Understanding RT-qPCR Analysis: Techniques and Applications
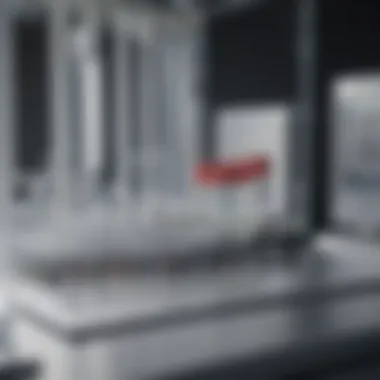
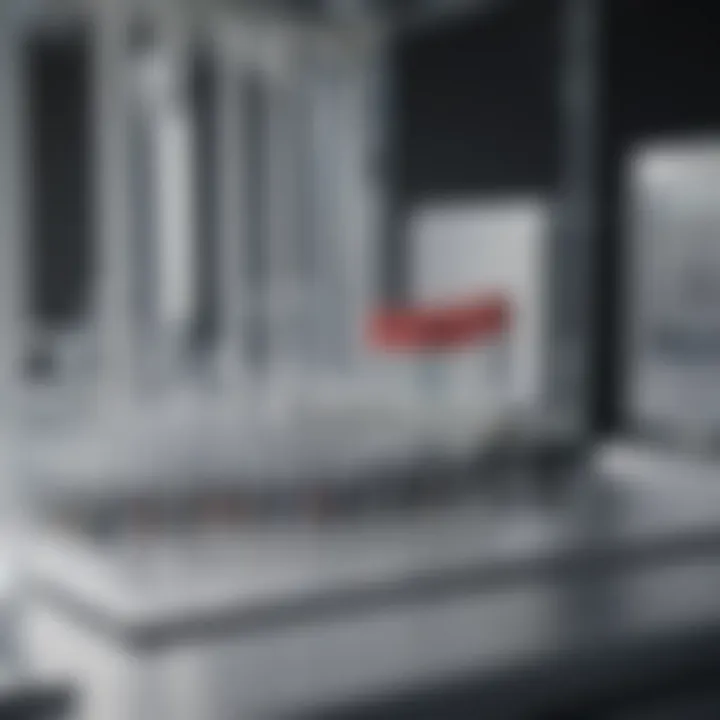
Intro
Real-time quantitative polymerase chain reaction (RT-qPCR) is a cornerstone technology in molecular biology. This technique enables precise quantification of RNA, essential for understanding gene expression and its regulation. As research expands across disciplines, RT-qPCR stands out for its versatility, offering applications in clinical diagnostics, environmental biology, and fundamental research.
The process involves a series of meticulous steps, starting from sample preparation and extending to the interpretation of the data. Each phase holds significance, requiring careful consideration of methodologies and approaches. In this article, we will explore these stages comprehensively, shedding light on their relevance in contemporary science.
Research Overview
Methodological Approaches
RT-qPCR analysis hinges on several methodological approaches. The first step is sample preparation, which involves isolating RNA from cells or tissues. This RNA is then converted into complementary DNA (cDNA) through reverse transcription. The cDNA serves as the template for the quantitative PCR process.
In the PCR phase, specific primers amplify the cDNA along with fluorescent dyes or probes that allow real-time monitoring of the reaction. The quantitation occurs as the fluorescence emitted during amplification correlates with the amount of cDNA present. Thus, RT-qPCR not only measures gene expression levels but also offers insights into the efficiency of the reverse transcription process.
Significance and Implications
The implications of RT-qPCR are profound. It has revolutionized the field of molecular diagnostics, providing a rapid method for detecting viral RNA in infectious diseases like COVID-19. Its sensitivity is unmatched, allowing for the detection of low-abundance transcripts in gene expression studies. Moreover, the ability to quantify RNA levels aids in biomarker discovery, which has transformative potential in personalized medicine.
"RT-qPCR is indispensable for both basic research and clinical applications, bridging the gap between experimental findings and therapeutic interventions."
This technique is also essential in fields such as agriculture, where it aids in the development of genetically modified organisms with enhanced traits. In addition, environmental scientists utilize RT-qPCR to monitor RNA from microorganisms in ecological studies, thus providing insights into biodiversity and ecosystem health.
