Exploring the Utility of ICP Instruments in Metal Analysis
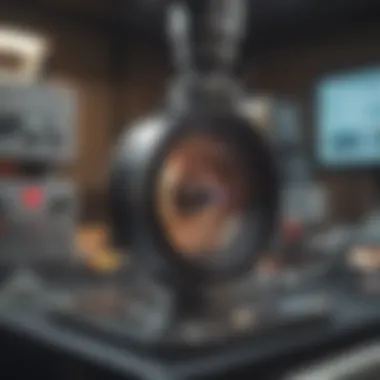
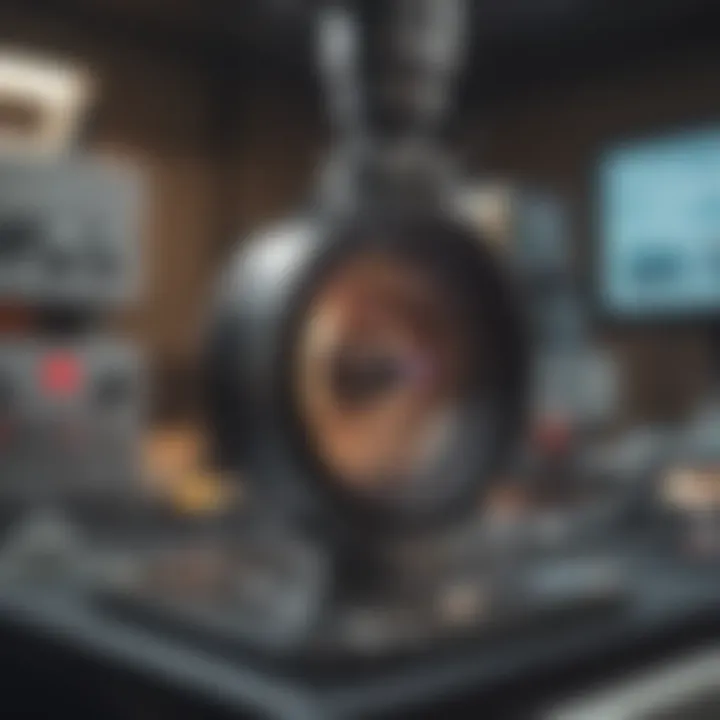
Intro
Inductively Coupled Plasma (ICP) instruments have become critically important in the field of metal analysis. This is mainly due to their ability to provide accurate and precise measurements across a wide range of metal types. The adoption of ICP technology is markedly increasing in both academic research and industrial applications. By understanding the principles that underpin this technology, as well as the methodologies employed, professionals can appreciate its significance.
ICP techniques stand apart when compared to traditional metal analysis methods. The precision they offer is valuable for numerous scientific investigations. Therefore, this article aims to provide a deep insight into various aspects of ICP technology. The focus will be on the methodologies and approaches used for metal analysis, along with its significance in contemporary research and industry.
The content will also touch on current trends that highlight innovation in ICP instrumentation and its interdisciplinary connections. These factors contribute to how ICP continues to shape the landscape of metal analysis in various sectors.
Research Overview
Methodological Approaches
ICP technology involves the conversion of a sample into an aerosol, followed by its ionization in a plasma state. This method ensures that the elemental composition of metals can be determined efficiently. The most common approaches include
- ICP Optical Emission Spectroscopy (ICP-OES)
- ICP Mass Spectrometry (ICP-MS)
ICP-OES utilizes light emissions from ionized atoms to determine their concentrations, while ICP-MS employs mass spectrometry to analyze isotopes. These methods provide sensitivity and selectivity unmatched by many other techniques.
Key considerations in selecting an ICP method include:
- Sample type and concentration
- Required detection limits
- Possible interference from other elements
Significance and Implications
The significance of ICP instruments extends well beyond mere measurements. They have a profound impact on various applications. For instance, in environmental science, ICP allows for the detection of trace metals in water samples, essential for water quality assessments. In the mining industry, it assesses the metal content of ores, informing extraction and processing strategies.
Benefits of ICP technology include:
- Rapid analysis time
- High throughput capabilities
- Detailed elemental data
Metals have diverse roles in different sectors. Therefore, ICP provides essential insights that inform decision-making processes, contributing to scientific advancements and efficiency.
Current Trends in Science
Innovative Techniques and Tools
The landscape of metal analysis continues evolving, driven by technological advancements in ICP instrumentation. Newer developments focus on micro-sampling techniques and improved sensitivity through advanced detectors. The incorporation of robotics for sample handling is also a notable trend, reducing human error while increasing throughput rates.
