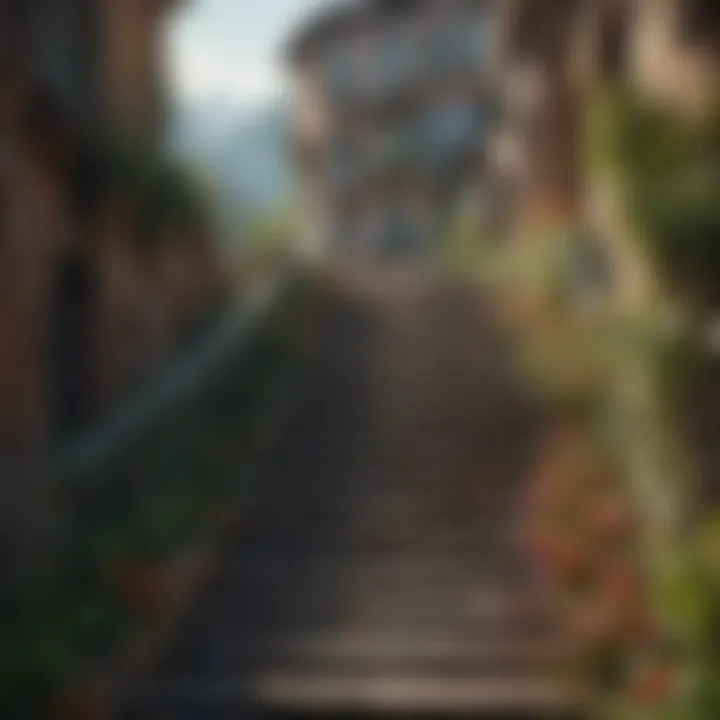
Binding of tRNA
The process of elongation involves the sequential addition of amino acids to the growing polypeptide chain. A crucial aspect of this phase is the binding of tRNA, which carries specific amino acids to the ribosome. Each tRNA molecule has an anticodon that pairs with the corresponding codon on the mRNA, ensuring the correct amino acid is added.
This characteristic of tRNA is a significant benefit for maintaining accuracy in protein synthesis. Each tRNA has a unique structure that facilitates this binding process. One unique feature of tRNA is its ability to recognize multiple codons due to wobble base pairing, broadening the efficiency of translation. However, incorrect tRNA binding leads to faulty proteins, which can impact cellular functions adversely.
Peptide Bond Formation
Peptide bond formation is the representive chemical reaction that links amino acids. During the elongation phase, ribosomes catalyze this formation, creating a new peptide bond between the amino group of one amino acid and the carboxyl group of another. This biochemical characteristic is vital as it defines the sequence and structure of the protein being synthesized.
The significance of peptide bond formation lies in the strong covalent bond it creates between amino acids, which is necessary for maintaining the integrity of proteins. Notably, the ribosome's catalytic activity is an advantageous feature, allowing it to facilitate this reaction efficiently. However, the formation process itself is energy-consuming, requiring input from GTP, which must be regulated carefully to avoid potential cellular energy depletion.
Termination of Translation
Release Factors
The termination phase concludes the translation process. Release factors are proteins that facilitate the recognition of stop codons on the mRNA, signaling the end of polypeptide synthesis. Their role is essential in detaching the completed polypeptide from the ribosome, ensuring a clean conclusion to the process.
One crucial characteristic of release factors is their ability to interact specifically with the ribosomal machinery. This connection is beneficial because it ensures accurate termination, thereby preventing the production of incomplete or dysfunctional proteins. One unique feature is the competition between multiple release factors that can occur, which may affect the efficiency of protein synthesis under different cellular conditions.
Disassembly of the Ribosomal Complex
Disassembly of the ribosomal complex is the final step in the termination phase. After release factors promote peptide release, the ribosomal subunits separate. This disassembly is essential for recycling ribosomal components for new rounds of translation.
