Understanding Spectra in Microwave Technology
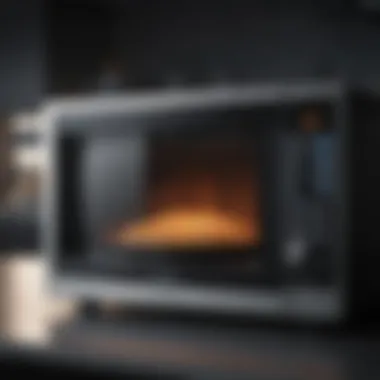
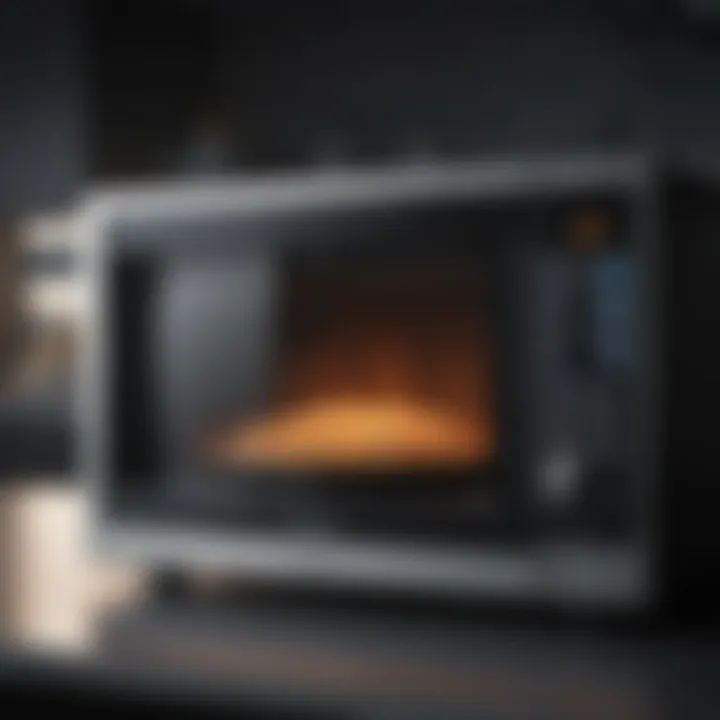
Intro
The study of spectra in microwave technology represents an intersection of fundamental physics and practical application. Microwaves, as a part of the electromagnetic spectrum, are utilized extensively in various scientific and industrial contexts. This article will unpack the components that comprise microwave spectra, their relevance, and the shift in detection methods that have occurred in recent years.
Prologue to Microwave Spectra
Microwave spectra play a crucial role in the field of microwave technology. These spectra provide essential insights into the molecular composition of materials when subjected to microwave radiation. Understanding this fundamental principle is vital in various scientific disciplines, including chemistry, physics, and material science. It enables researchers and professionals to analyze substances at a molecular level with unprecedented precision.
Definition and Importance
Microwave spectra can be defined as the range of electromagnetic radiation emitted by molecules when they transition between energy states. This transition occurs due to the absorption or emission of energy in the microwave region of the electromagnetic spectrum. The ability to interpret these spectra allows scientists to identify the unique signatures of different molecules, which serves multiple functions.
Key benefits of studying microwave spectra include:
- Identification of Substances: By analyzing the spectral data, researchers can determine the presence of specific molecules in a sample.
- Understanding Molecular Behavior: Insights gained from spectra can reveal how molecules interact with each other and participate in chemical reactions.
- Application in Various Fields: From atmospheric sciences to pharmaceuticals, understanding microwave spectra can enhance research and applications in several areas.
Thus, an in-depth understanding of microwave spectra is not simply academic; it has practical implications that advance technology and improve scientific inquiry in diverse fields.
Historical Context
The history of microwave spectroscopy dates back to the mid-20th century when scientists began to realize the potential of microwaves for molecular analysis. Early experiments conducted using Fourier Transform Microwave Spectroscopy opened new avenues for observing molecular spectra. Researchers found that microwaves could be used effectively to probe rotational and vibrational transitions in molecules, leading to unprecedented accuracy in material identification.
Over the decades, advancements in instrumentation, such as the development of better detection methods and data analysis software, have greatly enhanced the capability to study microwave spectra. Today, techniques such as rotational spectroscopy and vibrational spectroscopy allow for detailed molecular characterization and have been incorporated into a variety of scientific fields.