Interdisciplinary Connections
ICP technology is not confined to one field; it has found applications in various disciplines including:
- Forensic science: Used for analyzing trace evidence.
- Food safety: Monitoring metal contaminants in food products.
- Material science: Characterization of metal alloys.
The interdisciplinary nature of ICP technology enhances collaboration across fields, leading to a deeper understanding of metal interactions within various contexts.
The application of ICP instruments continues to redefine the standards for metal analysis, allowing professionals in diverse disciplines to achieve groundbreaking results.
Prolusion to ICP Technology
Inductively Coupled Plasma (ICP) technology serves as a cornerstone in the realm of metal analysis, exhibiting a wide range of applications due to its exceptional sensitivity and capability to analyze multiple elements simultaneously. Understanding ICP technology is essential for both academic and professional disciplines, as it significantly influences how metals are assessed in various environments.
ICP technology transcends traditional analytical methods by offering rapid and reliable elemental analysis. Its ability to analyze trace metals with high precision makes it indispensable in fields such as environmental monitoring and food safety.
Furthermore, the technological evolution of ICP presents opportunities for advancements in methodologies, which can further enhance analysis effectiveness. The intersection of academia and industry in utilizing ICP underlines its relevance. With ongoing developments, it is crucial to grasp both its foundations and its potential for future applications.
What is Inductively Coupled Plasma?
Inductively Coupled Plasma, commonly referred to as ICP, is a state of matter where ionized gas is created by using an electromagnetic field. The technique enables the rapid excitation of atoms for emission spectrometry, primarily used to detect metals within a sample. The ionization process occurs when a gas, typically argon, is energized by radio frequency (RF) energy, creating a high-temperature plasma.
The significance of plasma in ICP lies in its intense heat, which can exceed 10,000 Kelvin. This extreme temperature allows for the effective atomization and ionization of elements present in the sample. As a result, the electromagnetic radiation emitted from excited atoms can be analyzed to provide elemental content accurately. The broad usability of ICP extends to diverse fields including geology, material science, and biochemistry.
Historical Development and Evolution
The origins of ICP technology trace back to the 1960s, highlighting a rapid evolution from rudimentary spectroscopy methods. Early inductively coupled methods were primarily utilized for analytical applications. Over the decades, significant innovations have emerged, improving accuracy, speed, and usability.
In the 1980s, the introduction of the ICP mass spectrometry (ICP-MS) elevated the analytical capabilities of the technology. This advancement allowed for quantification at trace levels, making it possible to analyze even the most minute quantities of metal from a wide array of matrices. As technology progressed into the 21st century, enhancements in instrument design, automation, and software capabilities further expanded the reach and effectiveness of ICP.
The trajectory of ICP signifies not only a technological achievement but also a response to critical analytical needs across numerous disciplines.
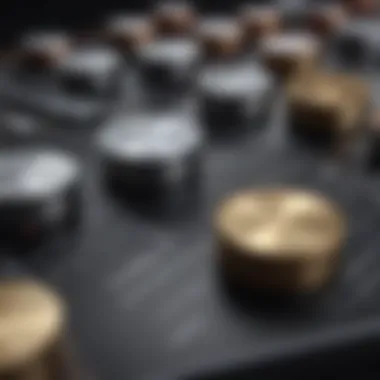
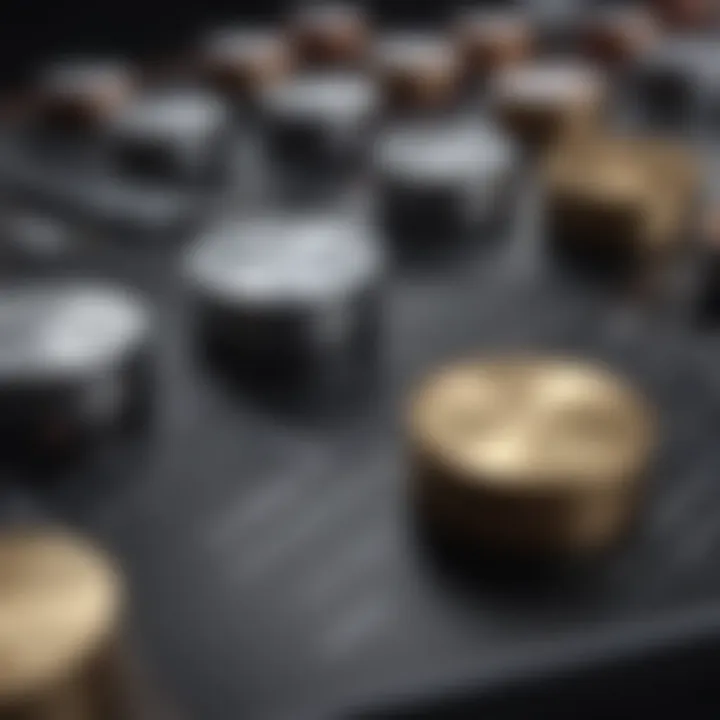
"The evolution of ICP technology reflects an ongoing commitment to precision and efficiency in metal analysis, shaping both academic research and industrial application."