The defining feature of this process lies in its role in conserving cellular resources. By disassembling the ribosomal complex, cells can effectively reuse ribosomal components in subsequent protein synthesis events. However, incomplete disassembly can lead to problems such as stalled ribosomes, which can consequently lead to disrupted protein synthesis pathways.
Role of Transfer RNA
Transfer RNA, commonly abbreviated as tRNA, plays a crucial role in the process of polypeptide synthesis. Its primary function involves the accurate translation of the genetic code into proteins. Without tRNA, the synthesis of polypeptides would be highly inefficient and disorganized, failing to produce the precise sequences essential for functional proteins.
Structure of tRNA
tRNA is a relatively small molecule typically ranging from 70 to 90 nucleotides in length. Its structure is distinctively described as a cloverleaf shape, which allows it to fold into an L-shaped three-dimensional structure. This complex shape is essential because it influences how tRNA interacts with ribosomes and mRNA during translation.
The tRNA molecule has several key components:
- Amino Acid Attachment Site: The 3' end of the tRNA binds to a specific amino acid, ensuring that the correct building block is added to the growing polypeptide chain.
- Anticodon: Located at one end of the tRNA, the anticodon consists of three nucleotides that are complementary to the corresponding codon on mRNA. This pairing ensures the specific delivery of amino acids according to the genetic instructions.
The balance between structure and function in tRNA allows it to accurately pair with mRNA and transport the correct amino acids.
Function of tRNA in Translation
tRNA functions as a bridge between the genetic code carried by mRNA and the amino acids that make up proteins. When the ribosome translates the mRNA sequence, tRNA molecules deliver specific amino acids. This delivery process occurs in several steps:
- Binding of tRNA to the Ribosome: Each tRNA molecule enters the ribosome based on the codon sequence present in the mRNA. The anticodon of the tRNA pairs with its complementary codon in mRNA, facilitating correct amino acid placement.
- Peptide Bond Formation: After the correct tRNA binds to the ribosome, it positions the associated amino acid for bond formation with the growing polypeptide chain. This reaction is catalyzed by the ribosome and is crucial for building the protein.
- Recycling of tRNA: Once the amino acid is incorporated into the polypeptide, the empty tRNA is released from the ribosome and can be reused to carry new amino acids for subsequent translations.
The efficiency and specificity of tRNA are essential for maintaining the integrity of protein synthesis, ultimately impacting the functionality of proteins within biological systems.
The precision of tRNA's function is vital for the synthesis of proteins, as even minor errors can lead to malfunctioning proteins with serious biological implications.
Overall, understanding the role of tRNA clarifies a fundamental aspect of molecular biology and its significance in the undeniable process of life.
Ribosomes in Polypeptide Synthesis
Ribosomes are essential cellular machines that play a vital role in polypeptide synthesis. They translate the information encoded in messenger RNA (mRNA) into proteins, which are crucial for numerous biological functions. The proper functioning of ribosomes ensures that proteins are synthesized accurately and efficiently. Disrupting this process can lead to questions regarding cellular health and functionality. Their structure, sites of action, and the steps they perform in translation highlight their importance in biology and biotechnology.
Ribosomal Structure
Ribosomes are composed of ribosomal RNA (rRNA) and proteins, forming two subunits: the large and the small subunit. This intricate assembly is not merely structural; it functions seamlessly in protein synthesis. The small subunit reads the mRNA, while the large subunit catalyzes the formation of peptide bonds between amino acids. The combination of rRNA and protein in ribosomes is optimized for their role in translating genetic information. This unique structure allows ribosomes to adapt and respond to the needs of the cell, being able to control the speed and accuracy of translation.
Ribosomal Sites and their Functions
Ribosomes contain three key sites that are fundamental to the translation process: the A site, the P site, and the E site. Each site plays a distinct role in the synthesis of polypeptides.