Current Trends in Science
Innovative Techniques and Tools
The landscape of RT-qPCR is evolving, with innovations enhancing its scope and efficiency. Digital PCR represents a new frontier, allowing absolute quantification of nucleic acids without the need for standard curves. This innovation minimizes variability and increases reliability in results. Furthermore, the integration of microfluidics is making RT-qPCR more accessible, allowing for high-throughput analysis with minimal sample volumes.
Interdisciplinary Connections
RT-qPCR transcends traditional boundaries, connecting various scientific disciplines. The technique is instrumental in genetics, virology, oncology, and environmental science, illustrating its adaptability. Researchers often collaborate, integrating RT-qPCR data with bioinformatics tools to better interpret complex biological phenomena. This interdisciplinary approach fosters comprehensive insights and drives innovation in scientific discovery.
Through understanding RT-qPCR analysis, researchers and practitioners can facilitate advancements in their respective fields, making it crucial for all stakeholders involved in molecular biology.
Prelims to RT-qPCR
Real-Time quantitative Polymerase Chain Reaction, or RT-qPCR, has emerged as a cornerstone technique in molecular biology. This method allows researchers to quantify RNA levels, providing insights into gene expression that are pivotal in various applications. Understanding RT-qPCR is not just about grasping the mechanism; it is about appreciating its profound impact on areas like diagnostics, therapeutic monitoring, and genetic research.
The significance of RT-qPCR lies in its ability to amplify RNA and monitor the reaction in real-time, making it a more precise alternative to traditional PCR methods. The technique offers several key benefits:
- Quantitative Analysis: Unlike conventional methods that only provide qualitative results, RT-qPCR allows for precise measurement of RNA levels.
- Sensitivity and Specificity: RT-qPCR can detect even low quantities of RNA, ensuring high sensitivity, while specific primers enhance the accuracy of the results.
- Rapid Results: The efficiency of real-time monitoring leads to quicker data acquisition, which is crucial in time-sensitive research scenarios.
However, employing RT-qPCR also comes with considerations. Primer design, sample quality, and reaction conditions can significantly affect outcomes. Therefore, a thorough understanding of the methodology and controls is imperative for accurate interpretation of data. This article will explore the intricate aspects of RT-qPCR, guiding readers through its principles, methodologies, and applications, enhancing their comprehension of this powerful tool in modern science.
Overview of RT-qPCR
RT-qPCR integrates reverse transcription and quantitative PCR into a single process, enabling the measurement of RNA molecules. The method begins with reverse transcription, where RNA is converted into complementary DNA (cDNA). This cDNA is then amplified using quantitative PCR, allowing for real-time monitoring of the amplification process. This capability is crucial for assessing gene expression levels in various biological contexts, including development and disease progression.
Historical Development
The development of RT-qPCR dates back to the 1990s when researchers sought efficient ways to quantify RNA. Early models utilized conventional PCR techniques, but the need for quantitative analysis led to the integration of fluorescent dyes.
By the late 1990s, the advent of TaqMan probes and SYBR Green dyes revolutionized RT-qPCR, facilitating real-time detection and quantification. This evolution marked a pivotal point in molecular biology, allowing for more accurate studies of gene expression. The continuous refinement of technology and techniques has since further solidified RT-qPCR's role in numerous fields of research and diagnostics.