As ICP continues to evolve, understanding its basis and development becomes essential for leveraging its capabilities effectively.
Principles of ICP Operation
The principles of Inductively Coupled Plasma (ICP) operation are essential in understanding how these instruments function in metal analysis. Their significance cannot be understated, as they form the backbone of the analytical capabilities that ICP provides. The operational principles dictate not only the efficiency of the analysis but also the quality and accuracy of the results obtained. Therefore, a deep dive into these principles is crucial for anyone involved in the relevant fields, such as environmental monitoring, food safety testing, and industrial applications.
Fundamental Mechanisms
At the core of ICP technology lies the generation of plasma. Plasma is a highly ionized gas composed of ions and free electrons. This state is achieved via the application of radio frequency (RF) energy administered to a gas, typically argon. The argon atoms get ionized and, as a result, produce a cloud of charged particles. This ionized gas serves as the medium to facilitate the excitation of the sample introduced into the plasma.
Key points include:
- The ionization of argon leads to extremely high temperatures in the plasma, often exceeding 10,000 Kelvin. This temperature is sufficient to excite metal atoms to higher energy states, thereby enhancing the detection process.
- The excitation energy allows for the detection of multiple metal elements simultaneously, making ICP a multi-element analytical technique.
- Understanding how these mechanisms interact with different metal samples is vital for optimizing detection limits and improving the robustness of the analysis.
This fundamental knowledge shapes how modifications and upgrades in ICP technology can lead to significant advancements in metal analysis.
Plasma Generation and Maintenance
Plasma generation and maintenance are critical components of the ICP process. The process begins with a high-frequency oscillating electric field in a quartz torch, producing a plasma that is sustained by a continuous flow of argon gas. The stability and consistency of this plasma are crucial for obtaining reliable and reproducible results during metal analysis.
The maintenance of such a plasma is influenced by several factors:
- Flow Rate of Argon: The rate at which argon is introduced influences the density and stability of the plasma. Too low a flow can cause instability, while too high can disperse the sample too quickly, reducing sensitivity.
- RF Power Input: The amount of power supplied to the plasma directly correlates to its temperature and ionization degree. This needs to be fine-tuned based on the specific requirements of the sample and metal analysis.
- Torch Configuration: The design of the torch affects how well the sample is introduced into the plasma and how consistently the plasma can be maintained.
Understanding these operational aspects not only improves analytical performance but also aids in troubleshooting any issues that may arise during metal analysis.
"The stability of plasma is foundational to the accuracy of ICP analysis; alterations in operational parameters can lead to significant deviations in results."
Thus, mastery of the principles behind ICP operation empowers researchers, educators, and professionals in achieving precise and nuanced metal analyses.
ICP Instrumentation Components
The framework of Inductively Coupled Plasma (ICP) technology relies on its core instrumentation components. These elements are crucial for the effective operation and analytical performance of ICP systems in metal analysis. Understanding these components not only enhances the user’s ability to interpret results but also identifies maintenance needs and troubleshooting methods. Each part plays a role in ensuring the system can efficiently analyze samples and deliver precise data.
Key Components of ICP Systems
ICP systems consist of several essential components that allow for the successful generation of plasma and subsequent analysis of metals. The main components include the power supply, plasma torch, nebulizer, spray chamber, and detector. Each of these parts must work together harmoniously to achieve reliable analytical results.
- Power Supply: This is a high-frequency generator that excites the argon gas, allowing it to form a stable plasma. It is essential for sustaining the energy needed for the ICP process.
- Plasma Torch: Often made from quartz, the torch facilitates the ionization of analytes. Its structure includes a central channel for the gas flow, surrounded by cooling water to prevent overheating.
- Nebulizer: This component transforms liquid samples into aerosol for easier detection. The quality of nebulization directly impacts the efficiency of the analysis.
- Spray Chamber: The spray chamber acts as a separator for larger droplets that do not evaporate properly, allowing only the finest particles to enter the plasma.
- Detector: Finally, the detector measures the emission of light from ionized elements. Options such as photomultiplier tubes or CCD detectors are common choices depending on the desired sensitivity and resolution.