Understanding the historical context of microwave spectra prepares us to appreciate its current applications and future innovations. Recognizing the trajectory of this scientific field reveals the complexity and significance of the issues we face in modern research situations.
Physical Properties of Microwaves
Understanding the physical properties of microwaves is crucial to grasping their application in various scientific fields. Microwaves fall within the electromagnetic spectrum, generally covering wavelengths ranging from one millimeter to one meter, which corresponds to frequencies between 300 gigahertz and 300 megahertz. These properties significantly determine how microwaves interact with materials and how their spectra are analyzed.
Wavelength and Frequency
The relationship between wavelength and frequency is fundamental in microwave technology. The speed of light remains constant; therefore, as the wavelength increases, the frequency decreases, and vice versa. This inverse relationship is expressed in the equation:
[ c = \lambda f ]
Where:
- (c) is the speed of light (approximately 3 x 10^8 meters per second),
- (\lambda) is the wavelength,
- (f) is the frequency.
In practical terms, different applications of microwaves exploit specific wavelengths and frequencies based on their unique benefits:
- Longer wavelengths can penetrate materials more effectively, which is useful in radar and some telecommunications.
- Shorter wavelengths translate to higher resolution in imaging techniques, such as microwave spectroscopy, enabling detailed analysis of molecular structures.
Understanding these properties allows scientists and engineers to optimize microwave systems for specific applications, making this knowledge pivotal.
Energy Levels and Transitions
Microwave spectroscopy is deeply interlinked with the concepts of energy levels and transitions. The energy levels of molecules dictate how they absorb and emit microwave radiation. Each molecule has specific vibrational and rotational energy states. When exposed to microwave radiation, molecules can transition between these states, resulting in the absorption of energy.
This transition can be categorized as follows:
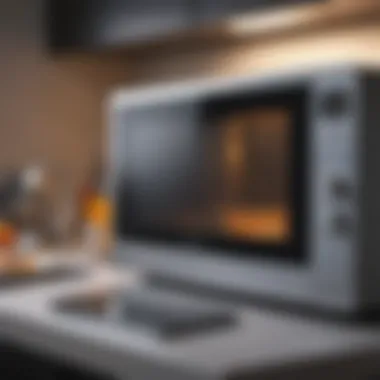
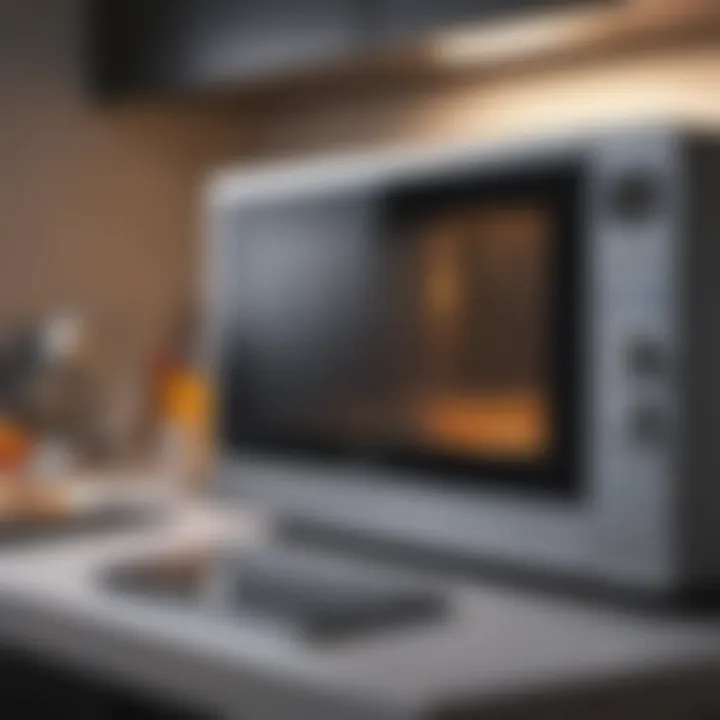
- Rotational transitions, which typically occur at lower energies and involve changes to the rotational state of the molecule.
- Vibrational transitions, which occur at higher energies and involve changes to molecular vibrations.
