Understanding Restriction Digestion in Molecular Biology
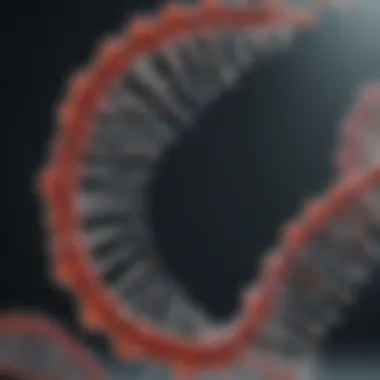
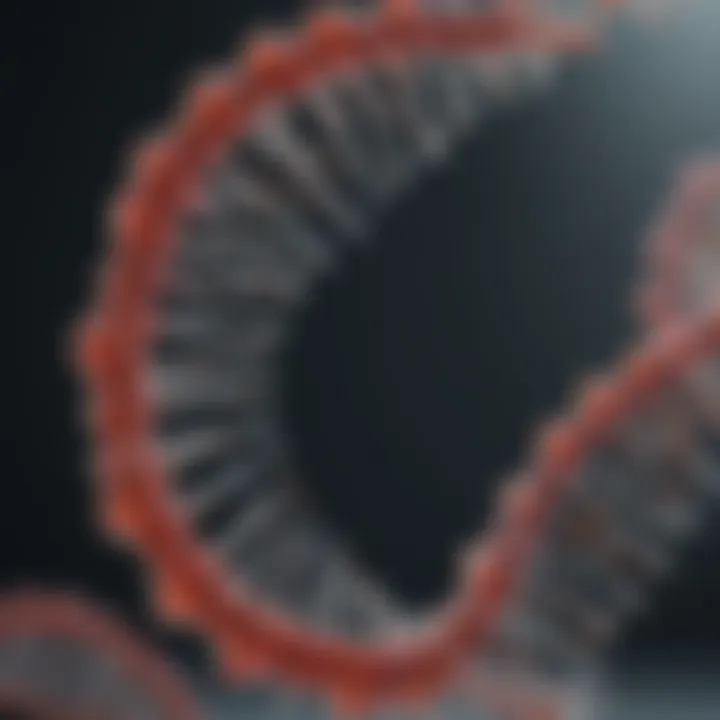
Intro
Restriction digestion is a critical technique in molecular biology, foundational for manipulating DNA in research and applications. It allows scientists to cut DNA at specific sequences, enabling the insertion, deletion, or modification of genetic material. The importance of understanding restriction digestion transcends simple lab protocols; it extends into the realms of cloning, genetic engineering, and advancements in biotechnology. This section will provide an insightful overview of this significant technique, highlighting its mechanisms, applications, and the continuing evolution within the field.
Research Overview
Methodological Approaches
Restriction enzymes, often referred to as restriction endonucleases, are proteins that can recognize specific sequences of nucleotides in DNA. They facilitate the cutting or cleavage of DNA strands at designated sites. The methodology of restriction digestion typically involves selecting the right enzyme based on the target DNA sequence. A common protocol includes:
- Selection of Restriction Enzyme: This can depend on factors like the recognition sequence and the desired sticky or blunt ends.
- Preparation of DNA Sample: The DNA must be isolated and quantified before applying the enzyme.
- Reaction Conditions: Optimal temperature, buffer, and incubation time must be established to ensure effective digestion.
- Analysis of Digestion: Electrophoresis typically follows to visualize the cut DNA fragments.
The careful consideration of these steps is critical for successful manipulation of DNA. Different enzymes exhibit distinct recognition sequences and cleavage patterns, making the choice of enzyme a significant factor in the success of cloning and other genetic engineering tasks.
Significance and Implications
The implications of restriction digestion are far-reaching. This technique not only helps in cloning but also plays a significant role in:
- Gene Mapping: By analyzing restriction fragments, scientists can determine the organization of genes within a genome.