Sample Prolusion Techniques
The method used to introduce samples into the ICP system is determining for the analysis quality. Each introduction technique has its own specific advantages and drawbacks, influencing the overall results they can provide. There are three primary techniques: liquid, solid, and gas sample introduction.
Liquid Sample Preface
Liquid sample introduction is the most prevalent method in ICP analysis. It involves converting a liquid sample into an aerosol that can be introduced into the plasma. This method is well-regarded for its reliability.
- The key characteristic of liquid sample introduction is its ability to easily handle a wide range of concentrations and types of samples.
- Liquid introduction is popular because it allows for straightforward preparation and minimizes sample loss. The unique feature is that it efficiently nebulizes liquid samples, resulting in a fine aerosol while providing good transport efficiency to the plasma.
- Some advantages include high sensitivity and straightforward calibration. However, it may struggle with very high viscosity liquids if proper care is not taken.
Solid Sample Prelims
Solid sample introduction is not as common but can be valuable when analyzing solid materials. This method involves using a solid sampling device, like a furnace or laser ablation, to convert solids into vapors or aerosols for analysis.
- A key characteristic of this technique is its ability to analyze materials directly in their solid form, which can be beneficial for certain applications where sample alteration is undesirable.
- This choice is beneficial as it reduces sample preparation time and minimizes contamination. A unique feature is that it allows for the analysis of heterogeneous samples.
- One significant disadvantage is that it typically requires longer analysis times and may need extensive method development compared to liquid introduction.
Gas Sample Prelims
Gas sample introduction is less common than the other methods but is utilized in specific applications. It involves injecting gaseous samples directly into the ICP system.
- The key characteristic of gas introduction is its ability to handle volatile organic compounds (VOCs) effectively. This method can analyze samples that are typically hard to examine using traditional techniques.
- It is a beneficial choice for applications in environmental monitoring where gas phase samples are prevalent. Notably, it allows for direct analysis without the need for conversion into another state.
- However, the unique feature of gas sample introduction comes with limitations, such as the need for proper calibration and the potential for lower sensitivity in comparison to liquid methods.
Applications of ICP in Metal Analysis
The exploration of Inductively Coupled Plasma (ICP) technology in the metal analysis landscape reveals a plethora of applications that highlight its significant role across various sectors. Understanding the applications of ICP provides insight into its benefits, operational efficacy, and relevance to contemporary analysis needs. Metal analysis is pivotal in multiple fields, including environmental science, food safety, and industrial processes. Hence, knowing how ICP instruments operate in these domains can enhance both the quality of analysis and assurance of accuracy.
Trace Metal Analysis
Trace metal analysis involves measuring minute concentrations of metals in different matrices. This application is vital in fields such as environmental monitoring and health assessments. ICP’s capacity for high sensitivity makes it particularly effective for detecting trace metals, which may have significant ecological and health implications, even at low levels.
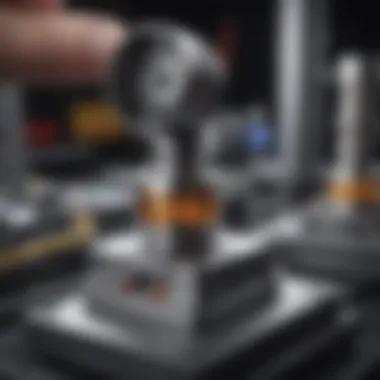
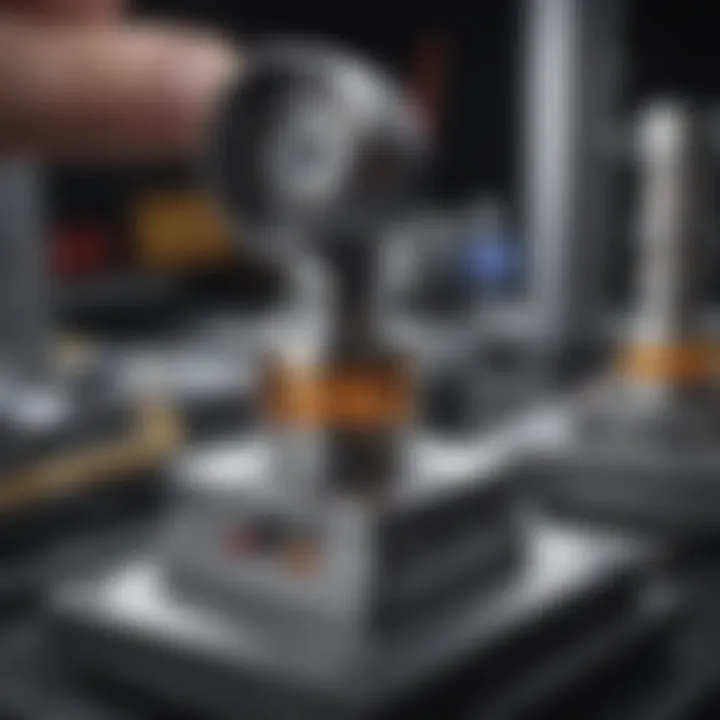
Modern ICP instruments can accurately quantify elements like lead, mercury, and arsenic, which have critical safety and regulatory standards. The analytical sensitivity achieved by ICP technology is often better than traditional methods such as Atomic Absorption Spectroscopy (AAS). With a detection limit that can reach the picogram per liter range, ICP is quite adept in providing precise data that can influence environmental health policies and remediation efforts.