A Site
The A site, or aminoacyl site, is where incoming transfer RNA (tRNA) brings specific amino acids. This site is crucial for adding new amino acids to the growing polypeptide chain. A key characteristic of the A site is its high specificity; it recognizes codons on the mRNA and ensures that the correct amino acid is incorporated. This specificity is essential for maintaining the fidelity of protein synthesis. The unique feature of the A site is its ability to accommodate various tRNA molecules, enhancing the efficiency of the ribosomal function. However, if mischarged tRNAs or incorrect codon-anticodon pairing occur, this can impact the overall process of translation negatively.
P Site
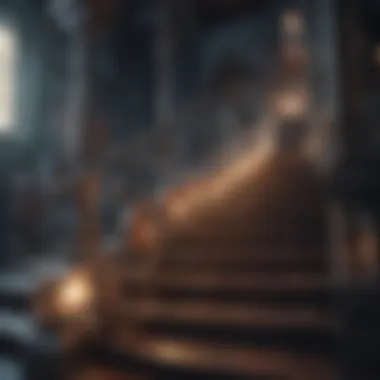
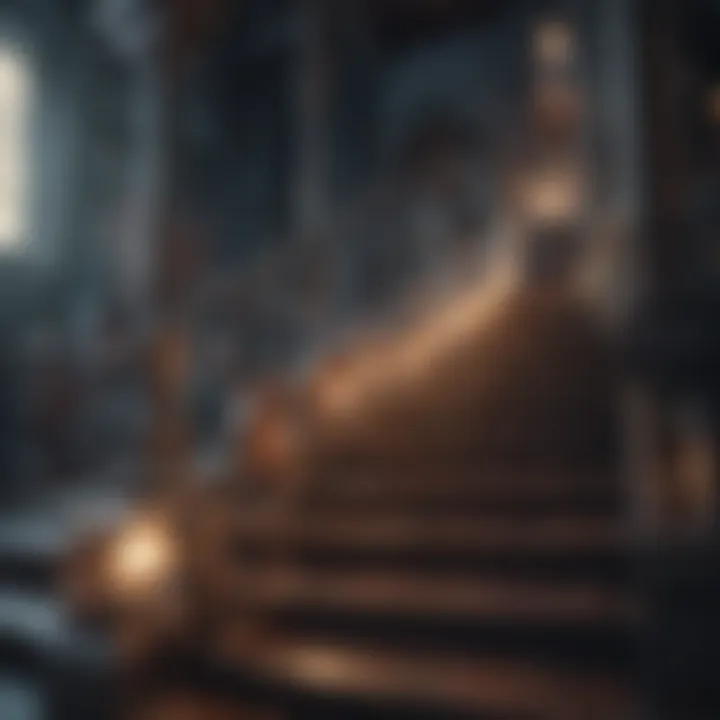
The P site, or peptidyl site, is responsible for holding the growing polypeptide chain. It is where the peptide bond formation occurs between the amino acid in the A site and the polypeptide chain. One key characteristic of the P site is its ability to stabilize the tRNA attached to the growing chain. This feature prevents the premature release of the nascent polypeptide. Nonetheless, if errors occur here, such as incorrect tRNA placement, it can lead to further issues in the synthesis of proteins.
E Site
The E site, or exit site, is where tRNA, after donating its amino acid, exits the ribosome. A characteristic of the E site is its function in recycling tRNA for further use in translation. This allows the ribosome to efficiently utilize resources. The exit site minimizes waste in the protein synthesis process by allowing the old tRNA to leave efficiently. However, if the E site becomes clogged or mismanaged, it can disrupt the overall efficiency of the ribosomal function.
"Ribosomes are the cornerstone of protein synthesis, ensuring that genetic information is transformed into functional biology."
As seen, each site within the ribosome has its own distinct role but operates cohesively to ensure the effectiveness of polypeptide synthesis. By understanding the ribosomal structure and function, we can appreciate how proteins are synthesized with precision and reliability.
Modifications Post-Translation
Post-translational modifications (PTMs) refer to the chemical changes that occur to a polypeptide chain after its initial synthesis. These modifications are critical for the functionality, stability, and localization of proteins. They play a significant role in regulating cellular processes by influencing protein activity, interactions, and degradation. In this section, the significance of PTMs, as well as some common types, will be examined.