- Genetic Engineering: The ability to precisely cut and join DNA allows for the creation of recombinant DNA, paving the way for novel therapies and advancements in medicine.
- Synthetic Biology: Restriction enzymes significantly contribute to the design and creation of genetically modified organisms (GMOs), which have applications across agriculture and pharmaceuticals.
"Restriction digestion remains a pillar in molecular biology, facilitating significant breakthroughs across various scientific disciplines."
Current Trends in Science
Innovative Techniques and Tools
In recent years, advancements in restriction digestion have emerged, driven by the need for more efficient and precise methods. Innovations like CRISPR-Cas9, while fundamentally different, share the underlying principle of recognizing specific DNA sequences. This intersection signifies a trend where traditional methods of restriction digestion are increasingly being complemented by cutting-edge technologies.
Emerging tools in molecular biology are often integrating multiple methodologies. For instance, next-generation sequencing technologies are being paired with restriction digestion, allowing for rapid and comprehensive genetic analysis.
Interdisciplinary Connections
Understanding restriction digestion is not limited to molecular biology. It finds relevance in bioinformatics, where data analysis includes mapping genomes and predicting the outcomes of specific digests. This capability highlights the importance of interdisciplinary approaches, allowing experts to collaborate and create frameworks for practical applications. The techniques are also crucial in synthetic biology and environmental science, demonstrating far-reaching impacts from ecological applications to developing sustainable agricultural practices.
As scientists continue to explore and expand the boundaries of restriction digestion, the significance and applications of these techniques will only grow, promising a future rich with potential discoveries and innovations.
Prolusion to Restriction Digestion
Restriction digestion is a crucial technique in molecular biology that allows scientists to manipulate DNA with precision. This section aims to provide an overview of its definition, historical context, and significance. Understanding restriction digestion is not just relevant for research labs but also serves as foundational knowledge for students and educators. This topic emphasizes the intersections of genetics, biotechnology, and molecular manipulation.
Restriction enzymes, the proteins that facilitate this process, are essential tools in various applications ranging from genetic engineering to biotechnology. Recognizing their importance can lead to better insights into their utility and limitations in experimental designs.
Definition and Historical Context
Restriction digestion refers to the process of cutting DNA at specific sequences using restriction enzymes. These enzymes serve as molecular scissors that enable researchers to isolate, modify or combine DNA fragments. Historically, the discovery of restriction enzymes dates back to the 1970s when the first enzyme, EcoRI, was isolated from Escherichia coli. This discovery marked a turning point in molecular biology, leading to the development of recombinant DNA technology.
The usage of restriction enzymes paved the way for cloning and analyzing genes. Initially, the enzymes were a curiosity of microbiology, but they quickly became indispensable in genetic research. This shift from basic research to applied molecular biology highlights the versatility and potential of these enzymes.
Importance in Molecular Biology
The significance of restriction digestion in molecular biology cannot be overstated. It forms the backbone of many techniques used in labs today.
Key applications include:
- Cloning: Restriction digestion allows for the cutting and pasting of DNA fragments, crucial for gene cloning tasks.
- Mapping Genomes: This technique assists in genome mapping, which is vital for understanding genetic structures and functions.
- Studying Gene Functions: Researchers can manipulate genes to explore their functions in various organisms.
Moreover, the ability to create recombinant DNA has vast implications for research and industry. It opens doors for the production of therapeutic proteins, genetically modified organisms, and much more.