Environmental Monitoring
In the realm of environmental monitoring, the significance of ICP instruments cannot be overstated. They play a crucial role in assessing pollution levels in air, soil, and water. The ability to analyze multiple elements simultaneously aids in developing comprehensive environmental assessments. This is particularly important when tracking contaminants or studying bioaccumulation of metals in ecosystems.
Key benefits of using ICP for environmental monitoring include:
- Rapid Assessment: ICP technology enables quick turnaround times for analysis, allowing for immediate decision-making.
- Multi-Element Capability: Standard environmental analysis often necessitates the assessment of various elements. ICP can simultaneously measure numerous metals, improving efficiency and reducing costs.
- High Precision: The high resolution in measurement contributes to better understanding trends in pollution and helps enforce environmental regulations.
Food Safety Testing
Food safety is another critical application area for ICP technology. As global standards for food quality tighten, the importance of reliable trace metal testing has increased. ICP instruments provide essential data to ensure food products do not exceed permissible limits of harmful metals.
This is important not only for consumer safety but also for compliance with health regulations. Regular monitoring of metals such as cadmium and lead in agricultural products and imported foods is significant in protecting public health. The ability to validate food sources through methodical analysis reinforces the integrity of supply chains.
"Food safety is often about understanding potential hazards before they become critical issues. ICP instruments help make that understanding solid with data."
The integration of ICP technology in metal analysis underlines its role in ensuring safety, compliance, and quality across various domains. With advancements continuing to emerge, the applications of ICP will likely expand further, demonstrating the instrument's versatility and essentiality in analytical chemistries.
Advantages of ICP over Traditional Methods
Inductively Coupled Plasma (ICP) instruments stand at the forefront of metal analysis due to their distinctive advantages when compared to traditional methods. The significance of these advantages lies not only in their technical prowess but also in the considerable implications for efficiency, accuracy, and versatility in analytical laboratories. With varying requirements across different fields, understanding these advantages provides a strong basis for choosing ICP as the preferred analytical technique.
Sensitivity and Detection Limits
One of the most compelling benefits of ICP technology is its unparalleled sensitivity. ICP instruments can detect metals at parts per billion (ppb) levels, making them ideal for trace metal analysis. This high sensitivity is crucial in contexts such as environmental monitoring and food safety. Traditional methods like flame atomic absorption spectroscopy (FAAS) may struggle to achieve the same detection limits, limiting their applicability in scenarios where minute concentrations of metals are of concern.
The ability to identify trace amounts ensures that critical insights into contamination and metal presence can be deciphered accurately. Moreover, advancements in ICP-MS (Mass Spectrometry) have further pushed the sensitivity thresholds even lower, enabling the detection of ultra-trace levels. This increased sensitivity allows researchers and industry professionals to make informed decisions based on detailed metal composition data.
Multi-Element Capability
The multi-element capability of ICP instruments is another significant advantage. Unlike traditional techniques, which often require separate analyses for different metals, ICP can detect several elements simultaneously. This characteristic enhances the efficiency of analyses and reduces the overall costs of testing in laboratories.
The simultaneous detection is achieved through the use of advanced plasma technology, allowing for the effective measurement of various metals across a wide spectrum of concentrations. This feature is particularly advantageous in industries where multiple elements need to be monitored, such as mining, pharmaceuticals, and food processing. The ability to gather comprehensive datasets in a single run means faster results and improved workflow.
Rapid Analysis Time
Timeliness is an essential consideration in metal analysis, and ICP instruments excel in this area as well. They offer rapid analysis times compared to traditional methods, where waiting for sequential measurements can extend testing periods significantly. Using ICP, samples can be prepared and analyzed swiftly.
