Understanding Sanger Sequencing: A Deep Dive
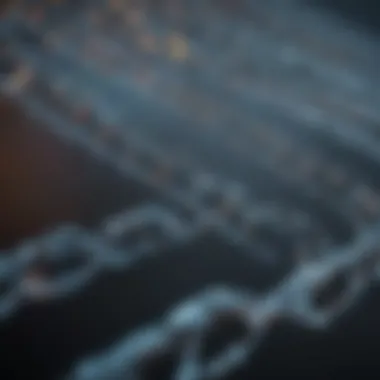
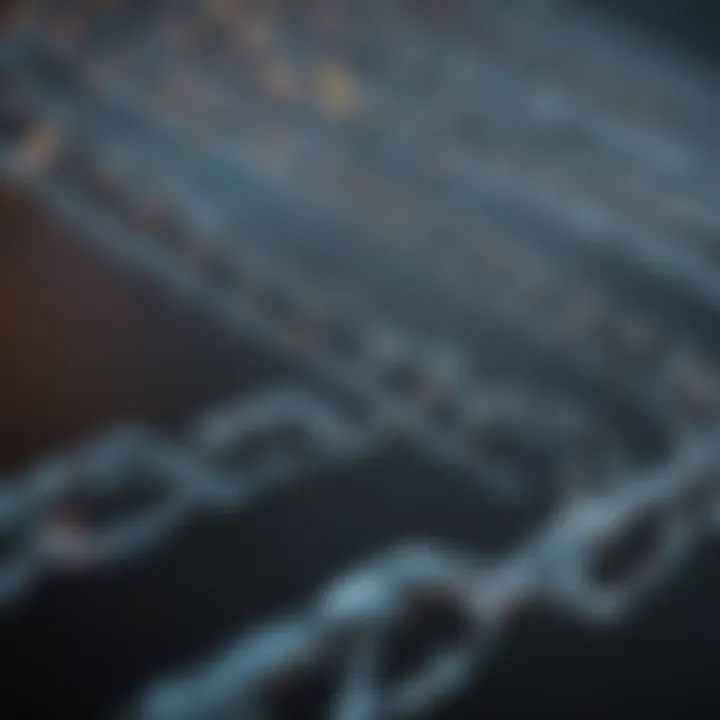
Intro
Sanger sequencing is a cornerstone of molecular biology, a technique fundamental for determining the sequence of nucleotides in DNA. Known for its precision, it has transformed our understanding of genetics since its inception. By delving into the details of this method, we can appreciate its historical significance, biochemical principles, and evolving role in genomics.
In this exploration, we will first provide a research overview that includes methodological approaches, followed by an examination of significance and implications. Subsequently, we will discuss current trends in science, highlighting innovative techniques and their interdisciplinary connections. This article is geared towards students, researchers, educators, and professionals who seek a comprehensive understanding of Sanger sequencing and its impact on genetic research.
Research Overview
Methodological Approaches
Sanger sequencing employs a specific methodology known as the chain-termination method. This process relies on incorporating chain-terminating dideoxynucleotides into a growing DNA strand during replication. When a dideoxynucleotide is added, it prevents the addition of any further nucleotides, thus terminating the chain. The key steps in the Sanger sequencing process typically include:
- Preparation of Template DNA: The DNA to be sequenced is isolated and prepared, ensuring it is free from contaminants.
- DNA Polymerization: A mixture containing the template DNA, DNA primers, DNA polymerase, and dideoxynucleotides is set up. The specific mix allows for the synthesis of varied lengths of DNA fragments, each ending with a dideoxynucleotide.
- Fragment Separation: The resulting DNA fragments are then separated by size, often using capillary electrophoresis or gel electrophoresis. The smallest fragments will travel the furthest.
- Data Analysis: After separation, the fragments are analyzed through fluorescence detection, allowing for the identification of the sequence based on the emitted signals.
All these steps are built on a rigorous scientific basis, and the accuracy of Sanger sequencing has made it a favored method for many applications.