Significance of Post-Translational Modifications
PTMs can alter proteins in several important ways. They can enhance protein stability, increase activity, or even target proteins for degradation. The modifications can affect how proteins function within a cell, which is pivotal for maintaining cellular health. Furthermore, many diseases are linked to dysfunctional proteins that may arise from improper modifications. Understanding these modifications is thus essential for researchers and medical professionals.
Common Types of Modifications
There are several notable types of post-translational modifications:
- Phosphorylation
Phosphorylation involves the addition of a phosphate group to specific amino acids, primarily serine, threonine, or tyrosine. This modification serves as an on/off switch for protein activity, influencing signal transduction pathways. The key characteristic of phosphorylation is its reversibility, allowing for dynamic regulation of protein functions. It is popular because of its role in various cellular processes such as metabolism, cell division, and apoptosis. However, improper phosphorylation can lead to diseases like cancer, highlighting its double-edged nature. - Glycosylation
Glycosylation involves the attachment of carbohydrate groups to proteins. This modification affects protein folding, stability, and cell recognition. The key feature of glycosylation is its impact on cell signaling and immune response. It is considered beneficial for enhancing protein solubility and stability. However, excessive or abnormal glycosylation patterns can contribute to pathological states, including certain autoimmune disorders. - Ubiquitination
Ubiquitination is the process of adding small proteins called ubiquitins to a target protein. This modification usually tags proteins for degradation by the proteasome, thus regulating protein levels in cells. The key characteristic of ubiquitination is its specificity in directing protein fate. It is essential for maintaining cellular homeostasis. However, dysregulation in ubiquitination pathways can result in diseases such as neurodegeneration and cancer.
In essence, post-translational modifications are vital to the functionality and regulation of proteins, influencing various biological processes that are crucial for life.
Regulation of Polypeptide Synthesis
Regulating polypeptide synthesis is essential for cellular homeostasis. This process determines when, where, and how much protein is produced in response to various stimuli. The regulation involves both transcriptional and translational mechanisms, allowing cells to respond quickly to environmental changes. This adaptability is crucial for survival, development, and overall cellular function.
One significant aspect of regulation is that it ensures gene expression matches the needs of the organism. Properly regulated polypeptide synthesis prevents excess proteins from accumulating, which can lead to cellular stress or misfolded proteins. Additionally, regulation plays a role in cellular differentiation. Specific cells must produce unique proteins for their function, so controlling synthesis is a key factor in this specialization.
Benefits of Regulation
- Adaptability: Cells can adjust protein levels in response to changes in their environment.
- Efficiency: Regulation ensures that cellular resources are used optimally, preventing waste.
- Specificity: Proteins can be synthesized only when needed, allowing for precise control over cellular activities.
Regulation mechanisms can also be complex, involving feedback loops, signaling pathways, and interaction with other molecules. Understanding these mechanisms provides insight into cellular processes and potential therapeutic targets for diseases where synthesis is disrupted.
"Understanding the regulation of polypeptide synthesis is vital in fields like molecular biology and biotechnology."
Transcriptional Regulation
Transcriptional regulation is the first step in controlling polypeptide synthesis. It occurs at the level of DNA transcription into messenger RNA (mRNA). Various factors govern this process, including transcription factors, enhancers, and repressors. Transcription factors are proteins that bind to specific DNA sequences to enhance or inhibit transcription. By interacting with the promoter regions of genes, these factors influence RNA polymerase's ability to initiate transcription.
Enhancers can be located far from the actual gene but can loop back to interact with transcription machinery. This flexibility allows for a nuanced control of gene expression. Conversely, repressors hinder the transcription process, preventing the synthesis of mRNA when it is not needed. This dynamic balance between activation and repression allows cells to fine-tune their protein output based on internal and external signals.
In addition, epigenetic modifications, such as methylation and acetylation of DNA and histones, can alter the accessibility of chromatin. This modification is also considered transcriptional regulation. By changing the structure of DNA, these modifications can either promote or inhibit the transcription of specific genes.