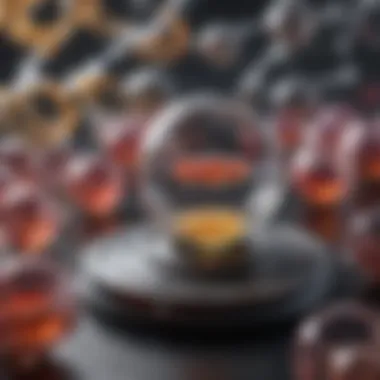
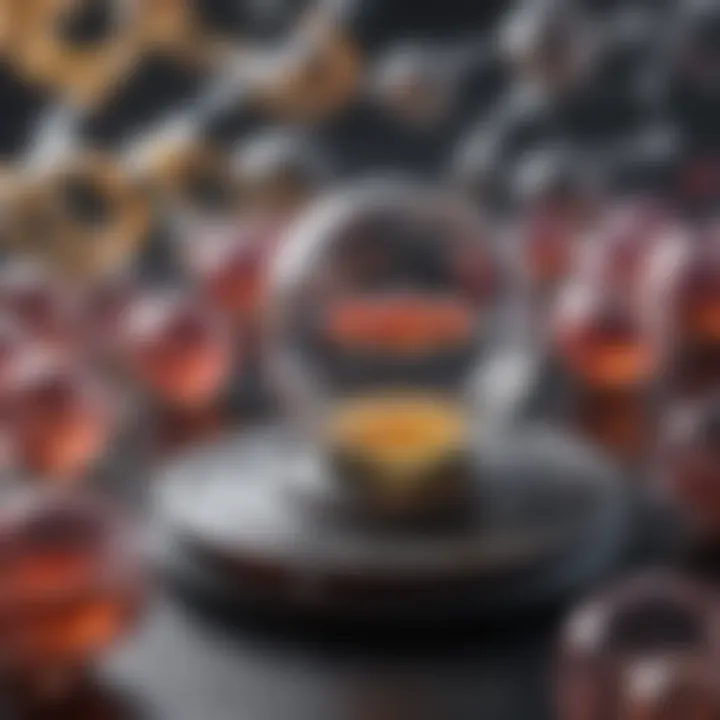
"Restriction enzymes function as sophisticated tools that facilitate the intricate dance of genetic manipulation, serving as the gateway to innovation in molecular biology and biotechnology."
In summary, the introduction to restriction digestion encapsulates its profound impact on biology. The definition and historical context provide a foundation for understanding the mechanisms and applications that follow.
Mechanisms of Restriction Enzymes
The mechanisms of restriction enzymes serve as the foundation of restriction digestion, playing a crucial role in molecular biology. Understanding these mechanisms is essential for researchers and students alike, as it allows for informed decisions when selecting enzymes for various experiments. Restriction enzymes are categorized into different types, each with unique characteristics and functions. These variations dictate how they interact with DNA and the nature of their cuts, influencing the applications and effectiveness of restriction digestion processes.
Types of Restriction Enzymes
Type
Type I restriction enzymes are multifunctional proteins that exhibit both restriction and modification activities. They are less common than other types, but their unique cutting capabilities make them valuable for specific applications. The key characteristic of Type I enzymes is their complex mechanism of action, which requires ATP for function. This aspect may prove advantageous in high-throughput applications where precision is vital. However, their reliance on ATP can also be a disadvantage, as it limits operational conditions. While they are not the first choice for routine cloning, Type I enzymes can be employed in specialized situations requiring complex modifications.
Type
Type II restriction enzymes are the most widely used class in molecular biology. They operate by recognizing specific palindromic sequences in DNA and cutting at defined locations within or near these sites. This predictability is a significant benefit, making them popular choices for cloning and genomic manipulation. A unique feature of Type II enzymes is that they do not require ATP, simplifying the digestion process. Their ability to precisely recognize sites allows researchers to tailor experiments effectively. However, the specificity of Type II enzymes can also limit their flexibility if no suitable recognition site is available.
Type
Type III restriction enzymes exhibit a dual function similar to Type I enzymes. They recognize specific sequences and require ATP for their activity but cut the DNA at a distance from the recognition site. This cutting pattern means that they can generate varied DNA fragments, which may be beneficial in certain contexts, such as creating unique DNA patterns in analytical applications. However, their complexity and the need for specific reaction conditions can pose challenges in standard laboratory protocols, perhaps making them less popular for routine use.