Significance and Implications
The implications of Sanger sequencing are extensive. This technique has significantly contributed to mapping genomes, the identification of genetic disorders, and the study of evolutionary biology. It has been pivotal in major projects like the Human Genome Project, allowing for unparalleled insights into genetic material. Sanger sequencing's reliability and accuracy are essential for fields including:
- Clinical Diagnostics: Accurate sequencing helps in identifying mutations linked to various diseases.
- Forensic Analysis: DNA evidence can be reliably sequenced to help solve criminal cases.
- Pharmaceutical Research: The development of targeted therapies often relies on genetic information derived through sequencing techniques.
"Sanger sequencing remains a unbiased and reliable bold technique even in the age of next-generation sequencing, particularly when accuracy is paramount."
This impact in various fields highlights the importance of Sanger sequencing, both as a standalone technique and as part of a broader toolkit in genomic science.
Intro to Sanger Sequencing
Understanding Sanger sequencing is crucial in molecular biology. This method, developed in the 1970s, is foundational for DNA sequencing. It plays a significant role in various scientific fields, including genomics, genetics, and diagnostics. By exploring the principles and historical context of Sanger sequencing, we can appreciate its impact on modern science.
Definition and Purpose
Sanger sequencing, sometimes called the chain-termination method, is used to determine the precise order of nucleotides in a DNA molecule. The technique allows scientists to read genetic information encoded within DNA. Sanger sequencing has been essential for many applications, such as identifying genetic mutations, cloning genes, and mapping genomes. The purpose of this method extends beyond mere sequencing; it has paved the way for understanding genetic diseases and the development of targeted therapies.
The accuracy and reliability of Sanger sequencing make it a preferred choice for many experiments. It is often employed when high-precision is necessary. Understanding the definition and purpose can enhance the appreciation of where this technique fits within the larger scope of molecular biology.
Historical Context
The historical context of Sanger sequencing offers insight into its evolution and relevance. The technique was first introduced by Frederick Sanger in 1977. Sanger, awarded the Nobel Prize in Chemistry for his work, demonstrated a novel approach to DNA sequencing using modified nucleotides. This groundbreaking research set a new standard for the field.
In the years following its development, Sanger sequencing became the primary method of DNA sequencing until the rise of next-generation sequencing technologies in the early 2000s. Despite its limitations, it remains a widely used method, particularly for smaller-scale sequencing projects and applications where accuracy is paramount.
Sanger sequencing has been instrumental in significant projects, such as the Human Genome Project. Understanding the historical context provides a framework for assessing its impact on genetic research and the continuous quest for knowledge in the life sciences.
"Sanger Sequencing has transformed our understanding of genetics and paved the way for public health advancements."
The Biochemical Basis of Sanger Sequencing
Understanding the biochemical basis of Sanger sequencing is critical for grasping how this technology works and its applications in molecular biology. The method relies on specific nucleotides and enzymes to accurately determine the order of bases in a DNA sequence. By studying its fundamental principles, one can appreciate the elegance and effectiveness of this technique.
DNA Structure and Function
DNA, or deoxyribonucleic acid, consists of two strands forming a double helix structure. Each nucleotide in DNA consists of a phosphate group, a sugar molecule, and a nitrogenous base. The bases are divided into two categories: purines (adenine and guanine) and pyrimidines (cytosine and thymine). The sequence of these bases encodes genetic information, making it crucial for biological processes.
The antiparallel arrangement of the DNA strands allows for base pairing through hydrogen bonding. Adenine pairs with thymine, while guanine pairs with cytosine. The familiarity with DNA's structure is vital, as Sanger sequencing takes advantage of these properties to determine sequences. When DNA is fragmented and modified using the right reagents, the sequencing technique can interpret the genetic code effectively.
The Chain Termination Method
The chain termination method is the hallmark of Sanger sequencing. This process involves incorporating modified nucleotides, called dideoxynucleotides (ddNTPs), during DNA synthesis. Unlike standard nucleotides, which allow further elongation of the DNA strand, ddNTPs lack a 3'-OH group. This absence means that once a ddNTP is integrated, no additional nucleotides can attach to the growing DNA chain, effectively terminating the synthesis at that point.