Mechanisms of Transcriptional Regulation
- Transcription Factors: Bind to DNA to help initiate or inhibit gene expression.
- Enhancers and Silencers: Influence the effectiveness of transcription from a distance.
- Epigenetic Changes: Affect chromatin structure and gene accessibility.
Managing transcription is vital for responding to developmental cues, stress responses, and more. By understanding these elements, researchers can explore therapeutic strategies to modulate gene expression in various diseases.
Translational Regulation
After mRNA is synthesized, translational regulation ensures that it is effectively translated into proteins. This regulation tightly controls the efficiency of translation, influencing how much protein is produced from a given mRNA transcript. Mechanisms of regulation can include the availability of ribosomes, the presence of specific translation initiation factors, and the stability of the mRNA itself.
Translation initiation is a crucial control point. Initiation factors are proteins that assist ribosomes in binding to the mRNA. Different conditions can modulate the activity of these factors, determining whether a ribosome can initiate translation. If conditions are unfavorable, cells may choose to slow down or completely halt the translation process.
Additionally, mRNA stability plays a significant role in translation regulation. Stable mRNAs have a longer life span in the cell, allowing for more translation rounds. Conversely, unstable mRNAs may be rapidly degraded, limiting the amount of protein synthesized. Various pathways, such as nonsense-mediated decay, can target faulty mRNAs for destruction.
Key Factors in Translational Regulation
- Translation Initiation Factors: Proteins that facilitate the assembly of the ribosome on mRNA.
- mRNA Stability: Determines how long an mRNA can be translated before degradation.
- Ribosome Availability: The presence of ribosomes can dictate the rate of translation.
In summary, both transcriptional and translational regulations are integral to polypeptide synthesis. They ensure that proteins are produced in the right quantities and at the appropriate times, allowing organisms to adapt and thrive in their respective environments.
The Role of Ribosomal RNA
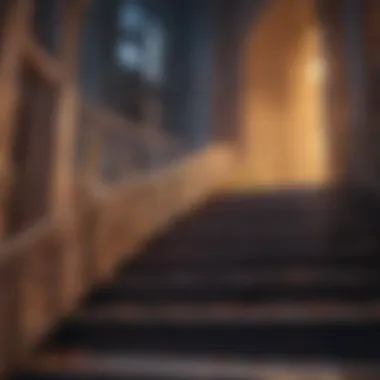
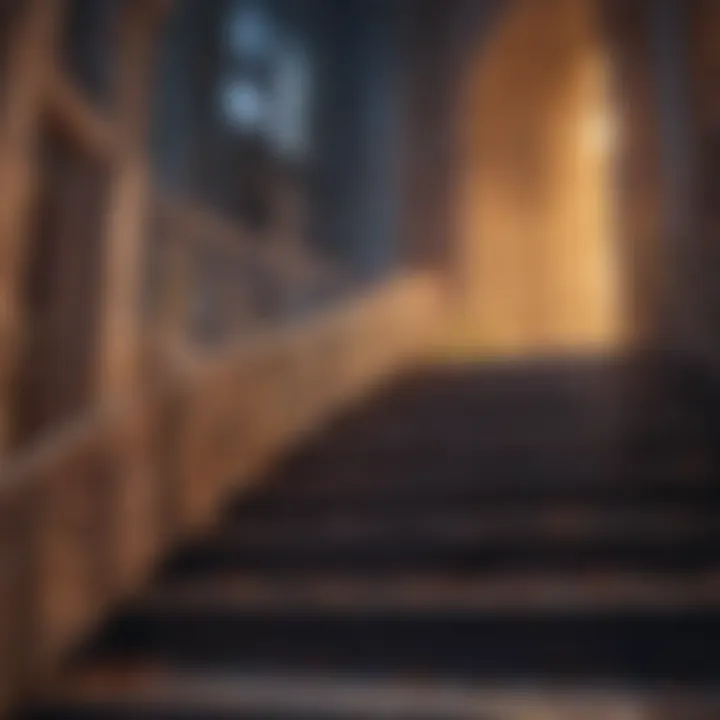
Ribosomal RNA, commonly abbreviated as rRNA, serves a central role in the machinery of protein synthesis. It not only makes up a significant part of ribosomes but also plays crucial functions in the process of translating messenger RNA into polypeptides. The structure and composition of rRNA is specifically designed to facilitate the efficient and accurate assembly of proteins, making it indispensable in molecular biology.