A thorough understanding of these transitions is necessary to interpret the spectra generated during microwave analyses. The peaks observed in a spectrum correspond to specific transitions. By examining these peaks, researchers can deduce a wealth of information regarding molecular composition, structure, and behavior.
The precise measurement of transitions enables not only the identification of substances but also the assessment of environmental conditions such as temperature and pressure.
In summary, grasping these fundamental physical properties of microwaves is essential to leverage their capabilities effectively in both theoretical and practical applications. Increasing the understanding of wavelength, frequency, energy levels, and transitions allows researchers and professionals to innovate and refine microwave technology to meet current and future challenges.
Spectroscopic Techniques in Microwave Analysis
The analysis of microwave spectra employs a range of spectroscopic techniques that are crucial for understanding material properties and interactions. In the field of microwave technology, knowing how to effectively analyze spectra is essential for both research and practical applications. Spectroscopic techniques enhance the capability to resolve various molecular interactions and contribute to both theoretical and applied sciences. Different methods offer unique insights, making this topic essential for students and professionals alike.
Types of Microwave Spectroscopy
Rotational Spectroscopy
Rotational spectroscopy is a method that focuses on the rotation of molecules when exposed to microwave radiation. The technique exploits the transitions between rotational energy levels of molecules. By measuring the frequencies at which these transitions occur, researchers can derive vital molecular characteristics. This technique is particularly beneficial for identifying molecular structures and measuring bond lengths.
A key characteristic of rotational spectroscopy is its sensitivity to the rotational states of the molecule. Because each molecule has a distinct rotational spectrum, this method allows for precise identification. One significant advantage is its ability to analyze gas-phase molecules in near ambient conditions, facilitating broader applications across different fields. However, it has limitations regarding the size of the molecule and is generally less informative for larger, more complex biometric molecules.
Vibrational Spectroscopy
Vibrational spectroscopy differs from its rotational counterpart by focusing on vibrational transitions within molecules. This method analyzes the interactions that occur when a molecule vibrates in response to microwave emission. The unique aspect of vibrational spectroscopy is its capability to provide insights into the molecular vibrations that denote structural properties such as bond strength and molecular geometry. It is widely regarded as a popular choice due to its rich analysis of vibrational modes, which can be especially useful in characterizing functional groups in organic compounds.
One advantage of vibrational spectroscopy is its versatility across various states of matter, including gases, liquids, and solids. Conversely, it can be less effective in distinguishing between closely related species, making it important for researchers to be aware of possible overlaps in spectral information. Understanding these dynamics is crucial when evaluating the effectiveness of vibrational spectroscopy in a given scientific investigation.
Instrumentation and Setup
Proper instrumentation and setup play a critical role in obtaining quality microwave spectra. This aspect of microwave spectroscopy encompasses the equipment used to emit and detect microwave radiation, along with the arrangements needed for optimal measurement. Key components often include a microwave source, a sample chamber, and detectors designed to capture spectral data.
Calibration of instruments is essential for accurate results. The complexity of designing experimental setups necessitates thorough attention to environmental conditions, such as pressure and temperature, as they can significantly influence spectral output. By understanding and controlling these parameters, researchers can ensure consistency and reliability in their findings.
Applications of Microwave Spectra
The applications of microwave spectra are vital in many scientific disciplines. Their importance spans fields such as atmospheric studies, material science, and biological research. Understanding how microwave spectra can be applied aids in many complex analyses. It also enhances critical research across various sectors. The benefits include improved detection methods, accurate data interpretation, and fostering advancements in technology. Moreover, microwave spectra offer unique insights into molecular structures and interactions, benefiting both theoretical and applied sciences.
In Atmospheric Studies
Microwave spectra play a crucial role in atmospheric studies. They provide important information about the composition and behavior of gases in the Earth's atmosphere. This data is essential to understand climate change, air quality, and weather patterns. By utilizing microwave spectroscopy, scientists can identify specific gases and their concentrations. This method is particularly effective for measuring water vapor and greenhouse gases, which are pivotal in climate models.
In addition, the remote sensing capabilities of microwave spectra allow for global monitoring of atmospheric changes. This includes tracking pollution events, studying weather fronts, and monitoring stratospheric conditions. The data derived from these analyses assists in developing accurate predictive models. Furthermore, this information influences environmental policy and helps mitigate the effects of climate change.