Other Types
Beyond Types I, II, and III, there exist other less common restriction enzymes. These may include Type IV enzymes that are known for their ability to target and edit methylated DNA. Their key characteristic lies in their recognition of modified DNA, which can be a significant advantage in epigenetic studies. However, their use may be constrained due to limited availability and the specific conditions needed for optimal functionality. Other types often provide specialized solutions that fit niche research needs, underpinning the diverse landscape of tools available to molecular biologists.
How Restriction Enzymes Work
Understanding how restriction enzymes work requires a look at their interactions with DNA. They bind to specific sequences in the DNA and induce a conformational change that leads to the cleavage of the double helix. The process can vary slightly between types but generally involves a series of steps: recognition, binding, and cleavage. This specificity and efficiency in processing DNA make them indispensable in molecular cloning and genetic engineering. Furthermore, their reliability in generating consistent fragments is essential for downstream applications such as gel electrophoresis and ligation.
Recognition Sites and Cut Patterns
Recognition sites are particular sequences of nucleotides that restriction enzymes target for cleavage. Most enzymes have a well-defined sequence, typically between 4 to 8 base pairs long, but some can even recognize longer segments. The cut patterns vary significantly depending on the enzyme type. For instance, Type II enzymes often produce blunt ends or sticky ends, which are crucial for subsequent ligation processes. Recognizing the cut patterns associated with each enzyme type assists researchers in planning their experimental setups.
"The precise nature of cut patterns dictates the direction of molecular cloning and genetic engineering."
Overall, knowledge of restriction enzyme mechanisms is fundamental in exploiting their full potential in molecular biology.
Protocols for Restriction Digestion
Protocols for restriction digestion form the backbone of molecular biology research. Understanding these protocols is vital for effective DNA manipulation, which impacts various applications from cloning to genetic engineering. Proper execution of these protocols ensures specific outcomes and reliability in experiments, leading to reproducible results. Below, we outline key components involved in the protocols for restriction digestion, focusing on the preparation of DNA samples, reaction conditions with buffer systems, and incubation times and temperatures.
Preparation of DNA Samples
The first step in the protocol for restriction digestion is the preparation of DNA samples. This stage is crucial because the purity and concentration of DNA can significantly influence the effectiveness of restriction enzymes.
- Isolate DNA: DNA is often extracted from different sources using various methods, such as phenol-chloroform extraction or silica column-based purification. Each method results in DNA of varying purity and yield, impacting the next steps.
- Quantify DNA: Use spectrophotometry or fluorometry to measure the concentration of DNA. This ensures you have the right amount of DNA for the restriction enzyme, typically in the nanogram range.
- Assess Quality: Run an agarose gel electrophoresis to check for DNA integrity. High-quality DNA should be free of contamination and exhibit distinct, high-molecular-weight bands.
By carefully preparing the DNA sample, researchers ensure that they set a solid foundation for the digestion process, minimizing the risk of issues later in the experiment.
Reaction Conditions and Buffer Systems
Having the right reaction conditions is essential for successful restriction digestion. These conditions generally include specific buffer systems that provide optimal environments for enzyme activity, which can vary among different restriction enzymes.
- Buffer Types: Most restriction enzymes function best in their recommended buffers. Typical buffers include Tris-acetate-EDTA (TAE) or Tris-borate-EDTA (TBE) to maintain pH stability and salt concentration.
- pH Levels: The pH of the reaction should typically be around 7.5 to 8.0, which supports enzyme stability and activity. Deviation from this range can lead to reduced enzyme efficiency.
- Salt Concentration: The ionic strength affects the binding of the restriction enzymes to the DNA. Too much salt can hinder enzyme activity, while too little may lead to non-specific activity. It is crucial to adjust salt concentrations accordingly based on specific enzyme recommendations.
- Components: Besides the DNA and buffer, adding other components like BSA (bovine serum albumin) can enhance enzyme performance. BSA stabilizes the enzyme and reduces the chance of non-specific cuts.