Function of rRNA in Ribosomes
The primary function of ribosomal RNA is to provide a structural framework that helps ribosomal proteins fold into their active conformations. This allows the ribosome to link amino acids together correctly during translation. rRNA molecules are involved in various catalytic activities within the ribosome. For instance, the peptidyl transferase activity, which is pivotal for peptide bond formation, is attributed largely to rRNA.
- Catalytic Role: rRNA acts as a ribozyme, meaning it has catalytic properties. This enzymatic activity is essential for forming peptide bonds between amino acids.
- Structural Role: rRNA provides a scaffold for ribosomal proteins, ensuring proper arrangement and stability of ribosomal structure.
- Decoding mRNA: rRNA is also integral in decoding the mRNA sequence, facilitating the correct pairing of tRNA and mRNA during the elongation phase of translation.
The intricate folding and function of rRNA highlight its essential role in the ribosome, extending far beyond mere structural support.
Formation of the Ribosomal Subunits
Ribosomal subunits are composed of rRNA and proteins. The assembly of these subunits occurs in the nucleolus, a specialized region within the nucleus. Here, the rRNA is synthesized and processed before it is combined with ribosomal proteins imported from the cytoplasm.
- Transcription: Ribosomal RNA genes are transcribed by RNA polymerase I and III. This process yields precursor rRNA molecules that undergo further processing.
- Processing: The precursor rRNA is cleaved and modified to produce mature rRNA. This includes methylation and pseudouridylation, which are critical for rRNA stability and functionality.
- Subunit Assembly: Once processed, the rRNA molecules associate with specific ribosomal proteins to form the small and large ribosomal subunits. The correct assembly is crucial for the ribosome's overall function in protein synthesis.
The precise formation of ribosomal subunits illustrates the complex interplay between rRNA and ribosomal proteins, essential for creating an effective synthesis apparatus in cells. Understanding this process is vital not only for basic biology but also for exploring potential therapeutic interventions that target ribosomal function.
Applications in Biotechnology
Biotechnology relies heavily on the principles of polypeptide synthesis. The ability to produce specific proteins through this process has revolutionized various fields, including medicine, agriculture, and industry. Understanding these applications can provide insight into the practical benefits and considerations that come with manipulating protein synthesis.
One notable application is the production of recombinant proteins. This technique has become a cornerstone in the development of biologics. Recombinant DNA technology allows scientists to insert specific genes into host organisms, enabling them to produce proteins that may not be found in sufficient quantities otherwise. This method is invaluable in generating enzymes, hormones, and antibodies.
Key points about recombinant protein production include:
- Efficiency: Engineered organisms can replicate quickly, increasing the yield of protein production.
- Purity: Recombinant proteins can be purified to high degrees, ensuring their suitability for research and therapeutic use.
- Customizability: Scientists can modify the peptides, adjusting their properties to suit specific needs.
Moreover, the production of therapeutic proteins and vaccines has significant implications for healthcare. Proteins like insulin and monoclonal antibodies are commonly synthesized using these biotechnological methods. These proteins serve critical roles in treating various conditions and diseases, significantly enhancing patient outcomes.
The importance of therapeutic proteins includes:
- Targeted Treatment: They provide specificity in treatment approaches, minimizing side effects.
- Prevention: Vaccines synthesized through biotechnological methods prepare the immune system to fight off diseases effectively, reducing the incidence of infections.
- Research Utility: Many therapeutic proteins facilitate ongoing research in immunology and cell biology, providing better understanding of diseases and their treatments.