In Material Science
The application of microwave spectra in material science is significant. This technique provides insights into molecular vibrations and rotations. It facilitates the study of material properties at the molecular level. Microwave spectroscopy can be used to analyze the structural characteristics of various materials, including polymers, metals, and ceramics. The importance of material characterization cannot be overstated. It informs product development and quality control across industries.
In addition, with microwave spectra, researchers can examine phase transitions and interactions within materials. It helps to determine properties such as conductivity, magnetic behavior, and thermal characteristics. Increased understanding of these properties can lead to the design of innovative materials. Such materials can improve electronic devices and other applications across technology sectors.
In Biological Research
In biological research, microwave spectra offer insights that can enhance our understanding of complex biological systems. This includes analyzing molecular interactions in proteins, nucleic acids, and other biomolecules. Understanding these interactions aids in drug design and the development of therapeutic strategies. By assessing how molecules absorb and emit microwaves, researchers can identify structural and dynamic properties of biological samples.
Furthermore, microwave spectroscopy is valuable for non-destructive testing of biological tissues. It allows for real-time analysis without compromising the integrity of the sample. This capability is beneficial in medical diagnostics, enabling early detection of diseases at a molecular level. The integration of microwave spectroscopy into biological research is, therefore, essential for advancing our comprehension of life sciences.
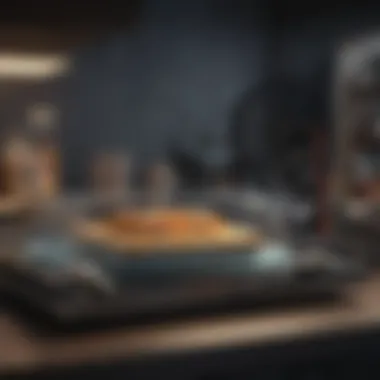
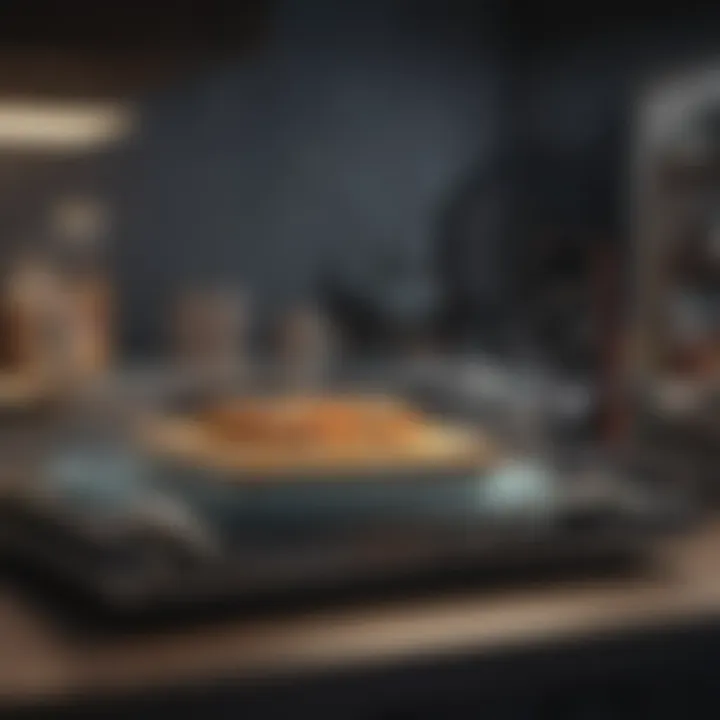
"Microwave spectroscopy exemplifies the intersection of technology and various scientific disciplines, proving invaluable in both analysis and research."
The applications of microwave spectra extend across numerous fields, showcasing their profound impact on modern science. Understanding these spectra not only enhances our fundamental knowledge but also paves the way for innovation across disciplines.
The Role of Computational Methods
Computational methods are becoming an essential part of understanding spectra in microwave technology. These methods provide tools to predict and analyze spectral data, which allows researchers to unravel complex phenomena. They enable scientists to simulate how molecules interact with microwaves, thus predicting their spectral characteristics without needing extensive experimental work. The significance of computational methods is highlighted in their ability to provide detailed insight into molecular behavior under various conditions. This leads to advanced analysis, revealing information that might not be readily obtained through traditional experimental approaches.