Incubation Times and Temperatures
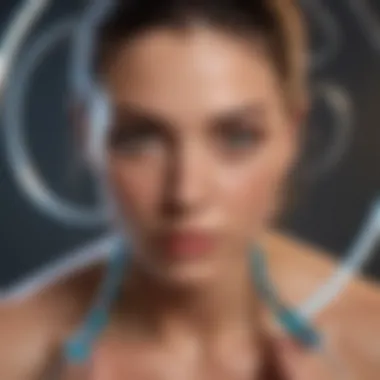
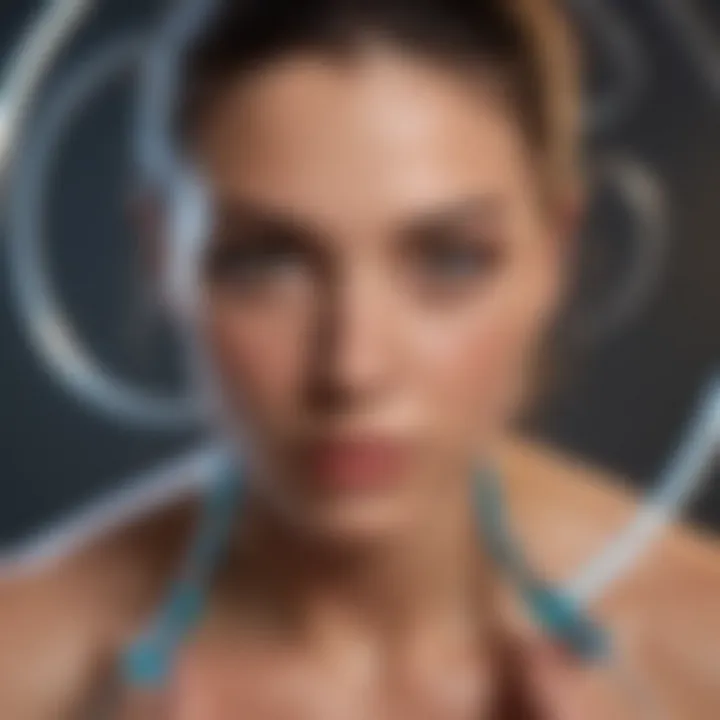
The incubation time and temperature during restriction digestion play critical roles in ensuring optimal enzyme activity. Both factors directly influence how thoroughly the substrate (DNA) will be digested.
- Temperature: Most restriction enzymes work best at 37 °C. However, for some enzymes, lower temperatures might be optimal for preventing non-specific activity, while others might require slightly higher temps for maximal efficiency.
- Incubation Time: Typical incubation times range from 1 to 5 hours. Some protocols may advise overnight incubations for complete digestion, especially with more robust enzymes.
- Monitoring Digestion: It is good practice to check digestion progress. This can be done using gel electrophoresis. Inspecting the results ensures that the DNA is cut into the desired fragments and that no incomplete digestion has occurred.
In summary, protocols for restriction digestion require careful attention to detail in every step. From sample preparation to optimal reaction conditions and precise incubation times, every facet contributes to the overall success of the procedure. Properly executed protocols are beneficial for researchers aiming for high-quality and reliable results in their molecular biology applications.
"Successful restriction digestion results derive from meticulously planned protocols, enabling advanced research across various domains."
For further detailed protocols and information, consider consulting reputable sources such as Wikipedia and Britannica.
Applications of Restriction Digestion
Restriction digestion plays a vital role in many molecular biology applications. Its utility spans various scientific disciplines, making it an essential technique for both basic research and practical applications. The ability to cut DNA at specific sites opens the door to numerous possibilities, including cloning, genetic engineering, and genomic analyses.