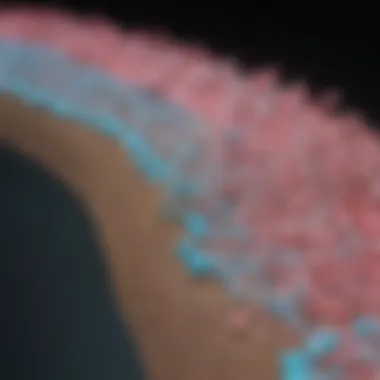
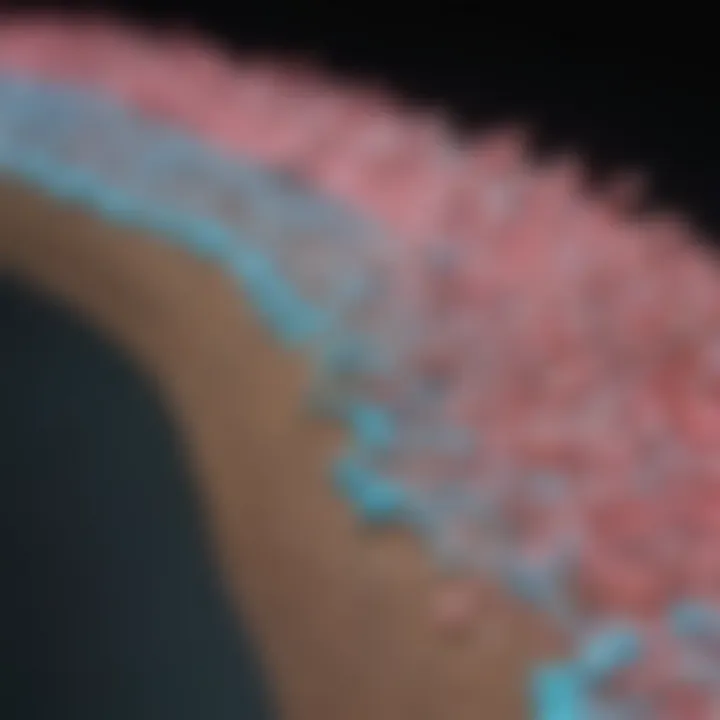
Principles of RT-qPCR
The principles of RT-qPCR are essential for understanding how this technology quantifies RNA. At its core, it is a method to measure gene expression by detecting and amplifying specific RNA sequences in real-time. This section will delve into the molecular foundations and the necessary components that enable RT-qPCR to function effectively.
Molecular Basis of RT-qPCR
RT-qPCR begins with the conversion of RNA into complementary DNA (cDNA) using the enzyme reverse transcriptase. This step is crucial since it allows the analysis of RNA, which holds valuable information about gene expression. cDNA is stable and can be amplified using conventional PCR techniques. The amplification occurs during the PCR cycles where the DNA polymerase enzyme generates multiple copies of the target DNA sequence. Understanding this molecular basis is vital. It illustrates how RT-qPCR is not merely a quantitative technique but also a powerful tool for studying gene activity under various conditions.
Key Components
For RT-qPCR to yield reliable results, certain key components must be utilized effectively. Each plays a distinct yet complementary role in the quantitative analysis of RNA.
Reverse Transcriptase
Reverse transcriptase is a pivotal enzyme that facilitates the conversion of RNA to cDNA. Its primary characteristic is its ability to synthesize a complementary strand of DNA from an RNA template. This enzyme is essential for the entire RT-qPCR process because it enables researchers to analyze RNA molecules.
One of the beneficial aspects of reverse transcriptase is its high fidelity, ensuring that the cDNA produced closely resembles the original RNA sequence. However, various reverse transcriptases exist, and their efficiency can vary. Some might work well with specific types of RNA, while others might present challenges in terms of temperature stability or reaction conditions.
DNA Polymerase
DNA polymerase is another crucial enzyme in the RT-qPCR setup. Its role is to amplify the cDNA, allowing for the detection of strands in real-time. A key characteristic of DNA polymerases used in this process is their ability to withstand high temperatures during amplification cycles, which enhances the accuracy of PCR.
Additionally, there are specific polymerases designed for use in qPCR, which often possess enhanced sensitivity and speed. Despite these advantages, careful optimization is necessary. The choice of polymerase can influence the results significantly, and suboptimal enzyme choice might result in inefficient amplification or inaccurate quantification.
Primers and Probes
Primers and probes are critical for targeting the specific sequences of interest. Primers are short DNA fragments that provide a starting point for DNA synthesis. Their sequence is designed to match the target region of the cDNA. Successful amplification depends on the specificity and efficiency of these primers, making them integral to the accuracy of the analysis.
Probes, on the other hand, are used to generate a measurable signal during amplification. They often contain a fluorescent dye that allows real-time monitoring of the PCR process. The combination of high specificity from primers and efficient signal generation from probes contributes greatly to the reliability of RT-qPCR.
Methodology of RT-qPCR
The methodology of RT-qPCR is a critical component of the entire analysis process, as it addresses how the assay is performed, from the initial sample to the final interpretation of results. It involves several intricate steps that ensure the reliability and accuracy of the data obtained. Each part of the methodology contributes to the overall aim of quantifying RNA molecules, and understanding these steps helps in utilizing RT-qPCR effectively in various applications.