Modeling Microwave Spectra
Modeling microwave spectra involves using computational tools to simulate the interaction of electromagnetic waves with matter. This process requires understanding both the physical principles behind microwave spectroscopy and the software tools available for simulation. The most common techniques include quantum mechanical modeling, molecular dynamics simulations, and statistical methods.
- Quantum Mechanical Modeling: This approach utilizes principles of quantum mechanics to predict energy levels and transitions of molecules. Software like Gaussian or GAMESS allows researchers to compute rotational and vibrational spectra effectively.
- Molecular Dynamics Simulations: These simulations provide insight into time-dependent behaviors of molecules when exposed to microwave radiation. They help ascertain how molecular motion influences the observed spectra.
- Statistical Methods: Statistical approaches leverage existing experimental data and mathematical models to analyze and predict spectral features. They can help identify trends and correlations that are not evident through basic observation.
The outcomes of these models can be significantly beneficial. They not only serve as a guideline for experimental designs but can also help validate findings from physical experiments. Additionally, they create an opportunity for innovative developments in microwave technology, as models can explore the limits of current methodologies and suggest new avenues for research.
Data Analysis Techniques
The analysis of data produced by microwave spectroscopy is a critical process that ensures the reliability of results. Effective data analysis techniques are necessary for interpreting spectral information accurately. These techniques often involve a mix of qualitative and quantitative methods, including:
- Fourier Transform Techniques: This method converts time-domain data into frequency-domain data, providing a clearer view of spectral lines.
- Curve Fitting: By fitting experimental data to theoretical models, researchers can extract precise parameters such as peak positions and intensities.
- Machine Learning: Emerging techniques involving machine learning are being adopted to recognize patterns within large datasets. They can significantly enhance the speed and accuracy of data interpretation.
The integration of these data analysis methods leads to a comprehensive understanding of the complexities involved in microwave spectra. This understanding is essential for deriving meaningful conclusions about molecular properties and behaviors.
Challenges and Limitations
In any scientific field, understanding challenges and limitations is crucial. This section focuses on how intricate the study of microwave spectra can be. The complexities involved not only reflect on the technologies available but also on the interpretations of the data that are gathered. These challenges, while significant, serve as opportunities for growth and innovation.
Instrumentation Constraints
Instrumentation is a core aspect of any spectral analysis, and microwave technology is no exception. The precision and capabilities of the instruments affect the accuracy of the data collected. Limitations in sensitivity, resolution, and range can lead to significant challenges in obtaining reliable results.
- Sensitivity: Microwaves often involve weak signals that can be difficult to detect. Instruments must be sensitive enough to discern these signals from background noise, failing which, the data may reflect inaccuracies.
- Resolution: The ability to resolve closely spaced spectral lines is essential. If the instrument lacks sufficient resolution, it may result in overlapping peaks, making interpretation problematic.
- Operational Range: Different materials exhibit spectra across various wavelength ranges. Instruments must be designed to operate effectively over these ranges, otherwise critical data may be missed.
The evolution of microwave instrumentation continues, but certain constraints remain, posing obstacles to researchers. Improvements are ongoing, yet understanding these constraints is vital for anyone working in the field.
Interpretative Difficulties
Interpreting results from microwave spectroscopy is another major hurdle. As the interpretation of spectra requires both technical knowledge and practical experience, varied factors can lead to confusion or misinterpretation.
- Complexity of Spectra: Spectral lines can be influenced by numerous factors including temperature, pressure, and environmental conditions, leading to complex spectra.
- Model Dependency: The models used to predict spectra must be validated. If these models are incorrect or incomplete, the interpretation may lead to erroneous conclusions.
- Data Overlap: Peaks in the spectra can overlap, especially in mixtures, complicating the identification of distinct components.
Understanding these interpretative difficulties is essential for accurate analysis and clear conclusions.
"Challenges in instrumentation and interpretation must be acknowledged to advance microwave technology effectively."
Recent Advances in Microwave Technology
Recent advances in microwave technology have catalyzed significant progress in several scientific fields. The evolution of spectroscopic techniques and integration with other analytical methods represents a vital juncture for researchers and technologists alike. As microwave technology continues to mature, it brings forth enhanced accuracy, efficiency, and versatility in data acquisition and analysis. This section delves into the critical innovations and integrations that redefine the landscape of microwave applications.