Cloning and Genetic Engineering
Cloning remains one of the hallmark applications of restriction digestion. Through this process, researchers can isolate and manipulate specific DNA fragments. This enables the amplification of desired genes, which can then be inserted into vectors for further study or therapeutic uses. By employing restriction enzymes, scientists can create compatible ends on DNA fragments, allowing them to join seamlessly. This method is not just limited to academic laboratories; it also holds significant implications in biotechnology and agriculture, where genetically modified organisms are developed.
Constructing Recombinant DNA
Constructing recombinant DNA involves combining DNA segments from different origins into a single molecule. Restriction digestion is crucial for achieving this goal. By using various restriction enzymes, researchers can tailor the DNA fragments to ensure proper joining. This method leads to the creation of novel genetic constructs that can express proteins absent in the original organisms. The process allows for advancements in therapeutics, vaccine development, and even basic studies of molecular function.
Routine Techniques in Genomics
Furthermore, restriction digestion is a foundational technique in genomics. It simplifies the process of analyzing large genomes by breaking them down into smaller, manageable fragments. Techniques like restriction fragment length polymorphism (RFLP) use this property to differentiate among variations in genes, which is fundamental in genetics research. It also aids in genetic mapping and understanding genetic diversity among populations.
"Restriction enzymes are not just tools; they are the gateway to unraveling the complexities of genetic material."
The applications of restriction digestion are vast and transformative. Each step from cloning to recombinant DNA formation demonstrates its indispensable role in molecular biology. As researchers continue to innovate and explore, the relevance of restriction digestion will likely grow even broader, solidifying its place in scientific advancements.
Challenges and Limitations of Restriction Digestion
Restriction digestion is not without its complications. Understanding the challenges can better equip researchers and practitioners. These challenges could affect the efficacy and outcomes of experiments in molecular biology. Addressing such limitations is significant when planning experiments in genetic engineering. Moreover, it is essential to develop strategies to mitigate these issues and ensure reliable results.
Incomplete Digestion Issues
One of the main challenges encountered in restriction digestion is incomplete digestion. This occurs when restriction enzymes do not cut the DNA at all the intended sites. There are several reasons why this can happen.
- Insufficient enzyme concentration: If the amount of enzyme is too low, it may not interact with all the DNA molecules.
- Short incubation times: If the reaction is not given enough time at the correct temperature, the enzyme may not have enough opportunity to bond and cleave the target DNA.
- DNA quality: The purity of the DNA used can also play a role. Contaminants and structural integrity affect the efficiency of enzyme activity.
Incomplete digestion can lead to several problems, such as the inability to successfully clone a gene.
"Understanding and addressing incomplete digestion is critical for reproducibility in molecular biology experiments."
Star Activity and Non-Specific Cuts
Star activity refers to the occurrence of non-specific cuts by restriction enzymes. Under certain conditions, enzymes can alter their specificity and lead to unexpected cleavages in the DNA sequence. This is often influenced by several factors:
- Altered buffer conditions: If the buffer system differs from the standard requirements, this can induce star activity.
- Higher salt concentrations: Increased salt can lead to changes in enzyme conformation, resulting in non-specific activity.
- Temperature variations: Operating outside ideal temperature ranges can also bigly affect enzyme behavior.
Non-specific cuts can result in the generation of unwanted DNA fragments, complicating the analysis and interpretation of results. This underscores the need for strict adherence to established protocols in digestion reactions.
Influence of DNA Methylation
The presence of DNA methylation adds another layer of complexity to restriction digestion. Methylated DNA can prevent restriction enzymes from recognizing and cutting their target sites. Methylation is a common epigenetic modification that naturally occurs in organisms. Its presence can severely reduce the efficiency of restriction digestion. Various consequences arise from this:
- Reduced cloning efficiency: When potential clones are methylated, they may not be cut properly, leading to a lower yield of recombinant DNA.
- Limitations in certain applications: In epigenetic studies or when working with certain pathogens, methylation status affects how DNA interacts with restriction enzymes.