For instance, an ICP analysis can yield results in a matter of minutes as opposed to hours or even days, which is often the case with other techniques. This rapid turnaround is critical for industries that depend on immediate results for quality control, compliance, or decision-making. The efficiency brought about by ICP technology thus facilitates not only faster workflows but also enhances productivity in a demanding laboratory environment.
The rapid advancement in ICP technology highlights its relevance in modern analytical practices, moving beyond limitations posed by older methods.
Challenges in ICP Metal Analysis
In the realm of metal analysis, utilizing Inductively Coupled Plasma (ICP) instruments brings both significant benefits and certain challenges. Understanding these challenges is crucial for researchers and industry professionals striving for accurate and reliable results. This section discusses specific factors that can impact the effectiveness of ICP techniques. Two primary challenges arise: interference issues and the need for regular instrument calibration and maintenance. Addressing these challenges is vital to enhance the precision and overall reliability of ICP methods.
Interference Issues
Interference in ICP analysis can significantly affect the accuracy of the results. There are several types of interference that analysts must consider. These include spectral interference, where different elements emit light at similar wavelengths, leading to potential overlap. Another common type is ionization interference, which occurs when the presence of certain elements changes the ionization balance in the plasma, thus affecting the signal observed for other elements. Additionally, physical interferences related to sample matrix effects can influence the analytical outcome.
Addressing these issues often requires careful sample preparation and the use of internal standards. Internal standards can help normalise variations in the signal due to interferences by compensating for changes in the plasma conditions. Moreover, selecting the appropriate method for data analysis can further mitigate these challenges, allowing analysts to derive more accurate quantitative results from their ICP measurements.
Instrument Calibration and Maintenance
Proper calibration and maintenance of ICP instruments are critical for obtaining valid analytical data. Calibration ensures that the instrument is producing accurate measurements by matching its response to known standards. This process should be conducted regularly, particularly when changes in sample types or matrix are introduced.
The maintenance of ICP systems involves routine checks and potential repairs to critical components, such as the nebulizer, torch, and spectrometer. Without regular maintenance, performance can degrade over time, leading to increased background noise and reduced detection limits. Specific maintenance procedures often include cleaning the sample introduction systems, replacing wear components, and verifying the stability of the plasma.
It is also important to keep an up-to-date log of maintenance activities and calibration history. This practice not only supports quality assurance but also aids in troubleshooting any issues that may arise during analysis. Establishing a rigorous calibration and maintenance schedule can thus significantly enhance the reliability and consistency of ICP results.
"Regular calibration and maintenance are keys to sustaining the performance of ICP instruments, ensuring that analytical results remain valid over time."
As the analytical landscape continues to evolve, it is essential for professionals in the field to stay informed about these challenges and implement strategies to overcome them. By doing so, they can promote effective and accurate metal analysis using ICP technology.
Case Studies in ICP Applications
The exploration of case studies in ICP applications paves the way towards a better understanding of how Inductively Coupled Plasma technology functions in real-world scenarios. This section highlights the significance of these practical examples, illustrating the effectiveness of ICP instruments in various settings. Each case study provides valuable insights into the benefits and considerations surrounding the application of ICP in metal analysis. By examining specific instances, we can appreciate the capabilities of ICP systems and their impact on industries and research fields alike.
Industrial Metal Assessment
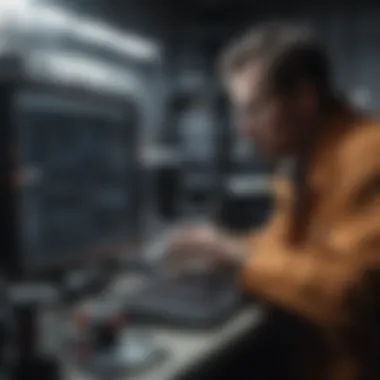
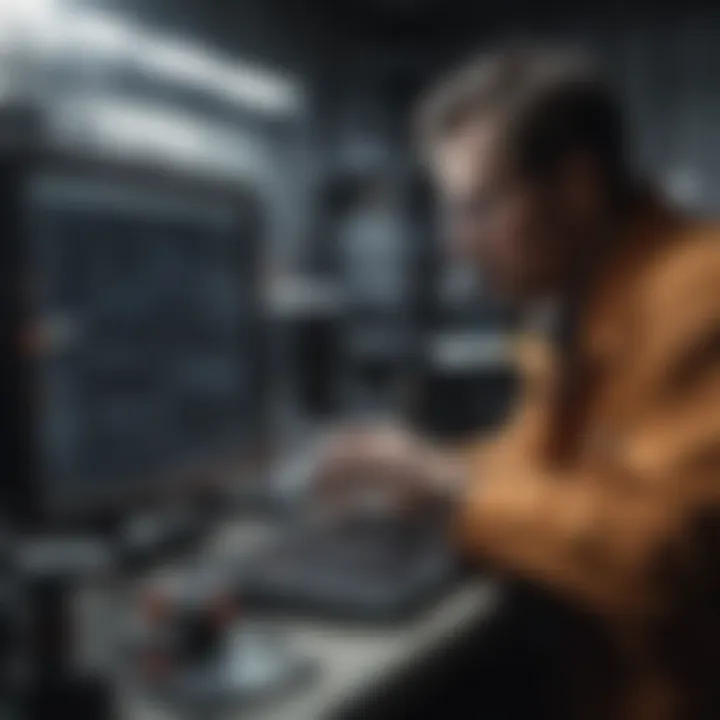
Industrial processes often require stringent testing and analysis of metal samples to ensure quality and compliance with standards. Case studies in industrial metal assessment reveal how ICP technology can be leveraged for effective quality control. In one notable instance, an automotive manufacturer employed ICP analysis to monitor the concentration of trace metals in alloy components used in vehicle production. Precise measurements of elements such as lead and cadmium were critical to meet the governing regulations in manufacturing. The results demonstrated not only the efficacy of the ICP method, but also its ability to deliver rapid and reliable data that guided quality assurance practices.