Innovations in Spectroscopy
Innovative approaches to spectroscopy have reshaped the way researchers analyze and interpret microwave spectra. Recent developments focus on miniaturized instrumentation, which allow for portability without sacrificing performance. Techniques such as microfluidic devices are being utilized to analyze samples with minimal quantities of material. This reduces waste and enables real-time analysis in dynamic environments.
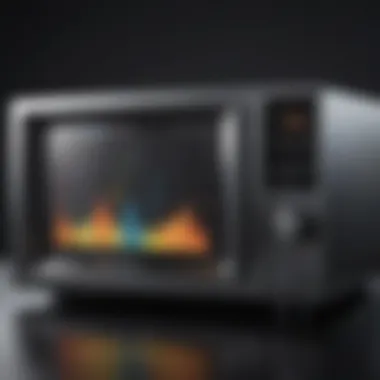
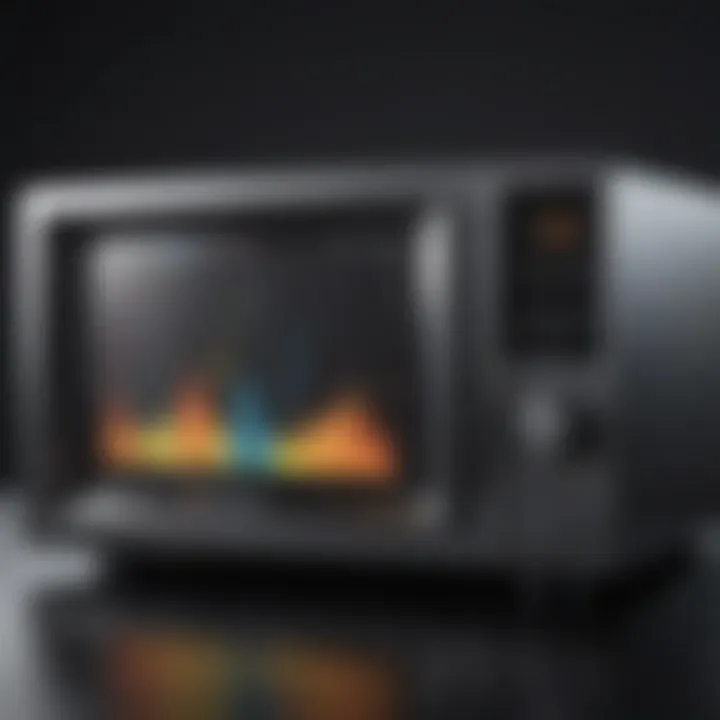
Furthermore, advancements in quantum cascade lasers and atomic magnetometers have improved the sensitivity and specificity of measurements. The ability to detect subtle changes in molecular environments has opened doors for precision applications in chemistry and biology. For example, continuous wave microwave spectroscopy has gained traction in identifying and characterizing complex mixtures.
Recent studies highlight the integration of machine learning algorithms in spectroscopy. These algorithms assist in interpreting complex datasets, making it possible to extract meaningful patterns and relationships that would have been previously undetectable. As a result, researchers can accelerate their discoveries and drive more informed and actionable insights into their work.
Integration with Other Analytical Techniques
Integrating microwave spectroscopy with other analytical techniques creates a synergistic effect, enhancing the capabilities of researchers. For instance, coupling microwave spectroscopy with mass spectrometry has gained popularity. This combination allows for the simultaneous analysis of structural and compositional characteristics of samples, providing a comprehensive view of their properties.
In environmental monitoring, microwave techniques are being paired with gas chromatography for enhanced pollutant detection. By combining the strengths of these two methods, scientists improve sensitivity and response times, allowing for quicker assessments that are critical for public health and safety.
Moreover, the integration of computational methods with microwave analysis provides powerful predictive capabilities. This marriage of experimental and computational approaches creates a robust framework for understanding complex molecular interactions and dynamics. Researchers can simulate microwave interactions with different materials, helping design experiments more efficiently.
Key takeaway: The advancements in microwave technology, including innovations in spectroscopy and integrations with other methods, are pivotal for the future of scientific research. They not only improve measurement capabilities but also facilitate the exploration of new applications across various fields.
Through sustained progress in these areas, microwave technology will continue to play a crucial role in driving forward research and innovation. The momentum generated from these advances sets a promising trajectory for future explorations in applied sciences.