Sample Preparation
Nucleic Acid Extraction
Nucleic acid extraction is vital for isolating RNA from biological samples. The success of RT-qPCR largely depends on the quality and purity of the extracted nucleic acids. Various methods, including phenol-chloroform extraction, silica-based methods, and magnetic bead-based techniques, can be employed. The key characteristic of nucleic acid extraction is its ability to yield high-quality RNA, free from contaminants that could interfere with downstream applications.
One significant advantage of using silica-based techniques is the rapid and efficient isolation of RNA, which is often critical when working with limited sample volumes. However, these methods may also have drawbacks, such as the potential for incomplete extraction or handling difficulties which could lead to sample loss.
Quality Assessment
Quality assessment ensures that the extracted nucleic acids are suitable for further procedures. This process often includes measuring RNA concentration with spectrophotometry and determining integrity through gel electrophoresis. A highly beneficial aspect of quality assessment is its ability to confirm that the RNA is intact and free from degradation.
For instance, using the RNA Integrity Number (RIN) is a common practice to gauge RNA quality. A high RIN value suggests that the RNA is less degraded, which is essential for reliable RT-qPCR results. However, some methods of quality assessment can be time-consuming or require specialized equipment.
Reaction Setup
Optimizing Conditions
Optimizing conditions for RT-qPCR is essential for achieving reliable and reproducible results. This step involves adjusting temperature, enzyme concentrations, and reaction times to ensure efficient amplification of the target RNA. The importance of optimization cannot be overstated, as suboptimal conditions can lead to poor sensitivity and specificity.
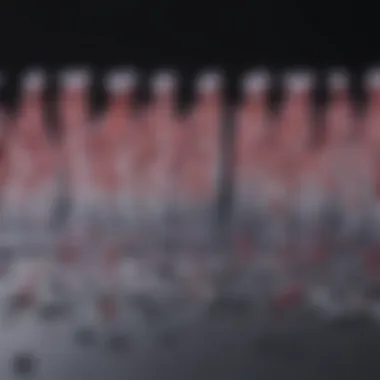
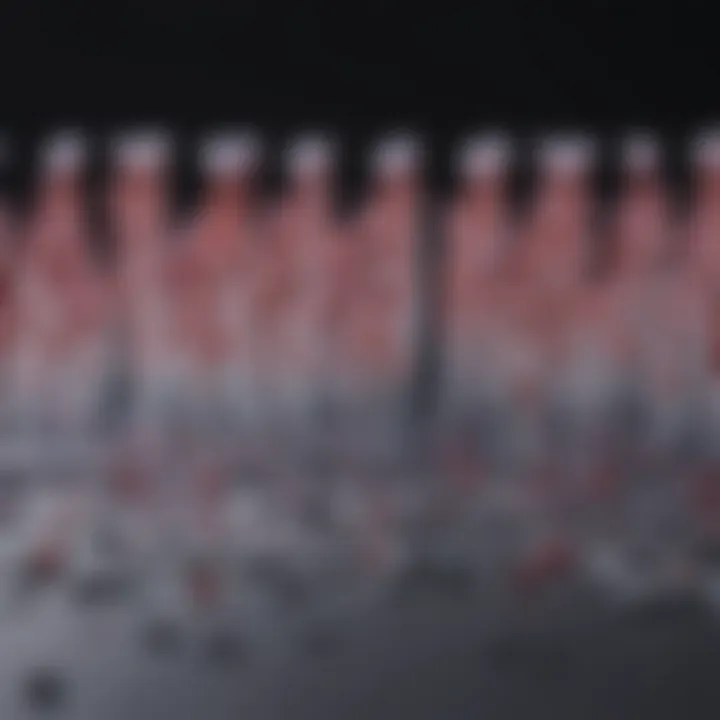
For example, adjusting the annealing temperature based on the melting temperatures of primers can enhance specificity. This aspect is a beneficial characteristic since optimized conditions yield better sensitivity, enabling the detection of low-abundance RNA targets. Conversely, the downside is that optimization can involve trial and error, consuming time and resources to achieve ideal results.