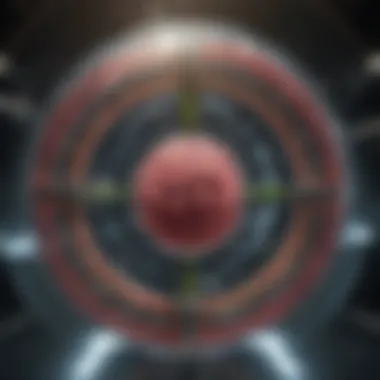
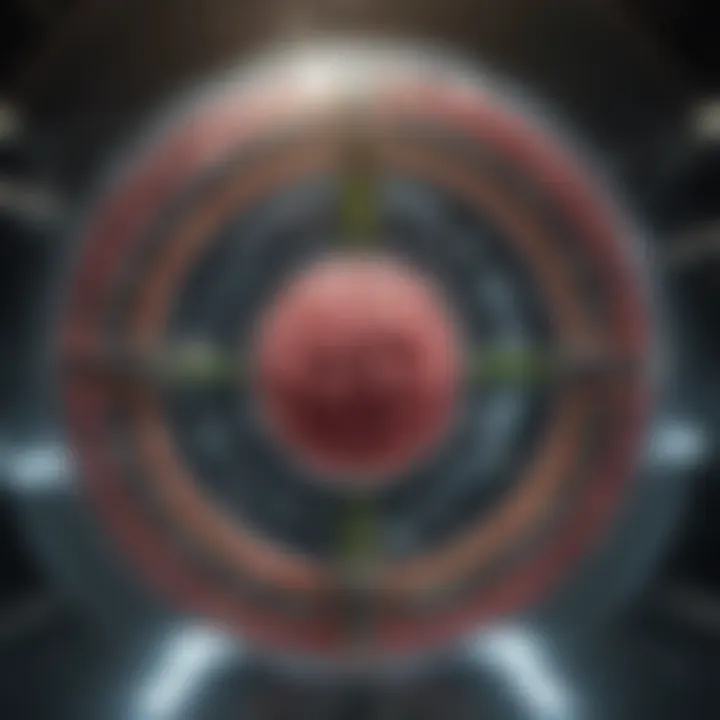
Thus, understanding the implications of DNA methylation is essential for researchers who rely on restriction digestion for accurate results. Managing these challenges involves a combination of correct protocol adherence and an awareness of the molecular context in which restriction digestion occurs.
Advancements and Innovations
Advancements in the field of molecular biology often emerge as a response to the limitations observed in traditional methods. Understanding the recent developments surrounding restriction digestion is crucial for anyone engaged in genetic experimentation and biotechnology. With new technologies, scientists gain more precise tools to manipulate DNA, leading to enhanced efficiencies in research and application.
Next-Generation Sequencing Techniques
Next-generation sequencing (NGS) represents a monumental shift from earlier sequencing strategies. Traditional methods, although reliable, were often time-consuming and costly. NGS, by contrast, allows for the rapid sequencing of entire genomes, which can incorporate results from restriction digestion.
Through NGS, researchers can analyze genetic material at an unprecedented scale. The combination of restriction enzymes with these sequencing techniques enhances the ability to explore complex genetic structures. Here are some notable benefits of these advancements:
- Higher throughput: NGS can process millions of DNA fragments simultaneously.
- Cost-effective: The reduction in costs per base results in broader access to sequencing technology.
- Rich data generation: It generates comprehensive data that can address specific research questions.
The integration of restriction digestion with NGS significantly has increased the understanding of genetic variation and gene function.
CRISPR and Genome Editing
CRISPR technology has transformed our ability to perform genetic modifications. Using a guide RNA, CRISPR directs enzymes to specific DNA locations, enabling targeted cuts. This precision revolutionizes the practice of genetic editing by ensuring that changes can be made in a controlled manner.