Future Directions in Microwave Research
Future directions in microwave research have significant implications for both scientific inquiry and practical application across various industries. As microwave technology advances, it opens up new horizons for exploration, enhancing our understanding and utilization of microwave spectra. Researchers are increasingly focusing on integrating microwave spectroscopy with other analytical techniques. This integration is vital, as it enables more comprehensive analysis and enhances data accuracy. The importance of this field lies not only in technological innovation but also in addressing global challenges, such as energy efficiency and environmental monitoring.
Emerging Trends
Emerging trends in microwave research demonstrate a shift towards interdisciplinary approaches. This includes the convergence of microwave technology with nanotechnology, materials science, and quantum computing. Researchers are exploring how novel materials can enhance microwave signal processing and detection.
Some specific trends include:
- Development of new materials: Research is being conducted on materials that can improve the efficiency and sensitivity of microwave sensors. These materials could lead to enhanced microwave devices and promote better interaction with electromagnetic waves.
- Machine learning applications: The use of artificial intelligence and machine learning algorithms in analyzing microwave spectra data. Such techniques allow for swift data processing and increased predictive accuracy in various applications.
- Real-time monitoring systems: Advances in wireless technologies are enabling real-time monitoring of complex systems. This is particularly applicable in environmental science, where continuous data collection about atmospheric conditions is crucial.
The incorporation of these trends into microwave technology holds the potential to redefine how we conduct research and develop applications in the field.
Potential Applications in Industry
Potential applications of microwave research in industry are vast and varied. Industries such as healthcare, telecommunications, and food safety are gearing up to harness these advancements.
Some noteworthy applications include:
- Healthcare: Microwaves hold promise in medical diagnostics and treatment. For example, microwave imaging is being explored for detecting tumors and monitoring biological systems. Improvements in this area could lead to non-invasive diagnostic techniques that are both efficient and cost-effective.
- Telecommunications: As demands on data speed and infrastructure grow, microwave technology can be pivotal in developing faster communication systems. Innovations such as microwave photonics are pushing boundaries, promising to enhance bandwidth and signal clarity.
- Food Safety: Microwave technology is being utilized to ensure food safety by improving methods for detecting contaminants. Research focuses on microwave sensing which could help in identifying spoilage or harmful pathogens in products.
As microwave research continues to evolve, the industrial landscape is likely to see significant transformation. The ongoing advancements will not only enhance productivity but also foster innovations that cater to the dynamic needs of society.
Finale
The conclusion serves as a critical synthesis of the information presented in this article. It encapsulates the relevance of microwave spectra within various scientific domains, emphasizing their role in enhancing our understanding of matter and energy interactions. By summarizing key insights, the conclusion provides a clear and concise recollection of the main arguments and evidence discussed in the previous sections.
Summary of Key Points
Throughout the article, several core elements were outlined:
- Microwave Spectra Basics: Understanding the definitions and principles of microwave spectra is foundational. This knowledge forms the basis for exploring more advanced applications and technologies.
- Techniques of Analysis: Rotational and vibrational spectroscopies are essential techniques within microwave technology. Their applications in assessing molecular structure and behavior are revolutionary.
- Applications across Disciplines: Microwave spectra have found significant applications in atmospheric studies, material science, and biological research, showcasing their versatility.
- Role of Computational Methods: Modeling and analysis techniques are increasingly employed to interpret microwave spectra effectively.
- Future Directions: Emerging trends indicate ongoing integration with other analytical methods, promising even greater advancements.
These points illustrate the multifaceted applications and relevance of microwave spectra in practical and theoretical contexts.
Implications for Future Research
The field of microwave technology will undoubtedly continue to evolve. Several implications arise from the findings discussed:
- Enhanced Accuracy and Techniques: Research can focus on innovating current spectroscopic techniques, aiming for heightened accuracy and reliability.
- Expanded Applications: Investigating new fields where microwave spectra can be instrumental will open pathways for significant breakthroughs, particularly in environmental and health sciences.
- Interdisciplinary Collaboration: Future studies may benefit from an interdisciplinary approach, combining insights from physics, chemistry, biology, and engineering to leverage the full potential of microwave technology.
In summary, the ongoing advancements in microwave spectra promise to not only enhance existing scientific frameworks but also pave the way for new discoveries and practical solutions in various industries.