During sequencing, a mixture of standard nucleotides and ddNTPs is used. When a DNA polymerase enzyme replicates the DNA strand, it will randomly incorporate either a standard nucleotide or a ddNTP. As a result, fragments of different lengths are created, each ending with a ddNTP that signifies the identity of the last base added.
These fragments can then be separated on a gel or capillary electrophoresis system, allowing for visualization and identification of the DNA sequence. The process ensures a high degree of specificity and accuracy, making Sanger sequencing a reliable method for genetic analysis.
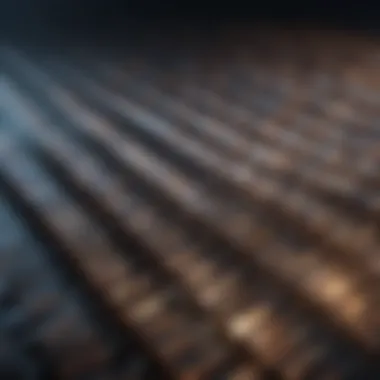
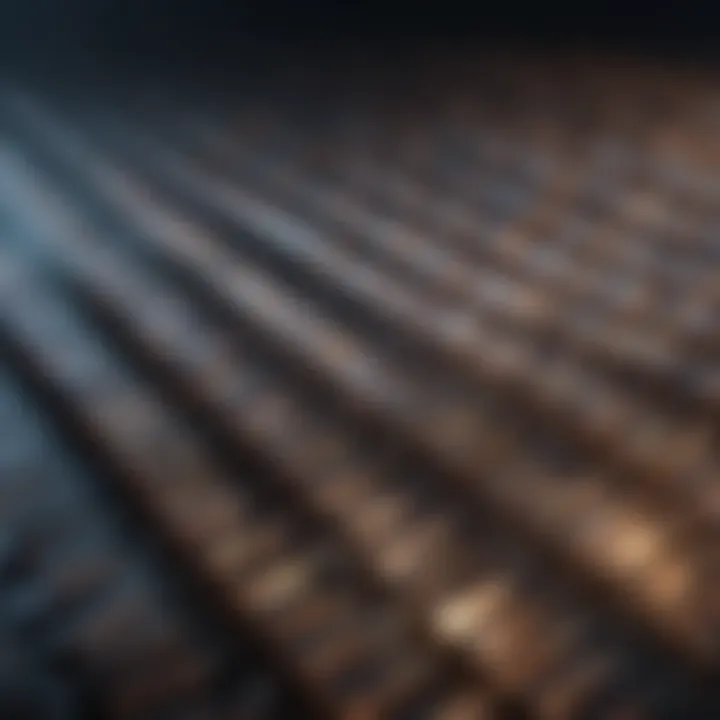
"Sanger sequencing has been a cornerstone in genomics, serving as the standard technique for decades. Its precise methodology continues to offer valuable insights into genetic structure and variations."
The technical nuances of the chain termination method and the structural characteristics of DNA illustrate the robustness of Sanger sequencing. Understanding these elements illuminates the importance of the technique in today’s genomic landscape.
Technical Components of Sanger Sequencing
The technical components of Sanger sequencing are vital to the success of the method. These components work together to ensure accurate determination of DNA sequences. To fully appreciate the nuance of Sanger sequencing, it is essential to understand three main areas: the key reagents, the sequencing protocol, and advancements in automated sequencing techniques.
Key Reagents
In Sanger sequencing, reagents play a fundamental role. Key reagents include:
- Template DNA: This is the DNA strand that is to be sequenced. It serves as the blueprint, guiding the synthesis of new DNA strands.
- DNA Polymerase: This enzyme is crucial for replicating DNA. It adds nucleotides to the growing strand, ensuring complementary base pairing.
- Dideoxynucleotides (ddNTPs): These are modified nucleotides that terminate DNA strand elongation when incorporated. This is how the method derives its name as a chain-termination method.
- Deoxynucleotides (dNTPs): These are the regular building blocks that are incorporated into the DNA strand, alongside ddNTPs.
Each reagent must be of high quality to ensure accuracy. Problems with reagents can lead to sequencing errors. For maximum reliability, quality control measures are necessary.