Technical Replicates
The use of technical replicates in RT-qPCR provides a means to ascertain the reliability of obtained data. Running multiple replicates of each sample ensures that any variability in the amplification process is accounted for and corrected. A fundamental feature of using technical replicates is their ability to improve the precision of the results.
For instance, if the differences observed among replicates are minimal, it indicates that the results are consistent. This practice is beneficial, particularly when dealing with experimental variability. However, there is a trade-off to consider: increasing the number of replicates means more reagents and longer processing times, which can be a limitation for high-throughput settings.
Data Acquisition and Analysis
Data acquisition and analysis are pivotal in the RT-qPCR process. This stage transforms the raw data into meaningful insights that reflect the abundance of RNA in a given sample. Effective data acquisition ensures precision in monitoring the amplification of nucleic acids, while proper analysis translates this information into quantifiable metrics. These elements serve as the bedrock for conclusions drawn in research and clinical settings.
Real-Time Monitoring
Real-time monitoring refers to the continuous measurement of the fluorescence emitted during the amplification steps of RT-qPCR. This technique allows researchers to observe the progress of the PCR reaction as it occurs. The key benefit is that it provides dynamic insights into the amplification process, which can lead to enhanced accuracy in quantification.
In practice, real-time monitoring employs fluorescent dyes or probes. These emit signals that correlate directly with the amount of product generated during each cycle. As the reaction progresses, increases in fluorescence signal indicate the formation of new DNA. This real-time feedback loop allows for adjustments to be made promptly in the experimental setup, which can be crucial for obtaining reliable results.
Data Interpretation Techniques
Data interpretation techniques are essential for deriving conclusions from the acquired data. These techniques involve the use of various methods to analyze the quantitative data generated during the RT-qPCR process. Two widely utilized techniques are Standard Curves and Relative Quantification.
Standard Curves
Standard curves are fundamental for quantifying nucleic acid concentrations in RT-qPCR. This technique involves generating a curve from known concentrations of a target nucleic acid, creating a reference for interpreting unknown samples. The key characteristic of standard curves is their ability to provide a quantifiable relationship between the cycle threshold (Ct) values and the initial amount of nucleic acid present in the samples.
Standard curves are a popular choice due to their high degree of accuracy and reproducibility. They facilitate absolute quantification of target RNA by correlating Ct values to the standard curve, enabling precise data interpretation. However, one must consider that the construction of standard curves requires careful calibration and validation to ensure accuracy. Otherwise, variations in the reaction conditions might affect the curve and consequently, the results obtained.
Relative Quantification
Relative quantification is another technique that allows researchers to compare the expression levels of a gene of interest against a reference gene. This method is useful when the goal is to analyze changes in gene expression under different experimental conditions. The key characteristic of relative quantification is that it provides insight into the relative levels of gene expression rather than absolute quantities.
This approach is beneficial because it allows for easier comparisons between samples, particularly when absolute quantification may not be necessary or feasible. However, relative quantification must account for the variability of reference genes, which can influence results if they are not stable across different samples. Despite this challenge, when performed with appropriate controls, relative quantification can yield powerful insights into biological processes.
Understanding both standard curves and relative quantification helps researchers accurately interpret RT-qPCR results, guiding informed scientific conclusions.
Applications in Science and Medicine
The applications of RT-qPCR in science and medicine are vast and impactful. This technique plays a crucial role in various fields, empowering researchers and clinicians to make significant advances. As a method for quantifying RNA, RT-qPCR offers unique insights into biological processes, diagnosis of diseases, and development of new therapies. Its adaptability and precision make it a go-to technique in modern molecular biology.
Gene Expression Studies
Gene expression studies through RT-qPCR facilitate the understanding of how genes function under different conditions. By quantitatively assessing RNA levels, researchers can infer the activity of specific genes. This information is essential in developmental biology, cancer research, and therapeutic drug development. For instance, measuring the expression of oncogenes or tumor suppressor genes can reveal insights into tumorigenesis.
Key points to consider:
- Targeted Analysis: Allows researchers to focus on specific genes of interest.
- Quantitative Data: Provides numerical data that indicates the relative expression levels.
- Comparative Studies: Enables comparison of gene expression across different conditions or treatments.