The benefits of using ICP in this context include:
- High Sensitivity: The capability to detect trace levels of contaminants ensures product safety and compliance.
- Speed: The rapid analysis time allows for timely decision-making in production processes.
- Multi-Element Detection: The ability to analyze multiple elements simultaneously enhances efficiency and reduces costs.
Geochemical Studies
Geochemical studies often delve into the elemental composition of natural resources, and ICP technology serves a pivotal role in this area. For example, a recent study focused on analyzing ore samples to assess mineral deposits for potential extraction. The researchers utilized ICP to determine the concentrations of various metals, including gold, silver, and copper, within the ore. The ICP analysis provided detailed information about the distribution of these elements, which informed decisions on the viability of mining operations.
Key factors in the application of ICP in geochemical studies include:
- Precision: The accuracy of metal concentration measurements aids in developing reliable geological models.
- Environmentally Sound: ICP helps in assessing the impact of mining on the surrounding environment by monitoring metal levels in soil and water samples.
- Research Implications: The data obtained can drive further studies on mineral resource management and sustainability practices.
"Case studies are vital for validating the applicability and effectiveness of ICP technology in diverse analytical scenarios."
Comparative Analysis of Analytical Techniques
In the landscape of analytical chemistry, the comparative analysis of various techniques plays a crucial role in assessing effectiveness, precision, and applicability. This section examines the merits and limitations of various analytical methods used for metal analysis, specifically focusing on Inductively Coupled Plasma (ICP) instruments compared to other techniques such as Atomic Absorption Spectroscopy (AAS), Optical Emission Spectroscopy (OES), and X-ray fluorescence (XRF). Understanding these comparisons is essential for researchers and industry practitioners in selecting the most suitable method for their specific needs.
ICP vs. AAS
Atomic Absorption Spectroscopy (AAS) remains a commonly used technique for trace metal analysis due to its straightforward operation and affordabilty. AAS measures the absorption of light by atoms in the vapor phase, which allows quantification of metals in samples. While AAS is useful, it often encounters challenges that limit its use. For instance, AAS requires extensive sample preparation and can only analyze one element at a time, making it time-consuming for multi-element analysis.
In contrast, ICP can handle multiple elements simultaneously. It operates by ionizing the sample in a plasma, allowing detection of numerous metals in a single run. This efficiency results in reduced analysis time and greater throughput. Additionally, the sensitivity of ICP is generally higher, meaning it can detect lower concentrations of metals than AAS.
ICP vs. OES
Optical Emission Spectroscopy (OES) uses the emission of light from excited atoms to analyze metals, primarily focusing on the spectral lines produced. This technique is similar to ICP in its ability to analyze multiple elements at once. However, OES can be less sensitive in some cases, particularly when measuring trace elements.
The coupling of ICP with OES has proven effective, as ICP provides a stable plasma that enhances the excitation of elements. This synergy improves detection limits and broadens the analytical range. Therefore, while OES has strengths, ICP's superior sensitivity makes it preferred for applications requiring precise measurements of trace metals in diverse matrices.
ICP vs. XRF
X-ray fluorescence (XRF) is another powerful tool for metal analysis that allows for rapid in situ analysis. Unlike ICP, which requires sample dissolution and ionization, XRF operates by bombarding the sample with X-rays, causing the emission of characteristic secondary X-rays. This method is non-destructive and ideal for bulk or solid samples.