Sequencing Protocol
The Sanger sequencing protocol is systematic. It involves a series of steps that facilitate DNA synthesis and termination. Here’s a brief overview of the typical Sanger sequencing workflow:
- Preparation of Reaction Mixture: The template DNA, DNA polymerase, dNTPs, and a small amount of ddNTPs are combined in a reaction tube.
- Initiation of DNA Synthesis: The mixture is heated to denature the DNA, separating the strands.
- Extension Phase: Conditions are adjusted to allow DNA polymerase to synthesize the new strand. Incorporation of ddNTPs stops synthesis at random points, creating fragments of various lengths.
- Separation of Fragments: The reaction is processed using gel electrophoresis or capillary electrophoresis to separate fragments based on size.
- Detection: The terminal labeled nucleotides allow visualization of the fragments, enabling sequence determination.
Following this protocol provides reproducible results, making Sanger sequencing a preferred method for many research applications.
Automated Sequencing Techniques
Automation has greatly enhanced the efficiency of Sanger sequencing. Automated platforms streamline the protocol, reducing time and enhancing accuracy. Key advantages of automated techniques include:
- Higher Throughput: Automated sequencers can analyze multiple samples simultaneously. This agility is particularly beneficial for large-scale studies.
- Increased Precision: Automation minimizes human error in sample handling, improving reproducibility of results.
- Improved Data Quality: Advanced detection methods yield clearer data, allowing more precise interpretation of sequences.
The continuous evolution of these technologies reshapes the landscape of genetic research. Automation not only accelerates the sequencing process but also broadens the scope of studies that can be performed with Sanger sequencing.
By understanding the technical components of Sanger sequencing, one can appreciate this enduring technique's relevance in both historical and modern contexts. The synergy between the reagents, protocol, and automation contributes to Sanger sequencing's continued importance.
Applications of Sanger Sequencing
Sanger sequencing plays a critical role in various fields of molecular biology. Its reliability and precision make it a preferred method in numerous applications. Understanding its applications is essential for both researchers and educators in genomics. This section explores cellular genetic testing, research applications, and diagnostic uses of Sanger sequencing.
Genetic Testing
Genetic testing is one of the primary applications of Sanger sequencing. This process is vital for identifying genetic disorders. It allows clinicians to analyze specific genes for mutations that may result in various diseases. For example, analyzing the BRCA1 and BRCA2 genes can help determine an individual’s risk for breast and ovarian cancers.
The accuracy of Sanger sequencing is crucial in genetic testing. Since errors may lead to misdiagnosis, the high fidelity of this method provides a level of assurance in results. Genetic testing via Sanger sequencing is widely accepted in clinical settings and often used in familial disease tracking. Its ability to sequence long DNA fragments provides comprehensive insights into genetic abnormalities.
Research Applications
Sanger sequencing remains a foundational technology in research. It is often used to validate findings from high-throughput sequencing methods. Researchers rely on it to confirm specific sequences of interest in various studies, including those in developmental biology and evolutionary studies.
In addition, traditional biotechnology applications benefit from Sanger's accuracy. It assists in cloning, genotyping, and constructing plasmids. The method enables the detailed sequencing of PCR products and provides reliable data necessary for further biological experiments. Researchers utilize Sanger sequencing for
- Quality control in plasmid preparations.
- Analyzing mutations in cancer studies.
- Studying genetic diversity in population genetics.
Diagnostic Uses
The diagnostic aspects of Sanger sequencing are profound. It is instrumental in identifying pathogens in infectious diseases. Sequencing bacterial genomes can shed light on the resistance genes that they may harbor. It guides treatment decisions in clinical microbiology. By accurately identifying microbes, clinicians can choose the appropriate antibiotics to combat specific infections.
Moreover, in oncology, Sanger sequencing assists in characterizing tumor gene mutations. This information can lead to targeted therapies and personalized cancer treatment plans. A strong understanding of genomic alterations provides healthcare professionals with the capability to tailor treatments based on specific patient needs.
"Sanger sequencing's precise methodology ensures that both researchers and clinicians have reliable data, which is invaluable in both experimental and diagnostic settings."