Analyzing changes in gene expression also offers insights into cellular responses to environmental cues or drug treatments. This capacity to measure shifts in RNA levels can inform treatment strategies and therapeutic decisions.
Pathogen Detection
RT-qPCR has proven invaluable for detecting a wide range of pathogens, including bacteria and viruses. Its high sensitivity and specificity make it suitable for diagnosing infectious diseases. During outbreaks, rapid RT-qPCR assays can quickly determine the presence of specific pathogens, aiding in timely clinical interventions.
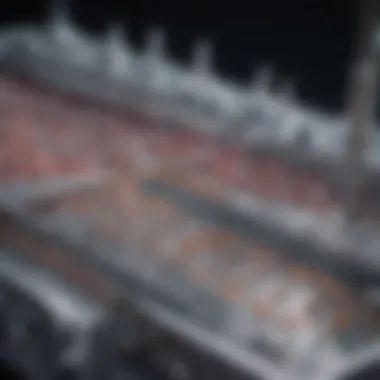
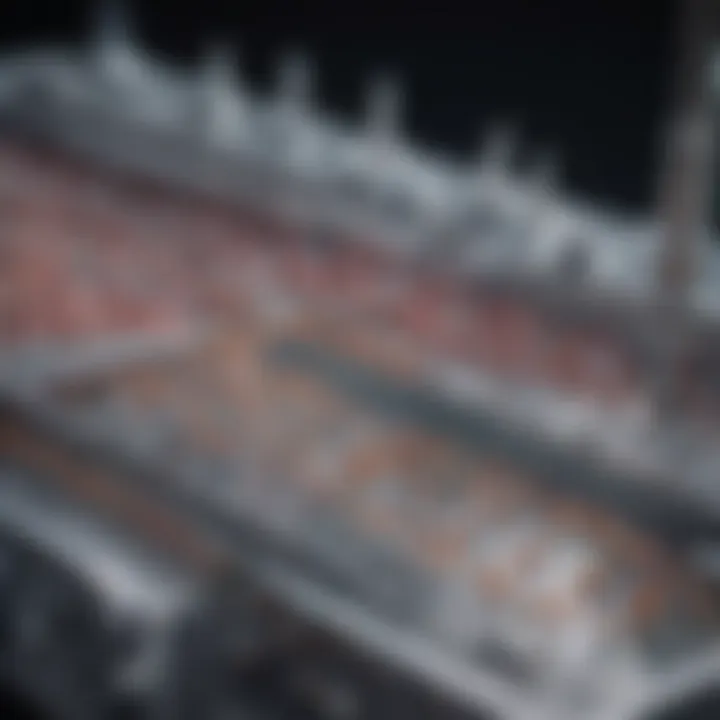
Consider the following advantages:
- Rapid Results: Enables quick detection of pathogens, crucial in clinical settings.
- High Sensitivity: Capable of detecting low levels of nucleic acids, which is vital in early infections.
- Versatile Applications: Can be applied to various pathogens affecting humans, animals, and plants.
An example is the use of RT-qPCR in diagnosing viral infections such as HIV and SARS-CoV-2. By targeting viral RNA, health professionals can confirm diagnoses and implement necessary public health measures.
Clinical Diagnostics
Clinical diagnostics represent one of the most profound applications of RT-qPCR. It allows practitioners to monitor disease progression and response to treatment. For example, RT-qPCR is extensively used in oncology to quantify circulating tumor RNA. This can provide invaluable prognostic information about tumor burden and response to therapy.
Key considerations include:
- Personalized Medicine: Helps tailor treatment approaches based on individual expression patterns.
- Monitoring Disease Progression: Allows for ongoing assessment of treatment effectiveness or disease recurrence.
- Standardization: Advances in RT-qPCR standardization improve comparability of results across laboratories.
In summary, RT-qPCR serves as an essential tool in clinical diagnostics. It bridges laboratory science with patient care, enhancing the quality of healthcare outcomes. The continuous evolution of this technology promises even more refined applications in the future.
Challenges in RT-qPCR
In the realm of molecular biology, RT-qPCR stands as a crucial technique for quantifying RNA expression. However, the journey of achieving reliable and accurate results is often riddled with challenges. These challenges can impact the reproducibility and reliability of the data generated, making it essential to address them thoroughly. Understanding these limitations and variabilities is vital not only for practitioners but also for those who rely on RT-qPCR data for further research and clinical applications.