Despite its advantages, XRF typically has lower sensitivity than ICP, especially for light elements. Additionally, the analysis might be influenced by sample matrix effects, making interpretation challenging. ICP, on the other hand, allows for more straightforward quantification thanks to its high sensitivity and ability to analyze a broader range of elements.
Recent Innovations in ICP Technology
The landscape of Inductively Coupled Plasma (ICP) technology is constantly evolving. Innovations continue to enhance its efficiency and scope, proving its relevance in the field of metal analysis. The topic of recent advancements is crucial in understanding not only the technological trajectory of ICP but also the resulting implications for various applications. With an increasing need for precision and accuracy in analytical chemistry, these advancements define the future of metal analysis.
Advancements in Detection Methods
Detection methods in ICP technology have seen considerable improvements. Innovations such as Matrix-Assisted Laser Desorption/Ionization (MALDI) and Laser Ablation ICP-MS offer superior sensitivity and lower detection limits. These methods facilitate the analysis of complex matrices and challenging sample types.
- Improved Sensitivity: Recent developments in detector technology allow ICP instruments to achieve lower detection limits. This is vital for trace analysis, especially in environmental and biological samples.
- Real-Time Analysis: The integration of advanced algorithms has made real-time processing possible. This allows researchers to make immediate decisions based on analytical results, significantly speeding up workflows.
"The enhancements in detection methods enable ICP instruments not only to perform traditional analyses but also to adapt to emerging requirements in diverse fields."
Integration of Automation
Automation plays a pivotal role in the evolution of ICP technology. Automated systems streamline processes, enhance reproducibility, and reduce human error. This is especially beneficial in laboratories where large volumes of samples are analyzed daily.
- Sample Handling: Modern ICP systems now incorporate automatic sample introduction mechanisms, which minimize manual intervention and improve throughput.
- Data Analysis: Automation extends into data processing. Software solutions now offer real-time analysis and interpretation of results, allowing scientists to focus on critical assessments rather than routine computations.
- User Experience: Innovative interfaces simplify usage, enabling even non-experts to operate sophisticated equipment efficiently. This user-centered design fosters broader acceptance and integration of ICP technology in various laboratory settings.
In summary, recent innovations in ICP technology, particularly in detection methods and automation, are reshaping metal analysis. These advancements ensure that ICP remains a pivotal technique, adept at meeting contemporary analytical demands.
Future Directions in Metal Analysis Using ICP
The field of metal analysis is undergoing significant advancement, particularly with the integration of Inductively Coupled Plasma (ICP) technology. This section aims to outline the future directions of metal analysis using ICP, along with pertinent considerations and benefits that arise from these developments. As industries and research continue to evolve, the demand for accurate, timely, and comprehensive data intensifies. Hence, examination of prospective developments in ICP technology is crucial.
Emerging Trends
Emerging trends in ICP technology indicate a clear trajectory towards enhanced performance and versatility. One notable trend is the integration of more sensitive detection techniques. In recent years, methods such as Laser Ablation ICP Mass Spectrometry (LA-ICP-MS) have gained prominence. This approach allows for real-time analysis of solid materials with minimal sample preparation, expanding the scope of analysis significantly.
Moreover, developments in software and data analytics facilitate the management of complex datasets generated by ICP instruments. The incorporation of artificial intelligence and machine learning to interpret results more efficiently is becoming increasingly common. These technologies not only optimize analytical workflows but also enhance accuracy and reliability in detecting lower concentrations of metals, thus paving the way for more detailed studies in diverse fields including environmental science and clinical diagnostics.
Potential for Broader Applications
The potential for broader applications of ICP technology is vast. Traditionally focused on industrial metal analysis, ICP is now branching into new areas. For instance, in the realm of nanotechnology, ICP can analyze nanoparticle composition and concentration, which is pivotal in developing novel materials and medical applications.
Furthermore, sectors such as pharmaceuticals are beginning to leverage ICP for quality control purposes. Ensuring that metal contaminants are within safe limits is crucial in drug development and production.
Another promising application lies in geosciences where ICP's capability to analyze trace metals aids in understanding mineral compositions and their environmental impacts. The rich data offered by ICP instruments enables researchers to draw valuable conclusions from geological samples, influencing conservation efforts and resource management strategies.
In summary, the future directions of metal analysis using ICP technology are characterized by emerging trends that enhance sensitivity and versatility, alongside its capacity for broader applications across various sectors. This integration fosters not only scientific understanding but also practical solutions to contemporary challenges.