In summary, Sanger sequencing serves a multifaceted role in the scientific and medical communities. Its importance cannot be overstated, as it supports genetic testing, advances research methodologies, and enhances diagnostic accuracy. This adaptability positions Sanger sequencing as a significant player in advancing our understanding of genetics and disease management.
Advantages of Sanger Sequencing
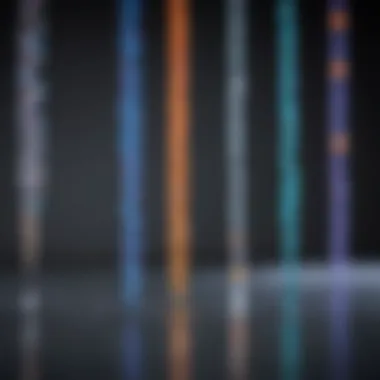
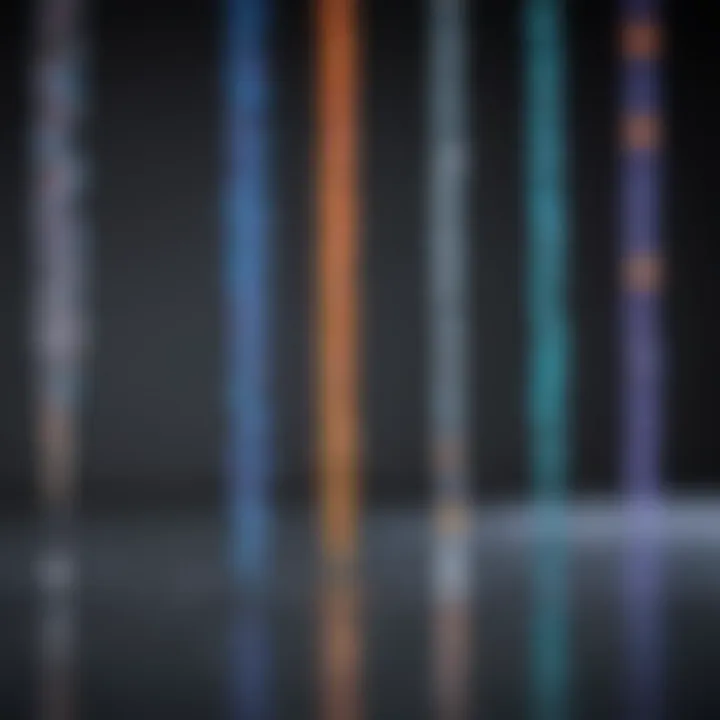
Sanger sequencing offers several benefits that have established it as a preferred method in various applications of molecular biology. One of the primary virtues of this sequencing technique is its accuracy. The process minimizes errors during nucleotide incorporation, resulting in high-fidelity sequences. This accuracy is crucial, particularly in clinical settings, where precise genetic information guides diagnosis and treatment decisions.
Accuracy and Reliability
The accuracy of Sanger sequencing has been well-validated through numerous studies. It operates on the principle of chain termination, which effectively prevents the sequence from extending beyond the designated limits. This feature significantly reduces the likelihood of misidentifications and errors. Typical Sanger reads can achieve up to 99.9% accuracy depending on the quality of DNA used and the sequencing conditions.
In practice, this reliability is vital when researchers are investigating genes associated with disease. For instance, mutations within a particular gene can be identified with high confidence. Consequently, clinicians can develop tailored therapeutic strategies based on precise genetic information, greatly enhancing patient outcomes.
"In precision medicine, the accuracy of genetic information is non-negotiable. Each base pair analyzed contributes directly to patient health."
Simplicity of the Protocol
Beyond accuracy, the simplicity of the Sanger sequencing protocol is another notable advantage. Compared to next-generation sequencing (NGS), Sanger sequencing involves fewer steps and requires less complex equipment. Researchers can execute the necessary protocols without needing extensive technical training or specialized facilities.
This accessibility makes Sanger sequencing a suitable choice for smaller laboratories and research teams. Typical protocols can often be completed within a day, allowing for rapid turnaround times in results. Such efficiency is beneficial for projects with tight timelines or for instances requiring urgent clinical decisions.
In summary, the combination of accuracy and simplicity underscores the ongoing relevance of Sanger sequencing. These advantages not only promote effective research but also enable critical applications in healthcare.