The relationship between restriction digestion and CRISPR lies in the precision of the cuts made in the DNA. By pairing these methods, researchers can refine genetic repairs or alterations. Some points to consider include:
- Site-specific targeting: CRISPR allows for accurate modifications, mitigating the risk of off-target effects.
- Versatility: This method's compatibility with various organisms expands the realm of genetic manipulation.
- Efficiency: Using restriction enzymes can streamline the preparation of DNA constructs for CRISPR applications.
Incorporating these tools facilitates advanced genetic studies critical for various applications, from agriculture to therapeutic therapies.
Novel Enzyme Discoveries
The discovery of novel restriction enzymes has opened doors to new possibilities in DNA manipulation. While classical enzymes have been well-characterized, newer variants provide specialized tools for diverse genetic modifications.
These novel enzymes often exhibit unique recognition sequences, allowing greater flexibility in DNA alteration. Their implications include:
- Expanded toolkit: Different cutting patterns enable tailored approaches to genetic engineering.
- Alternative preferences: Some new enzymes work efficiently under varying conditions, improving the scope for experimentation.
- Target specificity: These enzymes reduce unintended off-target effects that could disrupt genetic integrity.
As the field evolves, embracing these novel discoveries equips scientists with the necessary tools to tackle complex genetic challenges effectively.
Epilogue
The conclusion of this article encapsulates the significance of restriction digestion in the broader context of molecular biology. As we have seen throughout, this technique serves as a foundational tool. Its implications reach far beyond mere DNA manipulation.
The Future of Restriction Digestion
Looking to the future, restriction digestion techniques continue to evolve. Here are some specific elements worth considering:
- Integration with Advanced Technologies: As next-generation sequencing techniques improve, the precision and efficacy of restriction digestion will likely enhance. Researchers are constantly seeking methods to optimize results.
- Automation in Protocols: With advancements in laboratory automation, protocols for restriction digestion are becoming more streamlined. This can reduce human error and improve reproducibility.
- Innovative Applications: Restriction enzymes will continue to find new applications in areas such as synthetic biology. The growing field of synthetic biology relies on the precise construction of genetic sequences.
- Emerging Enzyme Technologies: Discoveries of novel enzymes that exhibit unique or improved properties can expand the scope of restriction digestion. Studying these enzymes leads to versatile tools for genetic manipulation.
"Keeping pace with these advancements will be crucial for researchers aiming to remain at the forefront of molecular biology."
In summary, restriction digestion is more than just a laboratory technique; it is an evolving tool that shapes the future of research and technology in genetics. The careful consideration of its applications, limitations, and innovations will be essential for any professional or academic involved in molecular biology.
Importance of References
Here are some critical elements regarding references:
- Credibility: Citing reputable sources adds authority to the discussion. It shows that the information is based on empirical evidence or expert consensus.
- Context: References provide background knowledge and situational context to the main arguments, helping the audience grasp why the subject is important in molecular biology.
- Further Reading: By offering additional material for exploration, references empower readers to expand their knowledge beyond the discussion presented in this article.
- Traceability: Readers can verify claims and findings by consulting original sources, which reinforces the integrity of the discourse.
Considerations When Using References
While using references, several factors should be considered:
- Recency: The field of molecular biology is rapidly evolving. Including recent studies and resources ensures that the information is up-to-date.
- Diversity of Sources: A wide range of references, from peer-reviewed journals to reputable databases, provides diverse perspectives and fosters a more rounded discussion.
- Relevance: It’s crucial to select references that directly support the article's points. Irrelevant citations can dilute the focus and confuse readers.
- Attribution: Properly citing sources avoids plagiarism and respects the intellectual property of other researchers and authors.
Proper referencing is more than just an academic formality. It is essential for maintaining the quality and trustworthiness of scientific literature.
In summary, the references section of this article will not only bolster its credibility but also serve as a gateway for readers to navigate through the complexities of restriction digestion. Well-chosen references illuminate the discussion, making clear the practical implications and future directions for research in molecular biology.