"The integration of biotechnology with polypeptide synthesis promises a future where diseases can be treated more effectively, and new therapies can be developed at a faster pace."
Overall, the applications of polypeptide synthesis in biotechnology reveal how essential these processes are for innovation and development in numerous sectors. By harnessing the power of recombinant techniques and therapeutic proteins, biotechnology can address critical challenges faced by society and push the limits of scientific discovery.
Future Directions in Polypeptide Synthesis Research
As scientific inquiry progresses, it becomes crucial to explore the evolving landscape of polypeptide synthesis research. Future directions in this field hold significant implications for enhancing our understanding of biological systems and developing novel applications in medicine and biotechnology. This section emphasizes the relevance of these advancements and their potential to transform our approach to protein synthesis.
Advancements in Synthetic Biology
Synthetic biology is a rapidly advancing domain that combines biology and engineering principles to create new biological systems and functions. One of the primary goals of synthetic biology is to redesign organisms for useful purposes. This involves harnessing the basic mechanisms of polypeptide synthesis.
Recent breakthroughs in gene editing tools, such as CRISPR-Cas9, have revolutionized synthetic biology. Researchers can now modify DNA sequences with precision. This capability enables customized polypeptide synthesis by adjusting the genetic inputs. Furthermore, protocols for designing synthetic genes pave the way for creating proteins with enhanced stability and functionality.
There is also a notable trend towards using machine learning algorithms to enhance protein design. These tools can predict the effects of mutations on protien structure and function, speeding up the development of new enzymes or therapeutic proteins.
Role of Artificial Intelligence in Protein Engineering
Artificial intelligence (AI) is becoming increasingly instrumental in protein engineering. The complexity of proteins often makes traditional experimental methods inefficient. AI algorithms can analyze vast amounts of data, identifying patterns that might not be obvious to human researchers.
These algorithms facilitate the design of novel proteins by predicting their structures and functions based on existing knowledge. For example, DeepMind's AlphaFold has made headlines for accurately predicting protein folding, a critical aspect of understanding polypeptide synthesis. This type of innovation provides a powerful tool for biotechnologists aiming to create proteins with unique properties.
Moreover, AI-driven approaches can streamline the screening process for potential therapeutic proteins, thereby accelerating drug discovery. This can lead to targeted treatments tailored to individual patients, ultimately improving therapeutic outcomes.
"The integration of artificial intelligence in protein engineering marks a new paradigm in the biosciences, pushing the frontiers of what is possible in protein synthesis and application."
Continued investment in both synthetic biology and AI will likely advance the capabilities of polypeptide synthesis extensively. These future directions are not just trends; they represent pathways towards innovative solutions in healthcare, sustainability, and beyond. Understanding these processes will be critical for students, researchers, educators, and professionals who seek to remain at the forefront of molecular biology.
Finale
Understanding the steps of polypeptide synthesis is crucial for comprehending how genetic information translates into functional proteins. This knowledge is fundamental in multiple disciplines, including molecular biology, genetics, and biochemistry. By recapulating the processes of transcription, translation, and post-translational modifications, we can appreciate the complexity and precision with which proteins are synthesized.
Key elements include:
- Initiation, Elongation, Termination: Each phase in translation carries out distinct functions critical to synthesizing proteins accurately.
- Role of tRNA and Ribosomes: tRNA's function in bringing amino acids and ribosomal involvement in facilitating peptide bond formation exemplify the sophisticated machinery of protein synthesis.
- Post-Translational Modifications: These modifications can significantly alter a protein's function and activity, highlighting the importance of processing after translation.
Understanding these steps enhances our grasp of cellular biology, and provides insight into how errors in these processes can lead to diseases. For researchers and educators, such understanding is not just academic; it informs the development of therapeutic strategies and advances in biotechnology.
"The deeper our comprehension of polypeptide synthesis, the more equipped we are to manipulate biological systems for innovation and healing."
In summary, the synthesis of polypeptides is a gateway to uncovering vast potential in both research and clinical environments.