Limitations of Sanger Sequencing
Sanger sequencing is a valuable tool in molecular biology, yet it has notable limitations that need to be acknowledged. Understanding these constraints is essential for researchers and practitioners who wish to select the most suitable method for their needs. The limitations of Sanger sequencing influence its applications, cost-effectiveness, and overall utility in modern genomics.
Throughput Constraints
One significant constraint of Sanger sequencing is its throughput. The technique can process only a limited number of DNA fragments at a time. Typically, Sanger sequencing can handle sequences from a single DNA molecule or a few fragments in one reaction. This limitation results in slower processing times.
Compared to next-generation sequencing (NGS) technologies, Sanger sequencing lacks the capacity for high-throughput analysis. NGS can sequence millions of fragments simultaneously, offering a far higher efficiency for large-scale genomic studies.
- In contrast, Sanger sequencing may take days or weeks to generate data when analyzing the same amount of DNA that NGS could accomplish in a matter of hours.
- This makes Sanger sequencing less suitable for whole-genome sequencing, where vast amounts of data are required.
Thus, when projects demand extensive sequencing efforts, relying solely on Sanger sequencing may not be practical. Researchers must weigh the limitations of throughput against their specific needs before making decisions.
Cost Considerations
Cost is another crucial aspect when discussing the limitations of Sanger sequencing. While the price per reaction may seem reasonable, especially for small-scale projects, the expenses can escalate rapidly with increased sample sizes.
- The individual costs for reagents, equipment maintenance, and labor can accumulate.
- For large-scale genomic studies, Sanger sequencing becomes more expensive than alternative methods like NGS.
Some factors contributing to the overall cost include:
- Reagent Prices: Each Sanger sequencing reaction requires specific reagents that can be costly, particularly when processing large numbers of samples.
- Time and Labor: The hands-on nature of Sanger sequencing necessitates additional time and personnel, increasing project budgets.
Ultimately, as sequencing requires scale, many labs find themselves shifting towards NGS due to its superior cost-effectiveness for comprehensive genomic analysis.
"Sanger sequencing remains the gold standard for certain applications, but its limitations in throughput and cost make it less applicable for high-volume projects."
Comparison with Next-Generation Sequencing
In the landscape of genomic sequencing, Sanger sequencing and next-generation sequencing (NGS) serve pivotal roles, but they are not interchangeable. Understanding their differences is crucial for researchers and professionals aiming for precision in genetic studies. Sanger sequencing, known for its reliability and accuracy, finds itself compared against NGS, which boasts higher throughput and scalability. This section details the technological distinctions and practical applications of each method, facilitating informed choices in sequencing strategies.
Technological Differences
Sanger sequencing relies on chain termination, which involves incorporating dideoxynucleotides into DNA strands during replication. This results in fragments of varying lengths, ultimately allowing the determination of the DNA sequence. In contrast, NGS utilizes massively parallel sequencing technologies. This enables the simultaneous sequencing of millions of fragments, generating vast amounts of data within a shorter timeframe.
Key distinctions include:
- Speed: NGS significantly outpaces Sanger sequencing in terms of speed. What takes days with Sanger can often be accomplished in hours or even minutes with NGS.
- Data Output: Sanger sequencing generates data for one sequence at a time, while NGS can sequence entire genomes in a single run. This makes NGS particularly suitable for large-scale genomic studies.
- Error Rates: Sanger sequencing is often considered to have lower error rates than NGS, making it preferred for applications requiring high fidelity, such as validating variants.
These technological differences highlight both methods' strengths and weaknesses, guiding users in selecting the appropriate approach for specific projects.
When to Use Each Method
Making the choice between Sanger sequencing and next-generation sequencing hinges on the context of the research question and the desired output.