Technical Limitations
Technical limitations in RT-qPCR can stem from multiple factors that affect the efficiency and fidelity of the process. Some key issues include:
- Instrument Variability: Different qPCR machines have varying levels of sensitivity and specificity. Calibration differences can lead to inconsistent results across experiments.
- Reagent Quality: The performance of reagents, such as reverse transcriptase and DNA polymerase, is critical. Low-quality reagents can lead to erroneous quantifications.
- Sample Quality: The integrity of the RNA sample directly influences results. Degraded or contaminated samples can compromise the accuracy of differential expression analysis.
- Optimization of Protocols: Each assay may require specific conditions for successful amplification. Insufficient optimization can lead to issues like primer-dimer formation, impacting the reliability of quantitative data.
It’s crucial for researchers to be aware of these technical barriers and ensure strict protocol adherence, alongside rigorous equipment maintenance, to mitigate errors associated with RT-qPCR.
Biological Variability
Biological variability is another prominent challenge in RT-qPCR analysis. The intrinsic variations within biological systems manifest in several ways:
- Sample Heterogeneity: Variations in sample composition can lead to differing nucleic acid concentrations and quality across samples, potentially skewing results.
- Expression Level Fluctuations: RNA expression can be influenced by many factors, such as developmental stage, environmental conditions, and methodological approaches. These factors complicate the interpretation of expression data.
- Experimental Reproducibility: Variations between experiments can arise simply from changes in handling techniques or environmental conditions, affecting the reliability of the data.
- Normalization Challenges: The choice of reference genes for normalization is critical. If the reference gene expression is not stable, it can further obscure quantitative accuracy.
Overall, understanding and addressing biological variability is essential for achieving meaningful RT-qPCR results. Both technical and biological challenges must be acknowledged to improve overall experimental design and data interpretation.
Future Directions in RT-qPCR
The field of RT-qPCR is continuously evolving, making it crucial to explore future directions in this area. As scientists strive for greater accuracy, efficiency, and versatility in their experiments, new technologies and methodologies will play a significant role. Future advancements are not just important for improving existing practices but also for shaping how molecular biology is approached in new research and clinical settings.
Emerging Technologies
Advancements in technology are driving the evolution of RT-qPCR, especially in two notable areas: Next-Generation Sequencing and Digital PCR.
Next-Generation Sequencing
Next-Generation Sequencing (NGS) has emerged as a powerful tool for genetic analysis. Its contribution to RT-qPCR lies in its ability to perform high-throughput sequencing. This means researchers can sequence numerous DNA fragments simultaneously, which allows for rapid data collection and analysis. One key characteristic of NGS is its capability to analyze millions of sequences in a single run. This makes it a popular choice among researchers aiming for comprehensive genome or transcriptome analysis.
A unique feature of NGS is its ability to provide more information compared to traditional techniques. However, it can be complex to analyze the resulting data, requiring sophisticated bioinformatics tools. Despite this challenge, NGS's advantages in speed and volume of data make it invaluable in applications like cancer research and infectious disease screening.
Digital PCR
Digital PCR (dPCR) represents another innovative advancement in RT-qPCR methodology. This technique allows for the precise quantification of nucleic acids by partitioning the PCR reaction into thousands of individual reactions. A significant advantage of dPCR over traditional methods is that it does not rely on standard curves, which can introduce variability and errors in quantification.
The unique feature of dPCR is its ability to achieve absolute quantification, providing a clearer interpretation of nucleic acid concentrations. While dPCR is more expensive and technically demanding than conventional RT-qPCR, it holds great promise for applications where precision is critical, like rare allele detection and gene copy number variation analysis.
Potential Innovations
The future of RT-qPCR will also likely see potential innovations that can address current limitations. For instance, integrating artificial intelligence in data analysis could greatly enhance the interpretation of results. Additionally, the combination of CRISPR technology with RT-qPCR could lead to advancements in targeted gene editing.
These innovations can foster greater understanding of complex biological systems and enable researchers to address challenging scientific questions. Thus, the trajectory of RT-qPCR continues to reflect a commitment to both improving existing methodologies and exploring groundbreaking new approaches.