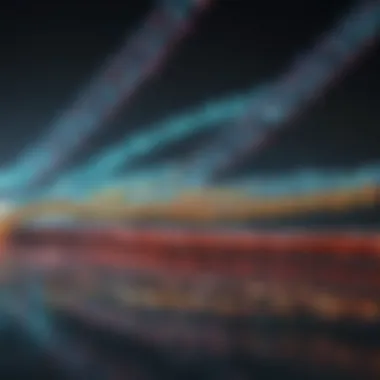
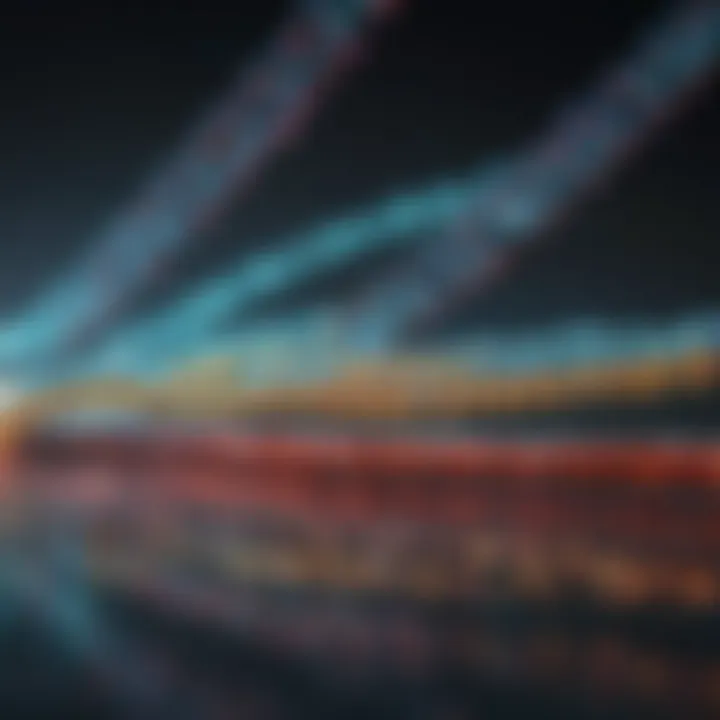
Sanger Sequencing is often ideal for:
- Validation of NGS Results: It is common practice to confirm variants identified through NGS with Sanger methods due to its accuracy.
- Small-Scale Projects: When sequencing a limited number of samples or a specific target region, Sanger provides a cost-effective solution.
- Targeted Sequencing: For projects focusing on a select area of a genome, Sanger's straightforward protocol remains useful.
Next-Generation Sequencing, on the other hand, is best suited for:
- Whole Genome Sequencing: NGS shines in comprehensive studies involving entire genomes, particularly in population genetics and evolutionary biology.
- Large Sample Sizes: Projects requiring sequencing of multiple samples can benefit from NGS's high throughput, saving both time and resources.
- Complexity of Analysis: NGS is indispensable in analyzing intricate genetic landscapes, such as those seen in cancer genomics.
Sanger Sequencing in Current Research
Sanger sequencing continues to hold significant importance in the field of molecular biology and genetics. While newer techniques like Next-Generation Sequencing (NGS) have emerged, Sanger sequencing still plays a crucial role, particularly in validating findings obtained from high-throughput sequencing methods. It offers a methodologically sound approach for confirming variants of interest, allowing researchers to obtain precise nucleotide sequences that contribute to our understanding of various genetic conditions.
Role in Genomics
In genomics, Sanger sequencing acts as a foundational tool, particularly in projects that require high accuracy for smaller-scale sequencing tasks. It is widely utilized for sequencing individual genes or specific regions of interest within a genome. This precision makes it invaluable for various applications, such as gene cloning, mutation detection, and even the sequencing of whole genomes in specific contexts.
- Gene Validation: Researchers ensure that cloned genes are sequenced accurately. This step is vital for functional studies and therapeutic developments.
- Variant Identification: In clinical genomics, this method is used for identifying pathogenic variants responsible for diseases. Accurate identification helps in genetic counseling and patient management.
- Quality Control: For larger sequencing projects, Sanger sequencing serves as a reliable method of quality control, providing a verification layer that high-throughput methods might overlook.
Impact on Personalized Medicine
Sanger sequencing significantly impacts personalized medicine. It enables tailored treatment approaches based on individual genetic profiles. Understanding how specific genetic variations affect drug response can lead to more effective and safer therapies.
This technique helps in:
- Pharmacogenomics: By identifying genetic markers that influence drug metabolism and efficacy, Sanger sequencing aids in designing better medication plans.
- Targeted Therapies: In oncology, it allows clinicians to determine mutations within tumors, guiding the selection of suitable therapies that target these changes.
- Patient Stratification: Genetic insights derived from Sanger sequencing improve patient stratification in clinical trials. This ensures that patients involved are more likely to benefit from the interventions tested.
In summary, Sanger sequencing remains a vital approach in the current research landscape. It reinforces the precision needed in genomic studies while significantly contributing to personalized medicine advancements.
Future Directions in Sequencing Technology
The landscape of sequencing technology is rapidly evolving. Future directions in sequencing technology are crucial for enhancing the efficiency and accuracy of genetic analysis. As the demand for high-throughput and cost-effective sequencing methods increases, researchers must adapt to the emerging trends and techniques. This section explores the integration of emerging technologies and potential innovations that may shape the future of sequencing.
Integration with Emerging Technologies
The integration of emerging technologies into Sanger sequencing can lead to significant advancements. For example, advancements in microfluidics may enhance the efficiency of sample preparation, reducing the time and cost associated with sequencing. Likewise, the combination of Sanger sequencing with CRISPR technology could enable targeted sequencing applications.
Recent developments in artificial intelligence also show promise. AI algorithms can improve data analysis and interpretation, allowing for more accurate variant calling and quicker insights into genomic data. This intersection of artificial intelligence with traditional Sanger methods could streamline processes and enhance the capability of researchers.
Similarly, the incorporation of cloud computing offers researchers the ability to handle large datasets more effectively. This could allow for real-time data sharing and collaboration among scientists globally. Greater collaboration can expedite advancements in genomics and facilitate new discoveries in personalized medicine.
Potential Innovations
Innovative approaches are crucial to address the limitations present in current sequencing technology. One notable potential innovation is the improvement of sequencing chemistry. By developing more efficient fluorescently labeled nucleotides, it may become possible to generate longer reads with greater accuracy. Such advancements could improve the quality of Sanger sequencing results significantly.
Moreover, efforts are underway to enhance the automation of Sanger sequencing. Automation can help in reducing human error and increasing throughput. In addition, creating user-friendly software for data analysis is essential to make Sanger sequencing techniques more accessible to a wider audience.
The integration of nanopore sequencing technologies could also be a game-changer. While still in its early stages, nanopore sequencing offers the possibility of reading longer DNA strands directly, potentially complementing Sanger methods. The synergism between these two technologies may result in more comprehensive genomic insights.
In summary, the future of sequencing technology looks promising. By embracing integration with emerging technologies and fostering innovative approaches, the field of Sanger sequencing can continue to evolve, addressing challenges and opening doors to new possibilities in genetics and molecular biology.
The continuous evolution of sequencing technology will likely lead to breakthroughs in our understanding of genetic diseases and personalized treatment options.
End
In the realm of molecular biology, the significance of Sanger sequencing cannot be overstated. This technique has provided a foundation for many advancements in genetic research and diagnostics. It remains a critical tool, despite the rise of newer technologies.
Summary of Key Concepts
Sanger sequencing, by utilizing the chain termination method, enables precise determination of DNA sequences. Key aspects include its historical significance, the biochemical mechanisms involved, and the advantages that have made it a reliable method.
- Accuracy: Sanger sequencing is known for its high fidelity, making it a trusted method for many applications.
- Simplicity: The procedure remains straightforward compared to other sequencing methods.
- Applications: Its roles in genetic testing, research, and diagnostics highlight its versatility.
The above elements demonstrate why Sanger sequencing continues to be relevant in both academic and clinical contexts.
Final Thoughts on Sanger Sequencing
The future of Sanger sequencing lies in its integration with emerging technologies. While new methods evolve, the principles behind Sanger sequencing provide valuable insights into DNA analysis. The technique is likely to remain a staple in genomic studies where accuracy is paramount. As we move forward, discussing further innovations alongside Sanger sequencing will be essential.
"Sanger sequencing has played a pivotal role in formulating our understanding of genetics, and its implications are still unfolding today."